MR imaging of thoracic veins is performed to evaluate the heart and thoracic vasculature. The protocol can be customized to the clinical question. In the embryo, systemic and pulmonary vein development is closely related to heart development. Congenital anomalies of the thoracic veins are strongly associated with other cardiac and situs abnormalities. Acquired venous abnormalities are often iatrogenic, or secondary to malignancy. This article discusses development and anatomy of the thoracic venous systems, clinical MR imaging methods for their evaluation, and illustrates the MR imaging appearance of congenital and acquired abnormalities of systemic thoracic veins, coronary sinus, and pulmonary veins.
Key points
- •
Anatomic and functional evaluation of the thoracic venous vasculature is achieved with intravenous gadolinium enhanced MRA, “noncontrast” MR techniques and phase contrast imaging.
- •
Congenital anomalies of systemic and pulmonary veins can be explained by disturbances at specific stages of the development of the primordial heart and venous systems.
- •
Acquired abnormalities are most frequently iatrogenic or related to cancer, and infrequently caused by inflammatory disorders.
Normal anatomy and development
Systemic Veins and Coronary Sinus
In the 3-week-old embryo, development of systemic thoracic veins starts with appearance of paired umbilical and vitelline veins, forming the sinus venosus. Anterior and posterior cardinal veins appear in the fourth week. They drain deoxygenated blood from the cranial and caudal portion of the embryo into the sinus venosus via the right and left common cardinal veins ( Fig. 1 A). At this stage, the sinus venosus empties into a common atrium. During the following weeks, a bridging vein develops between the anterior cardinal veins (see Fig. 1 B), which eventually becomes the left brachiocephalic vein (LBCV; see Fig. 1 C). During further development, there is regression of the left anterior cardinal vein, and the right common and anterior cardinal veins form the superior vena cava (SVC), and right brachiocephalic vein (see Fig. 1 C). The azygos and hemiazygos system develops from the embryologic supracardinal veins, except the arch of the azygos vein, which is formed by the right posterior cardinal vein (see Fig. 1 B). The suprahepatic inferior vena cava (IVC) is formed by the right vitelline vein, which also contributes to development of the hepatic veins, whereas the left vitelline vein, right umbilical vein, and suprahepatic left umbilical veins regress (see Fig. 1 B). The right horn of the sinus venosus is absorbed into the developing right atrium and eventually forms the majority of the mature right atrium, except the right atrial appendage, which is the remnant of the primitive right atrium. Transverse portion and left horn of sinus venosus forms the coronary sinus (CS) and the left common cardinal vein develops into the oblique vein of Marshal, a coronary vein running between the left atrial appendage and the left pulmonary veins.
The brachiocephalic veins drain deoxygenated blood from the subclavian and internal jugular veins. The LBCV courses to the right, crosses the midline anterior to the innominate artery, and merges with the right brachiocephalic vein to form the SVC, finally draining into the right atrium. There is no valve within the SVC or between the SVC and the right atrium. Near the superior cavoatrial junction is the location of the sinoatrial node.
The azygos system consists of the azygos, hemiazygos, accessory hemiazygos, and left superior intercostal veins ( Fig. 2 ). It drains blood from inferior body, diaphragm, chest wall, tracheobronchial tree, esophagus, and pericardium. The azygos vein courses in the right posterior mediastinum and arches over the right main bronchus, to drain into the SVC just anterior to the right tracheobronchial angle. The hemiazygos vein courses in the left posterior mediastinum, and crosses the midline at the level of T8–9, to drain into the azygos vein (see Figs. 1 C, 2 A). The accessory hemiazygos vein courses in the left posterior mediastinum, and crosses the midline at the level of T7–8, to drain into the azygos vein. The accessory hemiazygos vein communicates with the left superior intercostal vein, which drains blood from the left second to fourth posterior intercostal veins into the LBCV (see Fig. 2 B, C).
The IVC above the confluence with the hepatic veins courses through the “caval opening,” an opening in the central tendon of the diaphragm, into the thoracic cavity. The supradiaphragmatic portion of the IVC is considered “intrapericardial” because it is covered anteriorly and laterally by pericardium. In the fetal heart, the Eustachian valve at the junction of the IVC with the right atrium directs the blood flow from the IVC toward the foramen ovale and the left atrium. It regresses after birth to a variable extent, and remnants of the Eustachian valve frequently remain as a small crescentic fold at the anterior aspect of the IVC ostium.
The CS drains the majority of deoxygenated blood from myocardium and is located in the posterior portion of the atrioventricular groove ( Fig. 3 ). A variably sized Thebesian valve at its opening is encountered in the majority of pathology specimens.
Pulmonary Veins
Oxygenated blood from the lungs is drained by pulmonary veins into the left atrium. Early development of pulmonary veins is separate from the heart. In the human embryo, lung buds develop after the third week as an outpouching of the foregut. The developing lungs are surrounded by splanchnic mesenchyme, and the pulmonary veins develop as part of the splanchnic venous system, which drains into the cardinal, and umbilicovitelline venous systems. Independently, an outpouching from the primitive left atrium, the primordial common pulmonary vein, develops. The splanchnic pulmonary veins eventually connect with the common pulmonary vein, which starts to drain blood from the primitive lungs. Subsequently, the connections of the splanchnic pulmonary veins with the primordial systemic veins regress. During further development, the common pulmonary vein and, to a variable degree, its central branches are incorporated into the left atrium, forming its posterior portion and resulting in the pulmonary veins connecting individually with the mature left atrium.
Most commonly, there are 2 right and 2 left pulmonary veins ( Fig. 4 A ). However, variations are very common, specifically an additional right middle pulmonary vein (see Fig. 4 B) and a common trunk of the left pulmonary veins (see Fig. 4 C). Normal variants of the pulmonary veins are important in patients who undergo invasive electrophysiologic procedures for the treatment of atrial fibrillation and need to be described in the report of the pulmonary vein MR angiography (MRA) performed before left atrial or pulmonary vein ablation.
MR imaging techniques
Gadolinium Enhanced 3-Dimensional MR Angiography
Gadolinium enhanced 3-dimensional (3D) MRA is an off-label, however, established and robust technique for evaluation of systemic and pulmonary veins. Images are acquired with a T1 weighted 3D spoiled gradient echo (GRE) sequence, usually during breath-hold, and without cardiac gating. For pulmonary vein evaluation, we acquire the images during intravenous bolus injection of 0.15 mmol/kg gadolinium contrast material, injected at a rate of 2 mL/s, and image acquisition is timed for enhancement of the left atrium. Systemic veins can be imaged using the same injection, by repeat acquisition during the early equilibrium phase, when gadolinium contrast material has reached the venous compartment, but has not distributed into the extracellular space. As an alternative, dedicated 3D MRA of the SVC and its tributaries can be performed directly, during bilateral or unilateral upper extremity vein injection of a 20:1 dilution of normal saline and gadolinium contrast material. This method generates images that are relatively free of arterial contamination.
Image interpretation benefits from postprocessing, such as reformatting of the 3D dataset in multiple planes (see Fig. 2 ), maximum intensity projections, and volume rendering (see Fig. 4 ). Gadolinium-enhanced MRA is contraindicated in patients with prior anaphylactic reaction to gadolinium contrast material. Because of the risk of nephrogenic systemic fibrosis, gadolinium injection is also contraindicated in patients with advanced renal failure and in dialysis patients.
“Noncontrast” MR imaging Techniques
MR techniques for evaluation of the vasculature without gadolinium administration are broadly categorized into white blood, black blood, and phase contrast acquisitions.
Bright blood imaging
Bright blood imaging was originally performed with spoiled GRE sequences. A time of flight acquisition utilizes a spoiled GRE sequence with a high flip angle and a short repetition time. To evaluate the SVC for thrombus, a stack of consecutive 2D time of flight images in the transverse plane of the chest can be acquired ( Fig. 5 A, B ).
Currently, for imaging of the vasculature without gadolinium, GRE sequences have been largely replaced by 2-dimensional (2D) and 3D steady-state free precession (SSFP) acquisitions. SSFP is less sensitive to flow, but provides excellent contrast between fluid and soft tissue: the signal depends on the ratio of T2/T1, which is particularly high for fluids including blood. Two-dimensional SSFP images of the thoracic vasculature can be acquired with or without cardiac gating, during breath-hold or during free breathing. A vectorcardiogram (VCG)-gated 2D SSFP cine acquisition with about 20 to 30 reconstructed phases per heart beat and a slice thickness of 6 to 8 mm depicts accurately systemic and pulmonary veins, and also provides excellent visualization of the CS (see Fig. 3 ). The 3D SSFP technique is described in detail elsewhere in this article.
Black blood imaging
Black blood images of blood vessels are acquired with double inversion recovery prepulse technique, a flow-sensitive method to null the signal of flowing blood. For routine black blood imaging of systemic and pulmonary veins, we use a VCG-gated proton density weighted 2D fast spin echo sequence with a slice thickness of 6 to 8 mm. The slice thickness can be decreased to as low as 2 mm when imaging small children. As a minimum, we acquire a stack of consecutive slices in the transverse plane of the chest, with additional imaging planes as necessary (see Fig. 5 C).
Phase contrast imaging
Phase contrast sequences measure flow velocity and provide information about direction of flow. Flow volume per minute is calculated from flow velocity and cross-sectional vessel area using dedicated postprocessing software. The main application of phase contrast imaging, apart from flow quantification through specific vessels, is shunt quantification by comparing flow volumes per minute in pulmonary (Qp) and systemic (Qs) circulations.
Vectorcardiogram- and respiratory-gated 3-dimensional acquisitions
These acquisitions are advantageous for motion artifact–free imaging of small vessels. They acquire near isotropic 3D images with high spatial resolution during free breathing. Cardiac and respiratory motion compensation is achieved by simultaneous VCG gating and tracking of diaphragmatic motion (respiratory navigator technique). A VCG- and respiratory navigator-gated 3D SSFP sequence with a voxel size of about 1.2 to 1.6 mm can be used without gadolinium (see Fig. 5 D). However, gadolinium administration improves image quality by increasing the signal-to-noise ratio, particularly at small voxel sizes. Also available with the respiratory navigator technique are VCG-gated 3D inversion recovery GRE sequences. They can be utilized to acquire T1 weighted, gadolinium-enhanced, high-resolution images of the pulmonary and coronary veins and are particularly suited to be used with new gadolinium-based “blood pool” contrast agents. Intravenously injected blood pool agents have a much longer plasma half-time than conventional contrast agents, which quickly distribute into the extracellular space. The long duration of the equilibrium phase allows for imaging with increased spatial resolution, and with cardiac gating ( Fig. 6 ). Gadofosveset is the first gadolinium-based blood pool agent to be approved by the US Food and Drug Administration. It requires the same safety precautions as conventional gadolinium-based contrast agents and should be avoided in patients with acute kidney injury or a glomerular filtration rate of less than 30 mL/min/1.73 m 2 .
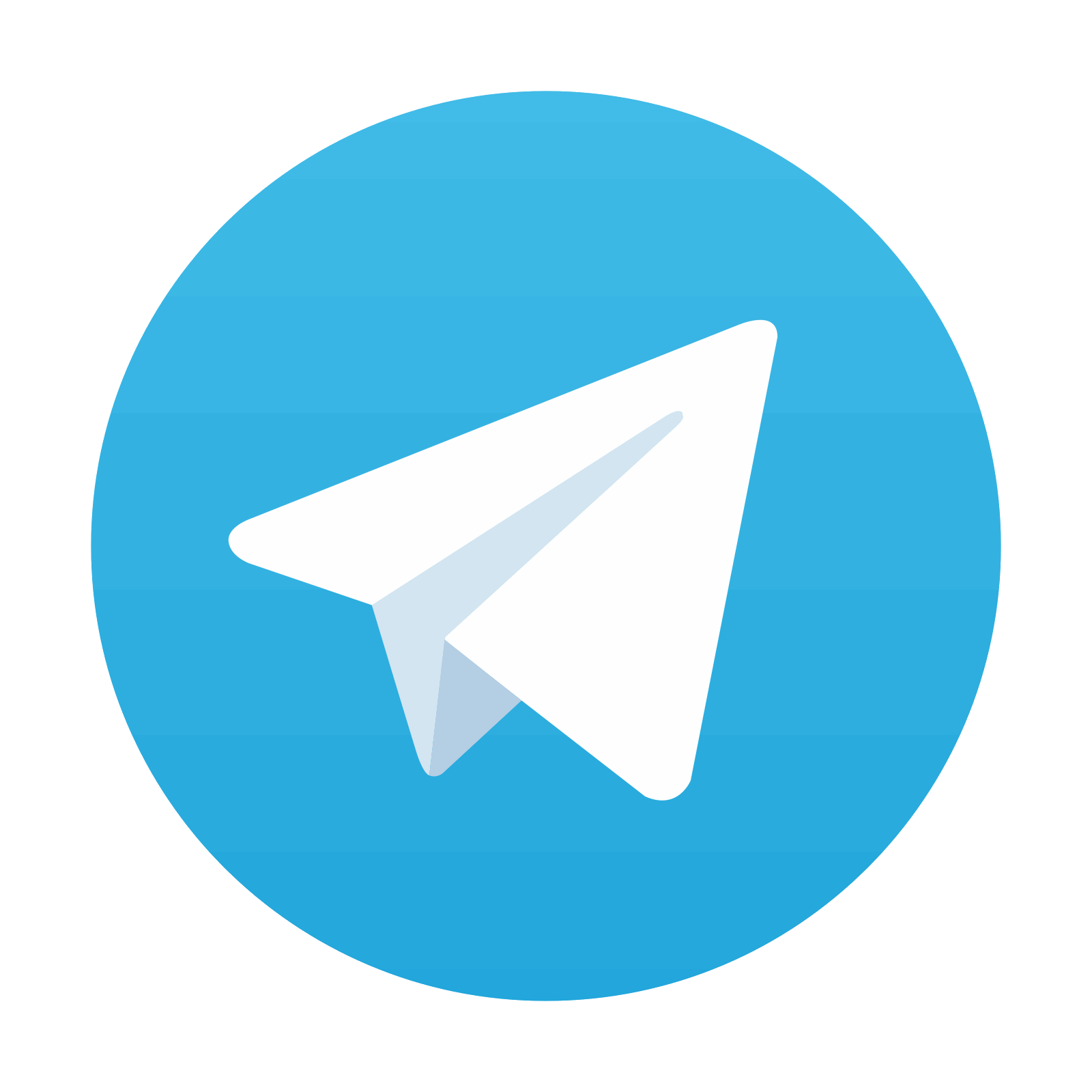
Stay updated, free articles. Join our Telegram channel

Full access? Get Clinical Tree
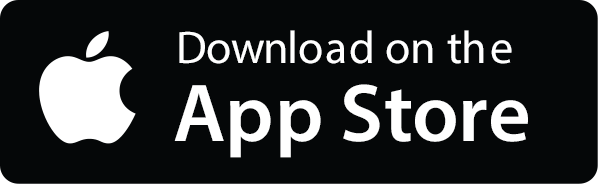
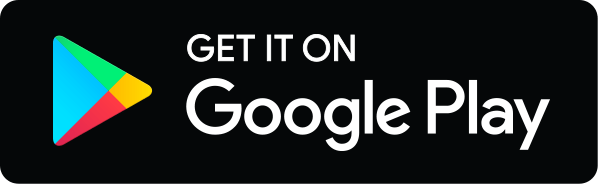
