MR imaging of the brachial plexus assesses the continuity of the elements, relationship, and orientation of lesions, evaluates morphology, and reveals secondary features of plexopathies. The selection of sequences and imaging planes is guided by the history, clinical examination, and suspected type and location of the abnormality. Increased magnet strength and isotropic imaging with multiplanar reconstructions may allow for standardized imaging protocols and shorter examination time. This article discusses MR imaging evaluation of the brachial plexus, relevant anatomy, and common pathology with clinical and imaging details, indications for use of intravenous contrast, differential considerations, and diagnostic pitfalls.
- •
Sagittal imaging should be used to assess the relationship of the plexus lesion to the plexus components and subclavian and axillary arteries.
- •
The coronal images with a large field of view allow for comparison of signal intensity, size, and enhancement of the affected side with the contralateral control plexus.
- •
The appearance and orientation of the lesion may provide valuable diagnostic hints.
- •
Routine use of intravenous contrast is not required.
- •
MR imaging after brachial plexus injury should be delayed at least 3 weeks, unless the patient develops progressive neurologic deterioration or Brown-Séquard syndrome symptoms.
- •
However, this is now controversial—some surgeons are recommending ultraearly plexus exploration within the first or second week when multiple root avulsions are suspected, so that nerve transfers can be done as early as possible, and to avoid operating through dense scar.
- •
Even in the presence of a nerve root avulsion, the residual root within the pseudomeningocele should be identified because it may allow nerve grafting.
- •
Involvement of C7 spinal nerve may prevent patients with C5 and C6 avulsions from undergoing an Oberlin nerve transfer procedure and should be mentioned in the report.
- •
Vascular evaluation should be performed in trauma cases if there is a distal pulse deficit, local hematoma, bruit, or anatomically related nerve symptoms, because of possible delay in development of pseudoaneurysm.
- •
In cases of direct neoplastic invasion of the brachial plexus, obliteration of the interscalene fat determines surgical candidacy.
- •
Unlike extrapleural lesions, the interface of the Pancoast tumor and the lung is irregular.
Introduction
The interpretation of the images of the brachial plexus may be intimidating for a general radiologist. However, knowledge of anatomy, common pathology, direct and indirect imaging signs of diseases affecting the brachial plexus, the patient’s history and clinical presentation, and a systematic approach to the images will allow a radiologist to construct an anatomically correct limited differential diagnosis. Judicious use of contrast could help in classification of vascular lesions, delineation of infection, and in cases of radiation-treated tumors.
Anatomy
The brachial plexus is a neural network responsible for both sensory and motor innervation of the ipsilateral chest, shoulder, arm, and hand. It is most commonly formed by the ventral rami of C5 through T1 spinal nerves as they exit the neural foramina ( Fig. 1 ). There are multiple variations in anatomy of the brachial plexus. However, from the perspective of a radiologist, the detectable variations are at the level of the roots. The most common variant at this level, the prefixed brachial plexus, includes only the C4 through C7 spinal nerves. The second-most common variant at this level, the postfixed plexus, receives contributions from the C6 through T2 levels. There are also rare cases of a combination of the two, with contributions from C4 through T2. These variants are important for predisposition to certain pathologic conditions, and need to be known for surgical planning.
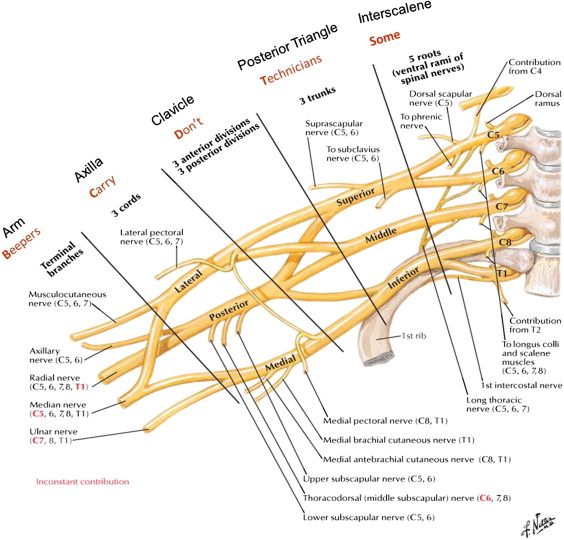
From the imaging perspective, the brachial plexus can be divided into the supraclavicular, retroclavicular, and infraclavicular portions. The supraclavicular plexus contains the spinal nerves (incorrectly called roots in some anatomic textbooks) and the trunks. After they exit the neural foramina, behind the vertebral arteries, the spinal nerves are located in the interscalene space ( Fig. 2 ), between the anterior scalene muscle anteriorly, middle and posterior scalene muscles posteriorly, and first rib and subclavian artery inferiorly. At the lateral border of the middle scalene muscle, the C5 and C6 spinal nerves form the superior trunk, C7 forms the middle trunk, and C8 and T1 form the inferior trunk. The trunks continue posterior and superior to the subclavian artery, and form anterior and posterior divisions within the posterior triangle and behind the clavicle. The costoclavicular triangle, a common location (see Fig. 2 ) of functional compression of the brachial plexus, is formed by the clavicle superiorly, the subclavius muscle anteriorly, and the first rib and middle scalene muscle posteriorly. The subclavian vein and artery are located within the inferior aspect of this space, with the former located anterior to the latter. This space contains the transition from the divisions, located in the retroclavicular space, to the cords. The infraclavicular portion of the plexus extends into the retropectoralis minor space. The anterior wall of this space is the pectoralis minor muscle, posterior and superior walls are the subscapularis muscle, and posterior and inferior walls are created by the anterior chest wall. In this space the cords are visualized posterior superior to the axillary artery (see Fig. 2 ). The medial cord, a continuation of the anterior division of the inferior trunk, in its distal portion is seen at the inferior wall of the artery. The lateral cord, receiving anterior division nerve fibers from the superior and middle trunks, is located adjacent to the anterior superior wall of the artery. The posterior cord, receiving posterior divisions’ contributions from all trunks, is located superior posteriorly to the artery. The infraclavicular portion of the brachial plexus also includes five terminal branches: median, ulnar, musculocutaneous, axillary, and radial nerves (usually visualized in the lower axilla and proximal arm; see Fig. 1 ). Some of the branches originate at other zones of the brachial plexus. The latissimus dorsi, teres major, and subscapularis muscles receive their innervation from the posterior cord. The supraspinatus and infraspinatus muscles receive innervation from the upper trunk via the suprascapular nerve. The biceps and coracobrachialis muscles receive innervation from the lateral cord via the musculocutaneous nerve. The medial cord innervates cutaneous structures and the ulnar nerve. In terms of the spinal nerve innervation, C5 is responsible for supraspinatus, infraspinatus, and the deltoid; C6 for biceps; C7 for triceps and forearm extensors; and C8 and T1 for finger flexors and intrinsic muscles of the hand. On deep reflex testing, the biceps reflex is predominantly supplied by C5 spinal nerve, the brachioradialis reflex by C6, the triceps by C7, and the pectoralis by the entire plexus. Although knowing the branches, their origin, and the 59 muscles innervated by the plexus is very important for a neurosurgeon for localization of the lesions based on the symptoms caused by the affected branches, current clinical MR imaging does not allow visualization of these structures in most cases. However, direct visualization of the components of the brachial plexus on MR imaging allows for confirmation and delineation of pathologic abnormalities. It is especially valuable because the nerve conduction studies only indirectly examine the brachial plexus and the symptom-based clinical localization may be misleading in the presence of brachial plexus branching variants.
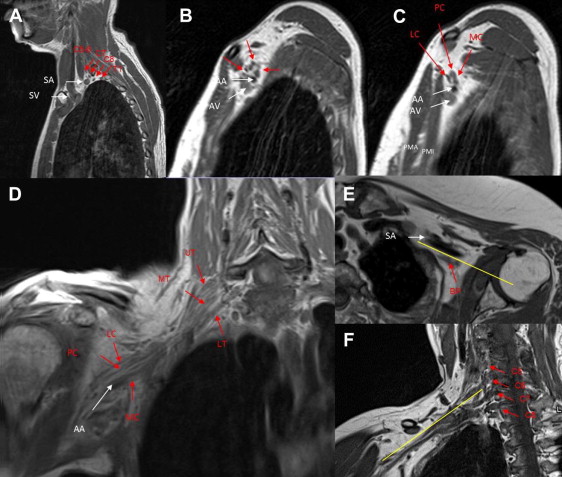
Anatomy
The brachial plexus is a neural network responsible for both sensory and motor innervation of the ipsilateral chest, shoulder, arm, and hand. It is most commonly formed by the ventral rami of C5 through T1 spinal nerves as they exit the neural foramina ( Fig. 1 ). There are multiple variations in anatomy of the brachial plexus. However, from the perspective of a radiologist, the detectable variations are at the level of the roots. The most common variant at this level, the prefixed brachial plexus, includes only the C4 through C7 spinal nerves. The second-most common variant at this level, the postfixed plexus, receives contributions from the C6 through T2 levels. There are also rare cases of a combination of the two, with contributions from C4 through T2. These variants are important for predisposition to certain pathologic conditions, and need to be known for surgical planning.
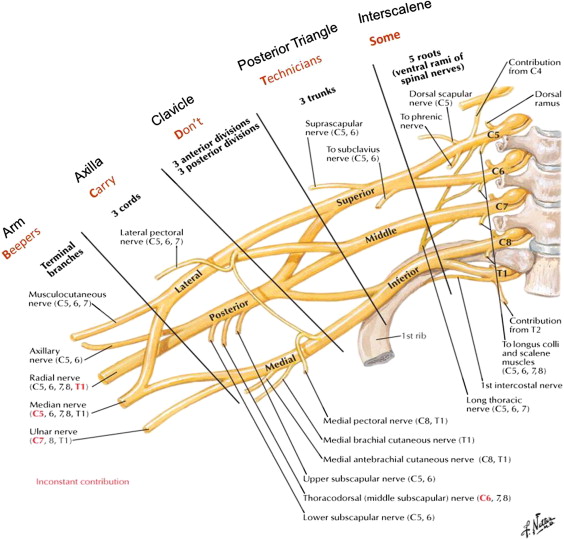
From the imaging perspective, the brachial plexus can be divided into the supraclavicular, retroclavicular, and infraclavicular portions. The supraclavicular plexus contains the spinal nerves (incorrectly called roots in some anatomic textbooks) and the trunks. After they exit the neural foramina, behind the vertebral arteries, the spinal nerves are located in the interscalene space ( Fig. 2 ), between the anterior scalene muscle anteriorly, middle and posterior scalene muscles posteriorly, and first rib and subclavian artery inferiorly. At the lateral border of the middle scalene muscle, the C5 and C6 spinal nerves form the superior trunk, C7 forms the middle trunk, and C8 and T1 form the inferior trunk. The trunks continue posterior and superior to the subclavian artery, and form anterior and posterior divisions within the posterior triangle and behind the clavicle. The costoclavicular triangle, a common location (see Fig. 2 ) of functional compression of the brachial plexus, is formed by the clavicle superiorly, the subclavius muscle anteriorly, and the first rib and middle scalene muscle posteriorly. The subclavian vein and artery are located within the inferior aspect of this space, with the former located anterior to the latter. This space contains the transition from the divisions, located in the retroclavicular space, to the cords. The infraclavicular portion of the plexus extends into the retropectoralis minor space. The anterior wall of this space is the pectoralis minor muscle, posterior and superior walls are the subscapularis muscle, and posterior and inferior walls are created by the anterior chest wall. In this space the cords are visualized posterior superior to the axillary artery (see Fig. 2 ). The medial cord, a continuation of the anterior division of the inferior trunk, in its distal portion is seen at the inferior wall of the artery. The lateral cord, receiving anterior division nerve fibers from the superior and middle trunks, is located adjacent to the anterior superior wall of the artery. The posterior cord, receiving posterior divisions’ contributions from all trunks, is located superior posteriorly to the artery. The infraclavicular portion of the brachial plexus also includes five terminal branches: median, ulnar, musculocutaneous, axillary, and radial nerves (usually visualized in the lower axilla and proximal arm; see Fig. 1 ). Some of the branches originate at other zones of the brachial plexus. The latissimus dorsi, teres major, and subscapularis muscles receive their innervation from the posterior cord. The supraspinatus and infraspinatus muscles receive innervation from the upper trunk via the suprascapular nerve. The biceps and coracobrachialis muscles receive innervation from the lateral cord via the musculocutaneous nerve. The medial cord innervates cutaneous structures and the ulnar nerve. In terms of the spinal nerve innervation, C5 is responsible for supraspinatus, infraspinatus, and the deltoid; C6 for biceps; C7 for triceps and forearm extensors; and C8 and T1 for finger flexors and intrinsic muscles of the hand. On deep reflex testing, the biceps reflex is predominantly supplied by C5 spinal nerve, the brachioradialis reflex by C6, the triceps by C7, and the pectoralis by the entire plexus. Although knowing the branches, their origin, and the 59 muscles innervated by the plexus is very important for a neurosurgeon for localization of the lesions based on the symptoms caused by the affected branches, current clinical MR imaging does not allow visualization of these structures in most cases. However, direct visualization of the components of the brachial plexus on MR imaging allows for confirmation and delineation of pathologic abnormalities. It is especially valuable because the nerve conduction studies only indirectly examine the brachial plexus and the symptom-based clinical localization may be misleading in the presence of brachial plexus branching variants.
Imaging technique
The imaging of the brachial plexus requires understanding of the course of its components and the best planes for their visualization. The identification of the components of the plexus and their relationship to the bony and vascular structures, and assessment of the adjacent fat planes are best done on the sagittal non–fat-suppressed T1-weighted images because this plane demonstrates the cross-section of these structures. However, most neuroradiologists are more comfortable dealing with the neural elements in the axial and coronal planes. The cords, nerve roots, and foraminal portions of the roots are best seen on the high-resolution axial and oblique coronal images. The coronal images with a large field of view allow for comparison of signal intensity (T2-fat-suppressed imaging), size, and enhancement of the affected side with the contralateral control plexus. The unilateral coronal images are most useful for appreciation of edema, enhancement, and enlargement of the roots, trunks, divisions, and cords. The visualization of anatomic detail is variable in this plane. However, the T1-weighted images may be useful for demonstration of bony anatomy.
The use of intravenous contrast for imaging of the brachial plexus is not required on routine basis, since the fat-suppressed T2 images demonstrate the location and the extent of the common pathology, while the T1 weighted images demonstrate the relationship of an abnormality and the adjacent structures. However, the contrast administration is necessary in cases of infection, both to define the extent of the infectious process and assess for presence of adjacent drainable collections. It also could help in defining the extent of the abnormality in secondary neoplastic involvement. Futhermore, in some radiation treated tumor cases, it may differentiate tumor recurrence from radiation changes based on progression of nodular enhancement. In cases of TOS (Thoracic Outlet Syndrome) the intravenous contrast, as a part of provocative position magnetic resonance angioaphic evaluation, commonly supplemented with sagittal T1-weighted imaging in both positions, demonstrates the location and the extent of the compression as well as secondary changes in the vessel lumen. Finally, in cases presenting with vascular lesions the contrast allows for better characterization and classification of a lesion, and selection of the appropriate management.
The appearance and orientation of the lesion may provide valuable hints ( Fig. 3 ). The lesion centered at the interscalene triangle usually involves spinal nerves or trunks. The long axis of an oval, well-defined lesion parallel to the nerve of origin suggests a nerve sheath tumor. If such a lesion is oriented obliquely, from superomedial to inferolateral, it originates from the upper roots. If it is oriented horizontally, it originates from the inferior roots. The vertical lesion in the interscalene triangle most likely represents secondary involvement of the plexus.
Although custom tailoring each examination for the expected pathologic abnormality and lesion localization may provide the best quality of imaging examination, it is impractical in today’s high-volume reading environment. The radiologist needs to separate the examinations by the pathologic categories and establish the general protocols for each category. This will allow judicious use of intravenous contrast and higher efficiency. The radiologist also has to find a comfortable balance between spatial resolution and the area of coverage based on the type of pathologic abnormality referred to the practice and adjust the MR imaging parameters, such as field of view and the matrix size, appropriately. Increased magnet strength and availability of isotropic imaging with multiplanar reconstructions may allow for a decrease in need for custom-tailored examinations and shorten overall time of the examination, especially for cooperative patients. A routine sample protocol is included in Table 1 .
Brachial Plexus MR Imaging Protocol (3T siemens) | |||||||||
---|---|---|---|---|---|---|---|---|---|
Sequence | Slice/skip (mm) | Field of view (mm) | Matrix | NEX | TE/TR | Turbo Factor | Flip Angle | Bandwidth (Hz/Px) | TI |
Axial T1-weighted | 4/1 | 240 | 256 × 205 | 2 | 700/9.1 | 3 | 130 | 271 | — |
Axial STIR (BLADE) | 4/1 | 240 | 256 Ă— 256 | 1 | 4500/80 | 16 | 130 | 362 | 220 |
Sagittal T1-weighted | 4/0.8 | 240 | 320 × 240 | 2 | 700/9.4 | 3 | 150 | 252 | — |
Coronal STIR (BLADE) | 4/1.2 | 240 | 256 Ă— 256 | 1 | 4500/80 | 16 | 130 | 362 | 220 |
Coronal T1-weighted | 4/1.2 | 240 | 320 × 240 | 1 | 625/9.6 | 3 | 150 | 240 | — |
Sagittal T1-weighted FS PRE- and POST | 4/0.8 | 240 | 320 × 240 | 1 | 750/9.4 | 3 | 150 | 252 | — |
Coronal T1-weighted FS POST | 4/1.2 | 240 | 320 × 240 | 3 | 650/96 | 3 | 150 | 240 | — |
Symptoms
Commonly listed brachial plexus–related symptoms are pain, paresthesias, sensory and motor deficits in the appropriate distribution, scapular winging (involvement of the spinal accessory, dorsal scapular, or long thoracic nerve), diaphragmatic paralysis (involvement of the phrenic nerve innervated by C3 and C5), and Horner syndrome (involvement of the postganglionic C8 and T1 nerve roots). However, depending on the type of pathologic abnormality, the predominant component changes (eg, motor deficits in trauma, pain in neoplasm, and sensory deficits in radiation-treated cases and some tumors).
Pathologic conditions
Brachial plexus pathologic conditions can be separated into two categories: traumatic and nontraumatic. If there is no history of trauma, then an MR imaging of the brachial plexus with contrast should be the study of choice, especially if a mass is suspected. In the setting of trauma, either CT myelography, especially for a suspected proximal injury, or brachial plexus MR imaging with T2-weighted myelography or high-resolution isotropic T2-weighted imaging could be performed.
Traumatic injury
Trauma is the most common cause of brachial plexus symptoms. Outside of the cord, it can be subdivided into complete or partial root avulsion, stretch injury, and vascular injury. In 1985, Narakas created the rule of “seven seventies” based on a series of 1068 patients, in which 70% of brachial plexopathies are caused by motor vehicle accidents, 70% of these accidents involved motorcycles or bicycles, 70% of these riders had associated multiple injuries, 70% of riders with multiple injuries had supraclavicular injuries, 70% of these supraclavicular injuries demonstrated at least one avulsed nerve root, 70% of avulsed roots involved C7 through T1, or C8 and T1, and 70% of patients with lower root avulsions had persistent pain. The more recent retrospective review of slightly more than 1000 charts of postsurgical patients reported that stretch and/or contusion (49%), laceration (7%), and gunshot wounds (12%) composed almost three-quarters of the injuries to patients. Tumors and thoracic outlet syndrome cases composed 16% each.
Traumatic injuries of the brachial plexus are most commonly seen in the younger population, including neonates and adolescents. The dominant symptom is motor deficit in the appropriate distribution. In addition to motor deficits in the upper extremity, the involvement of C4 and C5 roots, which contribute to the phrenic nerve, is associated with diaphragmatic paralysis. The presence of unilateral ptosis, myosis, and anhidrosis (Horner syndrome) is seen in patients with sympathetic injury at the level of C8 and T1. The postganglionic injury, unlike the preganglionic injury, involving only the motor roots, may have associated sensory component. Although controversial, some of the investigators suggest that the involvement of C7, as demonstrated clinically by loss of sensation in the middle finger and loss of strength in triceps and wrist extensors, prevents patients with C5 and C6 avulsions from undergoing an Oberlin nerve transfer procedure (transfer of redundant motor fascicle of the ulnar nerve to the musculocutaneous branch of the biceps muscle). Therefore, the status of the C7 should be specifically mentioned in the report.
The surgeons categorize the injury by the number of involved spinal nerves (breadth), level of involvement (length), severity of involvement (depth), and time of involvement. In terms of breadth of involvement, the injuries may be separated into complete brachial plexus involvement and partial injury (a single or several spinal nerves). In terms of timing, the plexus injury is divided into the following groups: immediate (less than 3 weeks), post 3 weeks (completion of Wallerian degeneration), and more than 6 months (onset of muscle degeneration). The ideal window for surgical intervention for stretch and/or contusion and gunshot wounds is usually from 3 to 6 months after injury, whereas trauma by sharp mechanisms should be managed as early as possible.
During the immediate presentation, the nerve conduction studies and imaging studies may be misleading because of extensive adjacent posttraumatic changes, incomplete formation of pseudomeningoceles, and incomplete denervation. Therefore, the MR imaging after the injury is usually delayed at least 3 weeks because of the limitations of early imaging, unless the patient develops progressive neurologic deterioration (suggesting an expanding hematoma or pseudoaneurysm) or symptoms of Brown-Séquard syndrome and requires evaluation for presence of myelopathy. The imaging and nerve conduction studies become useful after completion of Wallerian degeneration, when demonstration of nerve root avulsion facilitates early intervention. On the other hand, no invasive treatment is usually performed if nerve conduction studies demonstrate signs of muscle recovery after 3 weeks. Although some primary brachial plexus surgery could be performed after muscle denervation, up to 1 year after the injury, the success rate decreases every month. Tendon and free functional muscle transfers or joint fusions are commonly performed at this stage, more than 2 years after injury.
The mild stretch injury ( Fig. 4 ), predominantly represents neurapraxia (temporary failure of function without structural axonal injury), but includes mild cases of axonotmesis (disruption of axons without interruption of supporting structures), and presents with edema and tortuosity of the affected structures. The axonotmesis cases usually develop edema distal to the site of injury. The abnormalities can be demonstrated on the fat-suppressed T2-weighted images in the oblique coronal plane. Most of the cases of stretch injury improve over time.
Adult traumatic injuries
The nerve root avulsions, termed neurotmesis, can be divided into the preganglionic and postganglionic categories. The latter can be subdivided into complete and partial. In adults, the avulsion injury is associated with high-impact trauma. C5 and C6 are the most commonly injured roots because of their prolonged descent in the neck and susceptibility to traction injury. Based on a series of 26 patients presenting with traction injury ( Fig. 5 ), the most commonly seen abnormalities on MR imaging are (1) traumatic pseudomeningoceles (64%), (2) absence of the roots (64%), (3) lateral cord displacement (45%), (4) hemorrhage and/or scarring in the canal (45%), (5) denervation of erector spinae (27%), (6) syrinx (18%), and (7) spinal cord edema (9%). The CT myelogram has been used traditionally for evaluation of preganglionic lesions due to higher spatial resolution. However, the invasiveness of this technique is its biggest disadvantage, along with its lack of sensitivity for cord injuries. MR imaging using isotropic heavily T2-weighted technique (constructive interference in steady state [CISS] or fast imaging employing steady-state acquisition [FIESTA]) and MR myelography, based on Sampling Perfection with Application-optimized Contrasts using different flip-angle Evolutions (SPACE) sequences, is noninvasive and comparable to CT myelography in its accuracy. In many centers, this limits the use of CT myelography to patients unable to undergo MR imaging. Both CT myelography and MR imaging techniques may demonstrate avulsed roots, hematomas, and (in some cases) pseudomeningocele and displacement of the cord to the contralateral side (see Fig. 5 ). The pseudomeningoceles represent cerebrospinal fluid (CSF) leakage through the dural tear and, therefore, are seen as fluid-filled saccular dural outpouchings on MR imaging and are filled with contrast on CT myelogram.
MR imaging also allows for assessment of concomitant cord abnormalities, stretch injury, and denervation changes. In some cases, it is being combined with three-dimensional (3D) MR neurography (MRN), including multiplanar reconstructions and maximum intensity projections. The MRN studies are usually T2-weighted–based or diffusion-based techniques. The morphology of the neural structures is better defined by the T2-weighted–based techniques. Spectral adiabatic inversion recovery (SPAIR) is increasingly being used in some centers instead of short tau inversion recovery (STIR) imaging due to better signal-to-noise ratio and more favorable specific absorption rate. The diffusion-weighted MRN imaging demonstrates continuity of fibers and their relationship to pathologic lesions. This not only allows for qualitative but also for quantitative assessment of the nerve signal, using apparent diffusion coefficient and fractional anisotropy values. The diffusion tensor imaging–based tractography demonstrates myeloradicular and spinal nerve continuity; however, it is technically difficult because of movement of the adjacent structures, need for large volume coverage, high spatial resolution, and presence of pulsation and susceptibility artifacts. Therefore, it is not routinely used for brachial plexus evaluation. The hybrid diffusion-based MRN techniques, such as 3D diffusion-weighted reversed fast imaging with steady-state precession (3D DW-PSIF), allow for nerve-specific imaging due to strong suppression of the adjacent vascular signal but are more commonly used for lumbar plexus, peripheral, and cranial nerves.
Postganglionic injuries are usually associated with distal nerve retraction and may occasionally demonstrate mild enhancing nodular thickening known as posttraumatic neuroma. Approximately 80% of levels with nerve root avulsions develop pseudomeningoceles; about 15% of levels with pseudomeningoceles do not have an associated nerve root avulsion. Therefore, visualization of the nerve root is of the utmost significance. Even if there is a nerve root avulsion, the residual root within the pseudomeningocele should be identified because it may allow nerve grafting. Approximately 20% of cases demonstrate cord signal abnormality on MR imaging. Administration of contrast may increase the conspicuity of the denervated shoulder girdle and paraspinal muscles as early as 24 hours after injury, by virtue of muscular enhancement and enhancement of the injured portion of the brachial plexus.
Distinction between the preganglionic and postganglionic avulsions influences both management and prognosis. The postganglionic injuries are usually repaired after 3 months of observation to allow for some functional recovery, whereas the preganglionic injuries require early nerve transfers for reconstruction. In severe avulsion cases some surgeons now explore the brachial plexus within the first few weeks, and usually perform nerve transfers as a treatment. Although the procedure can be done up to 12 months after injury, the earlier transfers are performed, the better the outcome.
Neonatal traumatic injuries
Neonatal injuries are seen in up to 0.5% of live births and, depending on the position of the arm during the delivery, may present with superior or inferior plexus injuries. The most common neonatal plexus injury, Erb-Duchenne palsy, usually involves C5 and C6, but may also include C7 nerves. It is associated with supraspinatus, infraspinatus, deltoid, and bicep muscle weakness. The inferior neonatal plexus injury, Klumpke palsy, involves C8 and T1. It presents with hand interosseous and forearm muscle weakness. It may be associated with Horner syndrome. Rarely, there is a question of differential diagnostic considerations. The knowledge of fetal trauma during delivery and the expected recovery course may, in some cases, help determine the need for further evaluation. The most frequently used techniques are isotropic T2-weighted images, such as 3D CISS or 3D T2-weighted turbo spin echo, with multiplanar reconstructions. The most important preoperative question for early (3–4 months) imaging is the presence of preganglionic root avulsion in cases with insufficient recovery following partial plexus injury.
Nontraumatic pathologic conditions
Nontraumatic pathologic conditions include these common categories: acute or chronic infectious or inflammatory brachial neuritis or neuropathy, primary or secondary benign and malignant tumors, aggressive fibromatosis, radiation-induced plexopathy, vascular abnormalities, compression of the plexus, and infection of the plexus. These are better evaluated with MR imaging, except for previously treated malignant tumors in which case a PET-CT scan may be used to differentiate radiation fibrosis from the residual neoplasm.
Radiation plexopathy
The most common nontraumatic inflammatory cause of brachial plexopathy is radiation plexitis. The plexopathy development is dose-dependent, with the risk increasing with both increase in total dose and dose per fraction. Only 1.3% of patients develop radiation plexopathy when the dose is less than 50 Gy, whereas the risk increases to more than 5% when the dose is more than 50 Gy. A recent report of radiation doses of 70 Gy reported 16% incidence of brachial plexopathy. The peak presentation is several years after the treatment, but it has been reported as early as 1.5 months and as late as 22 years after the radiation. At a total dose of 60 Gy, the risk of plexopathy increases from 3.9% at 5 years to 54% at 19 years with annual incidence for grade 1 or higher modified Late Effects Normal Tissue-Subjective, Objective, Management, Analytic (LENT-SOMA) score lesion of 2.9%. The LENT-SOMA scale used for clinical grading in these cases ranges from 1 to 4, from mild sensory deficits to complete motor paresis, excruciating pain, and muscle atrophy ( Table 2 ).
LENT-SOMA Scale | |
---|---|
Grade 1 | Mild sensory deficits without pain |
Grade 2 | Moderate sensory deficits, tolerable pain, mild arm weakness |
Grade 3 | Continuous paresthesia with incomplete motor paresis |
Grade 4 | Complete motor paresis, excruciating pain, and muscle atrophy |
Radiation plexopathy is subdivided into acute and chronic. The time threshold separating the two subtypes is 6 months. The acute radiation plexopathy is considered ischemic, whereas the chronic may be related to fibrosis. There is a suggestion of genetic predisposition. Radiation fibrosis is most frequently related to breast or lung cancer treatment. Use of overlapping fields and chemotherapy increases the risk of this condition. Paresthesias and sensory loss dominate the symptoms; fewer patients have motor function loss. Although there are reports of recovery, the disease is frequently progressive. The upper trunk is more likely to be affected due to its length and absence of protection provided by the clavicle, especially in patients with axillary, infraclavicular and supraclavicular lymph node involvement. The imaging findings usually demonstrate diffuse thickening and distortion of the fibers, edema, and mild enhancement without a focal mass ( Fig. 6 ).
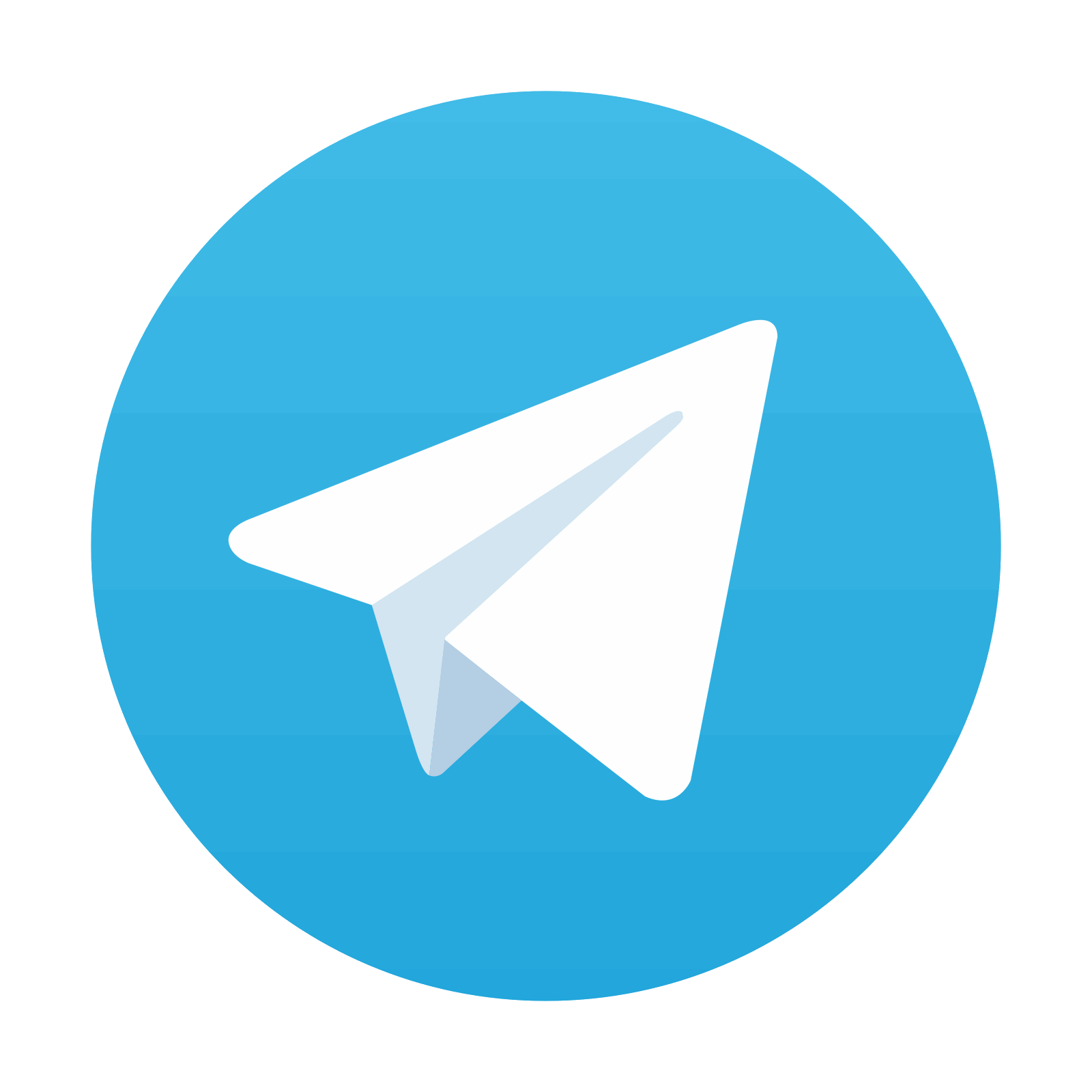
Stay updated, free articles. Join our Telegram channel

Full access? Get Clinical Tree
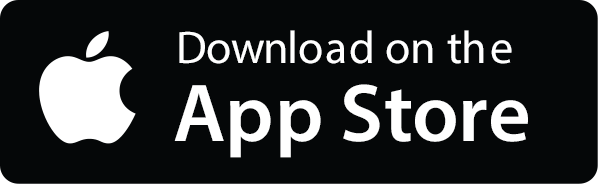
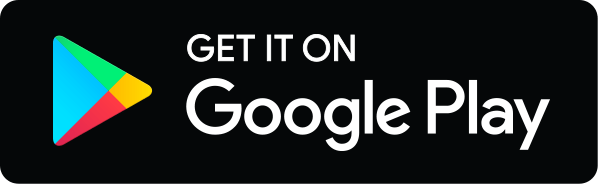
