Whole-organ assessment of a joint with osteoarthritis (OA) requires tailored MR imaging hardware and imaging protocols to diagnose and monitor degenerative disease of the cartilage, menisci, bone marrow, ligaments, and tendons. Image quality benefits from increased field strength, and 3.0-T MR imaging is used increasingly for assessing joints with OA. Dedicated surface coils are required for best visualization of joints affected by OA, and the use of multichannel phased-array coils with parallel imaging improves image quality and/or shortens acquisition times. Sequences that best show morphologic abnormalities of the whole joint include intermediate-weighted fast-spin echo sequences. Also quantitative sequences have been developed to assess cartilage volume and thickness and to analyze cartilage biochemical composition.
MR imaging must be tailored for imaging of osteoarthritis (OA) by using scanners with adequate field strength, coils that allow imaging with high spatial resolution, and optimized imaging sequences that best visualize tissues involved by OA. These tissues encompass cartilage, menisci, ligaments, and bone marrow. Among these tissues, cartilage has an outstanding role, and imaging cartilage is particularly challenging in terms of the required signal-to-noise-ratio (SNR), spatial resolution, and contrast. The requirements for cartilage imaging dictate the overall requirements in hardware and in sequence profiles.
This article (1) outlines requirements concerning field strength, analyzing scanners with different field strengths from 0.2 T to 7 T, (2) investigates the potential of open and extremity MR imaging, (3) examines the role of weight-bearing MR imaging, (4) reviews coil technology, and (5) analyzes sequence protocols and their role in imaging the different tissues involved in OA.
Field strength
Considerations concerning field strength required for OA imaging always should take into account that cartilage imaging is critical for adequate whole-organ OA analysis. Previous studies have shown that imaging with low field strength clearly has limitations in assessing cartilage morphology and therefore is not recommended for OA imaging. Woertler and colleagues compared the diagnostic performance of a dedicated orthopedic MR imaging system (0.18 T) and a conventional MR imaging system (1.0 T) in the detection of articular cartilage lesions created in an animal model. Using receiver operating characteristics (ROC) analysis with three different radiologists, these investigators found that the high-field-strength system demonstrated a significantly better diagnostic performance than the low-field-stength system in the detection of less-than-full-thickness articular cartilage lesions ( P < .001). Ahn and colleagues studied cadaver patellae using a 0.2-T extremity-only magnet and found that high-grade cartilaginous lesions could be evaluated reliably with low-field-strength MR imaging by using a combination of imaging sequences. Limitations were encountered analyzing less-than-full-thickness cartilage lesions, however. Based on the results of these previous studies, the use of MR imaging scanners with a field strength of at least 1.0 T is recommended for imaging cartilage.
The current standard is 1.5-T imaging, and most of the studies establishing MR imaging for assessment of OA were conducted at this field strength. Semiquantitative scores to grade OA and techniques to quantify cartilage volume were developed at 1.5 T. The early studies analyzing quantitative parameters to characterize the biochemical composition of cartilage, such as T2 relaxation time and T1rho mapping, as well as delayed gadolinium-enhanced MR imaging of cartilage (dGEMRIC), also were performed at 1.5 T.
Although 1.5-T imaging is standard, a number of studies have demonstrated that 3.0-T MR imaging allows better visualization of cartilage lesions and therefore may be better suited for the overall assessment of OA. Using optimized high-resolution MR imaging sequences in an animal model, Link and colleagues showed that cartilage lesions were visualized better and diagnostic performance was improved at 3.0 T compared with 1.5 T. Interestingly, however, standard lower-spatial-resolution intermediate (IM)-weighted fast spin echo (FSE) sequences did not improve diagnostic performance at 3.0 T. Fig. 1 shows two corresponding IM-weighted fat-saturated MR images obtained at 1.5 and 3.0 T demonstrating a superficial cartilage defect at the patella in a pig knee, which is visualized better at 3.0 T. Although this study was performed at the knee, additional studies performed at human cadaver ankles also showed better diagnostic performance in assessing cartilage lesions and a higher sensitivity for assessing ligamentous and tendon pathology at 3.0 T than at 1.5 T.
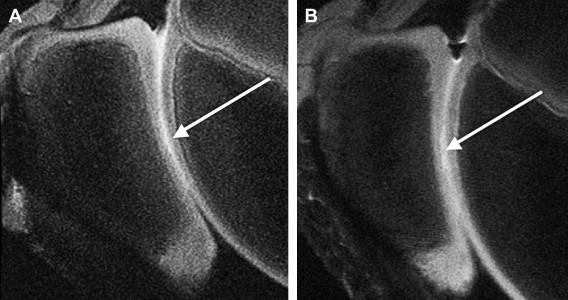
Recently Kijowski and colleagues performed a retrospective study comparing the diagnostic performance of 1.5-T and 3.0-T MR imaging protocols for evaluating the articular cartilage of the knee joint in symptomatic patients. Analyzing 241 knee MR images at 1.5 T and 226 MR images at 3.0 T, these investigators found that the sensitivity, specificity, and accuracy of MR imaging for detecting cartilage lesions were 69.3%, 78.0%, and 74.5%, respectively, at 1.5 T and were 70.5%, 85.9%, and 80.1%, respectively, at 3.0 T. The MR imaging protocol had significantly higher specificity and accuracy ( P < .05) but not higher sensitivity ( P = .73) for detecting cartilage lesions at 3.0 T than at 1.5 T. The investigators concluded that 3.0-T MR imaging protocols were superior to the 1.5-T protocol for evaluating the articular cartilage of the knee joint in symptomatic patients ( Figs. 2 and 3 ).
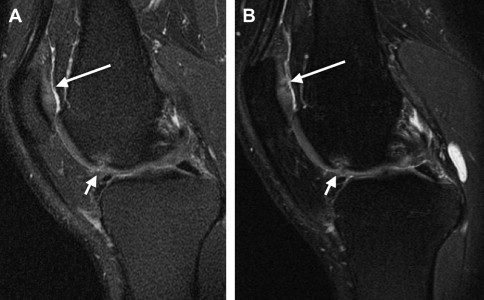
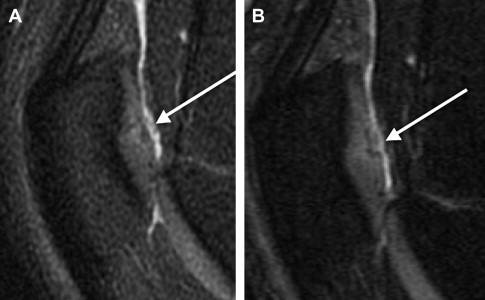
Bauer and colleagues compared the precision and accuracy of 3.0-T and 1.5-T MR imaging in the quantification of cartilage volume by using direct volumetric measurements as a reference standard in a cadaver model. These investigators calculated accuracy errors for MR imaging–based volume calculations of 3.0% at the femur for standard fat-suppressed spoiled gradient-echo (SPGR) sequences at 3.0 T, versus 16% for the standard fat-suppressed SPGR sequence at 1.5 T. Effective signal-to-noise ratio and effective contrast-to-noise ratio also were substantially improved at 3.0 T. This study provides evidence that cartilage volumetric measurements obtained at 3.0 T are more accurate than those obtained at 1.5 T. Eckstein and colleagues performed an in vivo study in patients who had OA and normal volunteers to evaluate the precision of quantitative MR imaging assessments of human cartilage morphology at 3.0 T and to correlate the measurements at 3.0 T with validated measurements at 1.5 T. They found that with a slice thickness of 1.5 mm, measurements tended to be more reproducible at 3.0 T than at 1.5 T, and they concluded that imaging at 3.0 T may provide superior ability to detect changes in cartilage status over time and to determine responses to treatment with structure-modifying drugs.
To achieve the best possible imaging technique for assessing OA, the National Institute of Health–sponsored Osteoarthritis Initiative (OAI) therefore adopted imaging at 3.0 T. The OAI is a nationwide, multicenter research study that provides a large dataset of clinical information, questionnaires, radiographs, and MR imaging studies obtained from nearly 5000 participants (4796 participants at baseline) who are followed up every 12 months for a period of 48 months. The overall aim of the OAI is to develop a public domain research resource to facilitate the scientific evaluation of biomarkers for OA as potential surrogate end points for disease onset and progression. The OAI recruits participants who have knee OA (the progression cohort) and participants who have risk factors but no symptoms of OA (the incidence cohort). The imaging protocol includes morphologic and quantitative MR imaging sequences performed at 3.0 T with five identical scanners from the same manufacturer.
To date, MR imaging at 7.0 T is a research application, and only limited studies have been performed in human participants. Currently available sequence protocols have not been shown to be superior to 3.0 T in the assessment of OA. Future research work clearly will need to focus on developing adequate surface coils and optimized sequences for imaging at 7.0 T.
Extremity and open MR imaging
Peripheral extremity magnets have lower installation, maintenance, and management costs than whole-body systems, and these systems are beneficial for patients who have claustrophobia. Moreover, they do not require the amount of shielding necessary for a whole-body system and potentially can be used in private offices, thus making MR imaging widely available. Dedicated extremity scanners operating at higher field strength have been developed to overcome the limitations of 0.2-T MR imaging scanners in visualizing cartilage and other anatomic structures such as ligaments ( Fig. 4 ). Using a dedicated peripheral extremity-only MR imaging system operating at 1.0 T, Roemer and colleagues examined 34 knees using fat-suppressed FSE proton density (PD)-weighted sequences. They found good to excellent interobserver performance in assessing OA-associated abnormalities, including cartilage lesions ( Fig. 5 ). These high-field peripheral scanners may offer a low-cost alternative providing adequate image quality for assessing cartilage pathology. Currently, peripheral extremity scanners operating at 1.5-T field strength are available also.
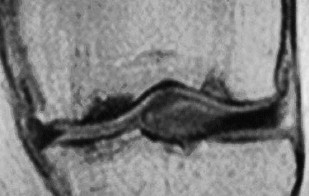
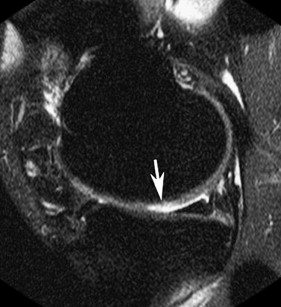
Depending on the open MR imaging configuration, patients can be placed in the scanner in either a supine or weight-bearing position. Open MR imaging scanners allow the functional aspects of joint function to be assessed and may therefore be useful in investigating conditions associated with abnormal articulation in certain joint positions that may lead to accelerated OA. For example, femoroacetabular impingement is a condition in which labral and cartilage damage results from an abnormal morphology of the head–neck junction (cam-type impingement) or an abnormally deep acetabulum (pincer-type impingement). This impingement typically occurs with flexion, abduction, and external rotation. Open MR imaging can be used to assess these functional aspects of the hip joint. Yamamura and colleagues demonstrated that, although impingement occurred frequently during daily activities, it was not associated with accelerated OA of the hip in male and female Japanese participants.
Open MR imaging scanners also can be used to assess patella kinematics and patellofemoral contact areas, which may play a role in development of femoropatellar OA. Hinterwimmer and colleagues studied a sample of 15 patients who had genu varum and mild OA and 15 healthy volunteers in an open MR imaging scanner. Three-dimensional (3D) gradient echo sequences of the knee were obtained in 0°, 30°, and 90° with and without activity of the extensor muscles. Contact areas between patella and femur cartilage were defined by intersection of opposing cartilage volumes. These investigators, however, were not able to demonstrate significant differences in patella kinematics and patellofemoral contact areas ( P > .05) between varus knees with mild OA and healthy knees either at the different flexion angles or under extending muscle activity.
Extremity and open MR imaging
Peripheral extremity magnets have lower installation, maintenance, and management costs than whole-body systems, and these systems are beneficial for patients who have claustrophobia. Moreover, they do not require the amount of shielding necessary for a whole-body system and potentially can be used in private offices, thus making MR imaging widely available. Dedicated extremity scanners operating at higher field strength have been developed to overcome the limitations of 0.2-T MR imaging scanners in visualizing cartilage and other anatomic structures such as ligaments ( Fig. 4 ). Using a dedicated peripheral extremity-only MR imaging system operating at 1.0 T, Roemer and colleagues examined 34 knees using fat-suppressed FSE proton density (PD)-weighted sequences. They found good to excellent interobserver performance in assessing OA-associated abnormalities, including cartilage lesions ( Fig. 5 ). These high-field peripheral scanners may offer a low-cost alternative providing adequate image quality for assessing cartilage pathology. Currently, peripheral extremity scanners operating at 1.5-T field strength are available also.
Depending on the open MR imaging configuration, patients can be placed in the scanner in either a supine or weight-bearing position. Open MR imaging scanners allow the functional aspects of joint function to be assessed and may therefore be useful in investigating conditions associated with abnormal articulation in certain joint positions that may lead to accelerated OA. For example, femoroacetabular impingement is a condition in which labral and cartilage damage results from an abnormal morphology of the head–neck junction (cam-type impingement) or an abnormally deep acetabulum (pincer-type impingement). This impingement typically occurs with flexion, abduction, and external rotation. Open MR imaging can be used to assess these functional aspects of the hip joint. Yamamura and colleagues demonstrated that, although impingement occurred frequently during daily activities, it was not associated with accelerated OA of the hip in male and female Japanese participants.
Open MR imaging scanners also can be used to assess patella kinematics and patellofemoral contact areas, which may play a role in development of femoropatellar OA. Hinterwimmer and colleagues studied a sample of 15 patients who had genu varum and mild OA and 15 healthy volunteers in an open MR imaging scanner. Three-dimensional (3D) gradient echo sequences of the knee were obtained in 0°, 30°, and 90° with and without activity of the extensor muscles. Contact areas between patella and femur cartilage were defined by intersection of opposing cartilage volumes. These investigators, however, were not able to demonstrate significant differences in patella kinematics and patellofemoral contact areas ( P > .05) between varus knees with mild OA and healthy knees either at the different flexion angles or under extending muscle activity.
Weight-bearing MR imaging
Weight-bearing MR imaging can be performed using open MR imaging systems that have vertically orientated magnets or with whole-body MR imaging systems that use special loading devices for the knee, such as the one described by Nishii and colleagues. Although the vertical alignment of the magnets in a double-doughnut system allows true weight-bearing MR imaging studies, the field strength and image quality of these scanners are limited, affecting cartilage imaging in particular. Image quality in whole-body systems generally is superior, and loading devices also have been applied successfully in 3.0-T scanners. Static loading conditions usually are obtained by applying an axial compression force of approximately 50% of body weight during imaging.
Anterior cruciate ligament (ACL) tears have been identified as an important factor in the pathogenesis of OA, and it also has been found that patients who have ACL repair experience accelerated OA. Logan and colleagues used a vertical open MR imaging system to study the tibiofemoral kinematics of ACL-deficient weight-bearing in 10 patients. The tibiofemoral motion was assessed through the arc of flexion from 0° to 90° in the ACL-deficient and normal contralateral knees. These investigators found that ACL tears change tibiofemoral kinematics, producing anterior subluxation of the lateral tibial plateau. They hypothesized that altered kinematics may explain, at least in part, the increased incidence of secondary OA in patients who have had an ACL tear. In another study, the same investigators used the same weight-bearing technique in an open MR imaging system to study 10 patients who had isolated reconstruction of the ACL (hamstring autograft) in one knee and a normal contralateral knee. They found that ACL reconstruction reduces sagittal laxity to within normal limits but does not restore normal tibiofemoral kinematics; the abnormal kinematics, again, may explain the relatively high rate of accelerated OA in this patient population.
Currently there has been substantial interest in studying changes in cartilage volume and biochemical matrix in response to load-bearing. It has been suggested that failure to respond to normal load-bearing may be caused by the disorder or degeneration of articular cartilage with collagen disorganization or abnormal water content. Nishii and colleagues used T2 relaxation time measurements to study the biochemical composition of the normal hyaline knee cartilage under loading. Using 3.0-T MR imaging and applying an axial compression force of 50% of body weight during imaging, they obtained sagittal T2 maps of the medial and lateral femorotibial joints of 22 healthy volunteers. They compared the T2 values of the femoral and tibial cartilage at the weight-bearing area in the unloading and loading conditions. These investigators found that under loading conditions, mean cartilage T2 values generally decreased. At the medial joint compartment, a significant decrease in T2 values with loading was observed at the femoral region in direct contact with the opposing tibial cartilage. A significant decrease in T2 values with loading also was observed at the medial and lateral tibia, at regions both covered and not covered by the meniscus.
In addition, the role of the meniscus during weight-bearing is critical in preventing OA. While axial compression forces are applied, MR imaging directly visualizes changes of the meniscus in morphology, deformity, extrusion, and, potentially, biochemistry ( Fig. 6 ). These findings may help elucidate the evolution and pathophysiology of OA, but at present the experience in direct visualization of meniscal abnormalities with weight-bearing MR imaging is limited.
Surface coils
In addition to adequate field strength, dedicated surface coils are important prerequisites to achieve good image quality and visualization of the joint tissues involved in OA. Currently, surface coils for the wrist, shoulder, knee, and ankle are standard; most of these coils are multichannel phased-array coils. For visualization of smaller structures such as the fingers and toes, smaller (so-called “microscopy”) coils have been developed. These coils allow imaging with small fields of view and high spatial resolution.
Fig. 7 shows the effect of the coil on the image quality. In panel A , a non-dedicated two-element paddle coil was used; in panel B , a dedicated three-element shoulder coil was applied. The effect on visualization of the cartilage is evident. Even if a high-quality, high-field scanner is used, inadequate coils limit image quality substantially, as shown by Lutterbey and colleagues. These investigators found that using the standard body coil at 3.0 T for imaging of the knee gave a lower image performance than achieved using a 1.5-T scanner with a dedicated knee coil.
Multichannel phased-array coils give high SNR and allow parallel imaging, which can provide better image quality with the same acquisition time or can shorten acquisition time by maintaining image quality. With parallel imaging, each of the coil elements/channels provides image information separately; the information then is fused to obtain one image ( Fig. 8 ).
In an in vitro study performed in human cadaver ankles, Bauer and colleagues compared an autocalibrating parallel imaging technique at 3.0 T with standard acquisitions at 3.0 T for small field-of-view imaging of the ankle. Using parallel imaging techniques, these investigators obtained a reduction in scan time of approximately 44%. All images were analyzed for image quality by two radiologists. Macroscopic findings after dissection served as a reference for the pathologic evaluation. This study did not find a significant difference in ligament and cartilage visualization or in image quality between standard and generalized, autocalibrating, partially parallel acquisitions reconstructions at 3.0 T. The authors concluded that parallel imaging can provide more flexibility in protocol design by either shortening image acquisition time or improving image quality with the same acquisition time.
Zuo and colleagues evaluated the feasibility and reproducibility of quantitative cartilage imaging with parallel imaging at 3.0 T and determined the impact of the acceleration factor on morphologic and relaxation measurements. They found that morphologic parameters and relaxation time maps from parallel imaging showed results comparable with those obtained by the conventional technique. Intraclass correlation coefficients of the two methods for measuring cartilage volume and mean cartilage thickness were very high both for T1rho, and T2 measurements, and the reproducibility was excellent. In summary, for both quantitative and morphologic OA imaging, multichannel phased-array coils with parallel imaging techniques are recommended.
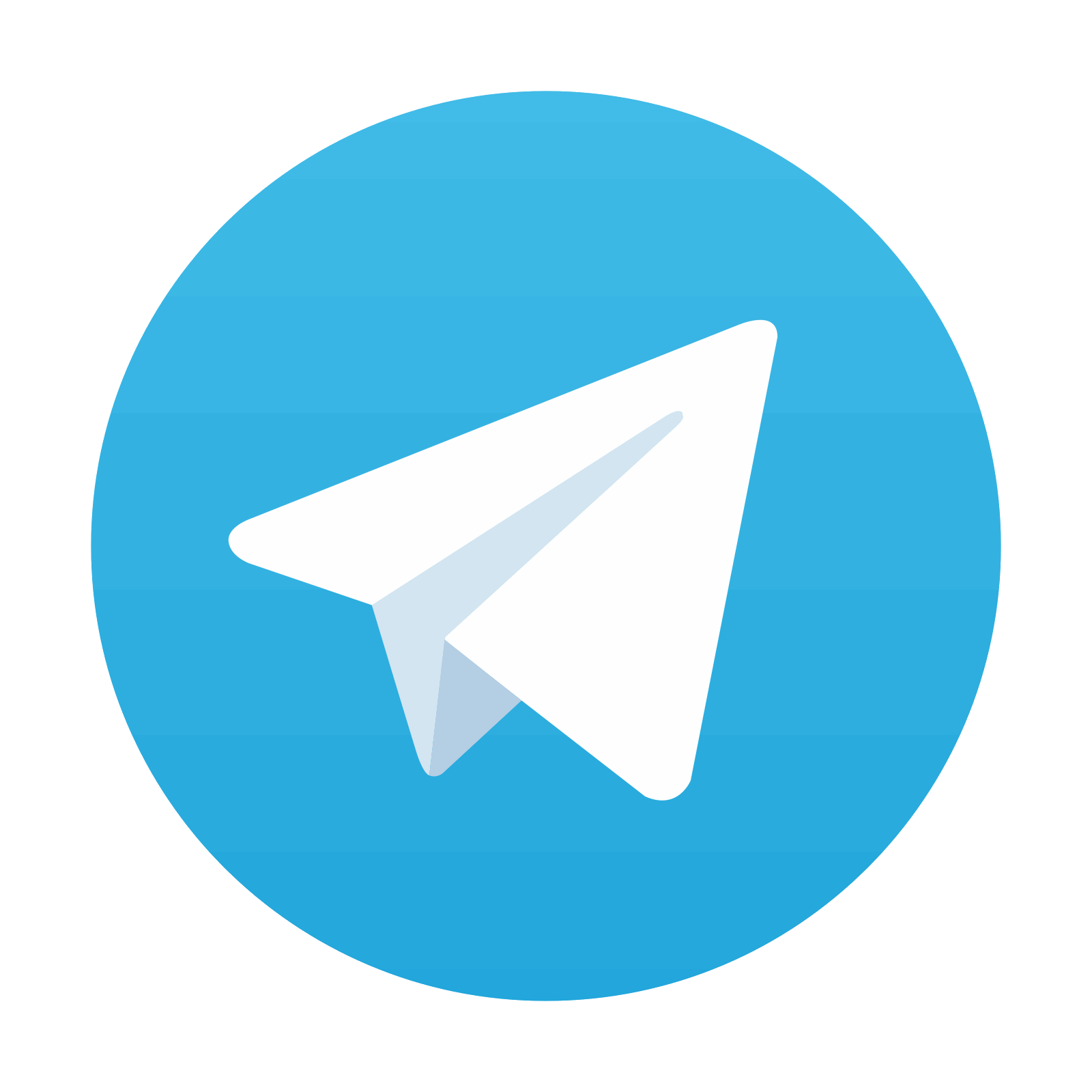
Stay updated, free articles. Join our Telegram channel

Full access? Get Clinical Tree
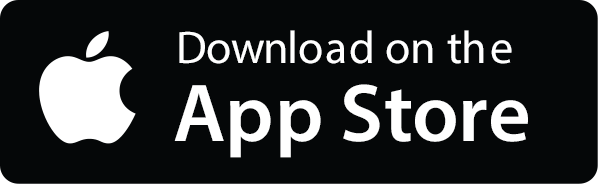
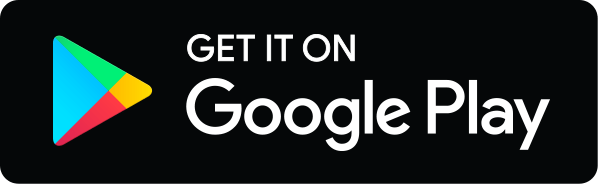