Fig. 42.1
Grade I lesion image of feathery edema-like pattern with intramuscular high signal on the fluid-sensitive sequences, with no discernible muscle fiber disruption (arrow) and adjacent to distal quadriceps tendon (arrowhead)
In grade II injury, a partial tear is macroscopically evident, with some continuity of fibers at the injury site (Slavotinek 2010) (Fig. 42.2a, b). Less than one third of fibers are torn in low-grade injuries, from one third to two thirds in moderate ones, and more than two thirds in high-grade injuries. Muscle strength and high speed/high resistance athletic activities are impaired, with marked loss of muscle function. At US, muscle fibers are discontinuous, the disruption site is hypervascularized, and echogenicity is altered in and around the lesion (Lee and Healy 2004). At MRI, appearance varies with both the acuity and the severity of the partial tear, changes are time dependent, and edema and hemorrhage of the muscle or MTJ may extend along the fascial planes, between muscle groups. MRI can sometimes be predictive of the time high-performance athletes will be away from play.
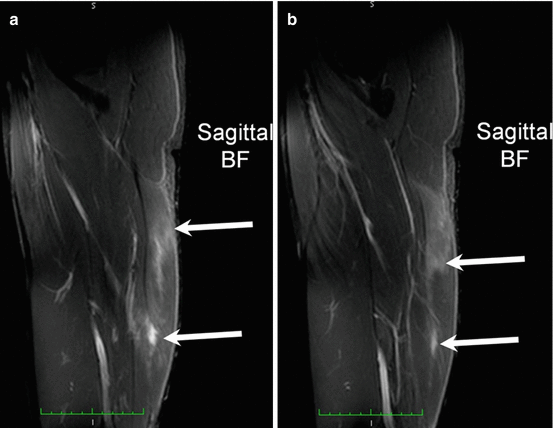
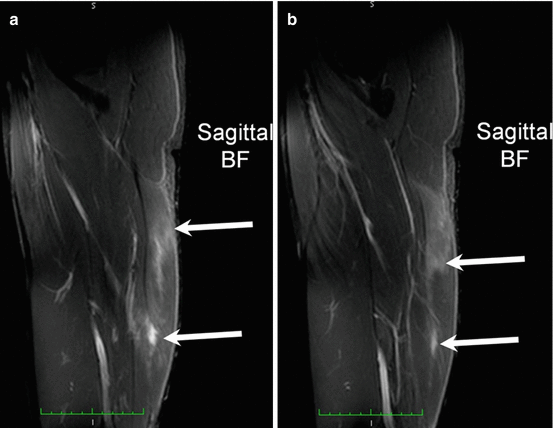
Fig. 42.2
(a, b) Grade II tear edema (arrow) and hemorrhage of the muscle or MTJ extending to the fascial planes of biceps femoris (arrow)
A grade III injury is a complete tear (Fig. 42.3a, b). At US and MR imaging, these injuries show complete discontinuity of muscle fibers, hematoma, and retraction of the muscle ends (Lee and Healy 2004). Clinically, muscle function is lost. When extensive edema and hemorrhage fill the defect between the torn edges, it is difficult to distinguish partial from complete tears, whereas real-time dynamic US imaging may be helpful (Koh and McNally 2007).
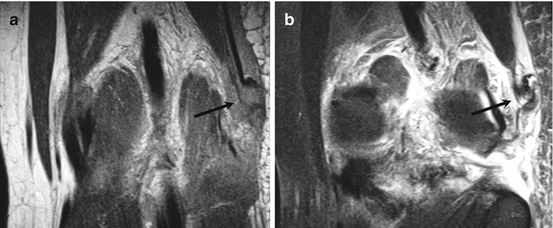
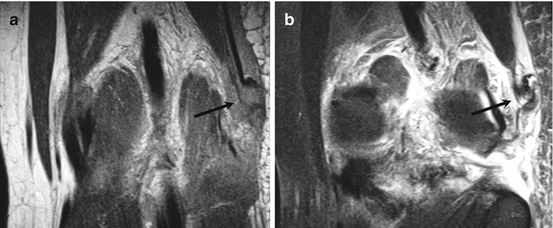
Fig. 42.3
(a, b) Grade III tear showing femoral biceps muscle and avulsed MTJ from fibula head with complete avulsion of musculotendinous junction and associated large amount of edema with complete interruption of muscle fibers and associated hematoma
42.3 Predisposing Factors
Traumatic muscle injuries vary on the directions and angle movements of forces applied. Contusions, strains, or lacerations may be distinguished (Garrett 1996). Contusions and strains account for more than 90 % of all sports-related skeletal muscle injuries; lacerations are uncommon (Jarvinen and Lehto 1993). Contusions occur in contact or combat sports after application of large compressive forces on the muscle. Muscle strains, very common in sprinters and jumpers (Crisco et al. 1994, Garrett 1996), usually arise from an indirect trauma, from application of excessive tensile forces. Muscle lacerations, rare in athletes, arise from direct blunt trauma to the epimysium and underlying muscles (Koh and McNally 2007).
Three types of muscle are at possible risk for injury:
1.
Two–joint muscles. In such instance, the motion at one joint may increase the passive tension of the muscle and lead to an overstretch injury.
2.
Muscles contracting eccentrically. Concentric and eccentric contractions are normally performed in functional activities. Specifically, eccentric contractions, common in the deceleration phase of activity, may induce acute strains by producing specific tensions which lead to myofiber overload injury (Friden et al. 1983).
3.
Muscles with a higher percentage of type II fibers. These are fast-twitch muscles, in which speed of contraction produced is greater than in other muscles, predisposing a muscle to injury. The fact that most of the muscle action involved in running and sprinting is eccentric, muscle strains most often occur in sprinters or “speed athletes.” In these sports, the muscles more susceptible to be strained are the hamstrings, gastrocnemius, quadriceps, hip flexors, hip adductors, erector spinae, deltoid, and rotator cuff.
The coordination and balance between agonist and antagonist muscles have to be taken into account. Specifically, flexibility imbalances between agonists and antagonists may predispose to injury. Flexible muscles are most likely to be injured (Page 1995). A previous injury makes more vulnerable to reinjury, justifying that sprinters with recent hamstring injuries have tighter and weaker hamstrings than uninjured muscles (Garrett 1996).
When rehabilitation is inadequate, strength, flexibility, and endurance may not be completely restored before return to activity. Therefore, residual weakness and impairment may predispose the muscle to a new injury. From the assumption that cold or tight muscles are more predisposed to muscular strain, proper stretching exercises and warm-up may prevent muscular injury (Strickler et al. 1990). After warm-up, muscle elongation before failure is increased. In addition, since warm muscles (40 °C) are less stiff than cold (25 °C) muscles, warm-up may prevent and enhance performance (Noonan et al. 1993).
42.4 Structural Changes
The most vulnerable site for an indirect strain injury is the musculotendinous junction, the weakest link within the muscle tendon unit (Bach et al. 1987). In eccentric muscle actions, when muscle tension increases suddenly, the damage may occur in the area beneath the epimysium and the site of muscle attachment to the periosteum (Garrett 1990). In fascial injuries, common in the medial calf and biceps femoris, differential contractions of adjacent muscle bellies may produce aponeurotic distraction injuries (Koulouris and Connell 2005; Malliaropoulos et al. 2011). Hamstring strain muscle injuries, the most widely studied, typically occur in the region of the MTJ, a transition zone organized in a system of highly folded membranes, designed to increase the junctional surface area and dissipate energy. The region adjacent to the MTJ is more susceptible to injury than any other component of the muscle unit, independently from type and direction of applied forces and muscle architecture (El-Khoury et al. 1996). In this area, even a minor strain, by inducing an incomplete disruption, evident only at microscopy, may weaken it and predispose to further injury. Disruptions in the fibers cause biochemical changes both from direct injury to the fibers and from the inflammatory reaction.
42.5 Biochemical Changes
Serum creatine kinase (CK) and lactate dehydrogenase (LDH) enzyme levels are used to indirectly assess muscle damage following eccentric exercise (Stauber 1989). These biochemical markers are released after the insult. In addition, inflammatory reactions occur. Acute inflammation is designed to protect, localize, and remove injurious agents from the body and promote healing and repair (Askling et al. 2007). Chemical inflammatory mediators are present in acute muscular strain, such as histamine, serotonin, bradykinin, and prostaglandin. The capillary membrane permeability is increased, blood vessel diameter is changed, and pain receptors are stimulated. As consequence, the accumulation of proteins and transudate in the interstitial space produces edema. Therefore, swelling, heat, redness, and pain of inflammation are due to biochemical changes stimulated by chemical mediators. Inflammatory reaction and edema occur 1–2 days after a stretch-induced muscular injury (Nikolaou et al. 1987). The acute phase of inflammation lasts up to 3–4 days after the initial insult. Proliferation of fibroblasts, increased collagen production, and degradation of mature collagen weaken the tissue. In this way, stretching the tissue induces progressive irritation and limitation, up to predisposition to chronic muscle strains. When the inflammatory phase subsides, repair is started, for 2–3 weeks. Specifically, capillary growth and fibroblast activity to form immature collagen are promoted. This immature collagen is easily injured if overstressed. The final stage of healing is maturation and remodeling of collagen, occurring from 2 to 3 weeks after the insult, until patients are pain-free. In the healing phase, if fibers are not properly stressed, surrounding adhesions and scar resilient to remodeling may be formed.
42.6 Treatment of Acute Strains
Management varies on the severity of the injury, the natural healing process of the body, and the response of the tissue to new demands.
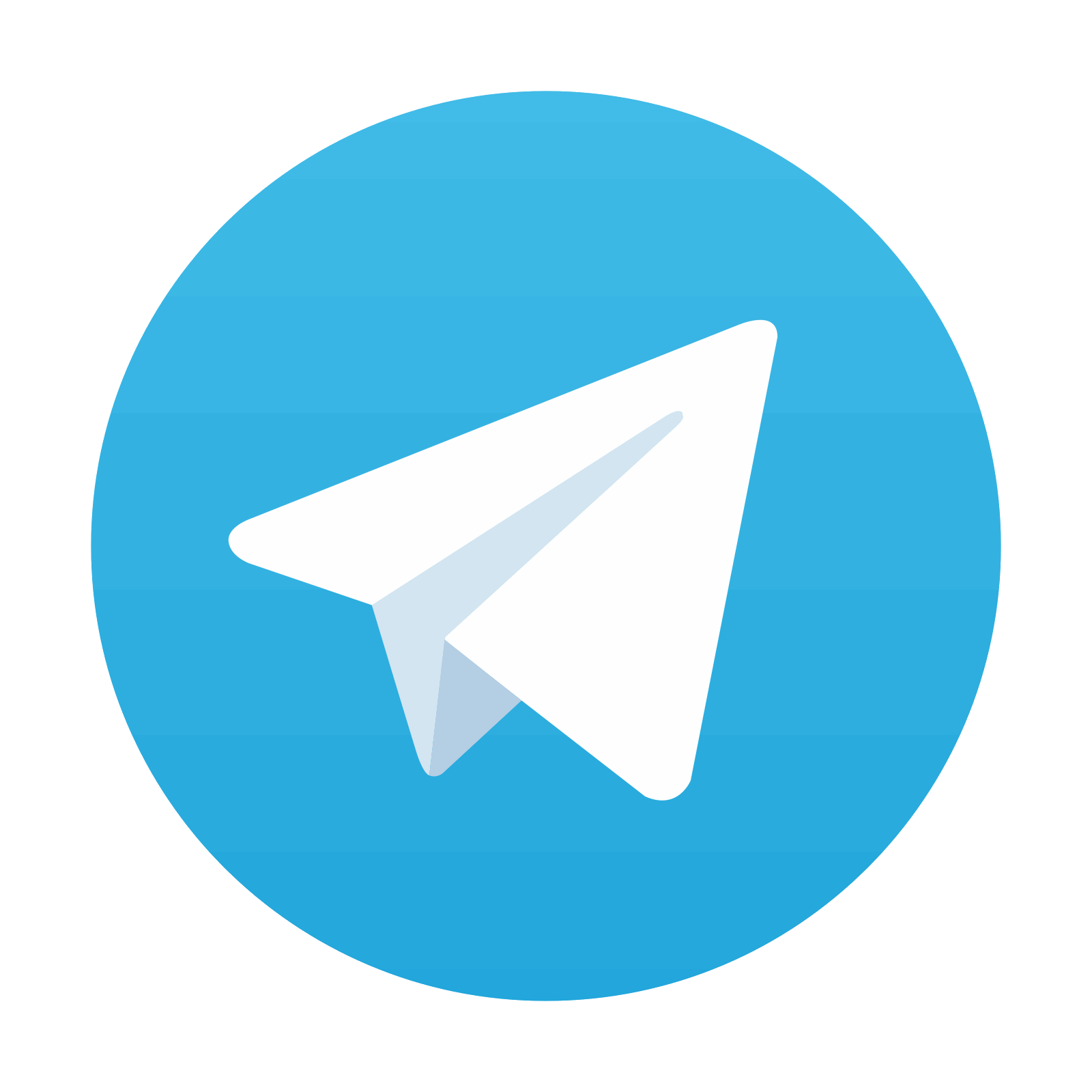
Stay updated, free articles. Join our Telegram channel

Full access? Get Clinical Tree
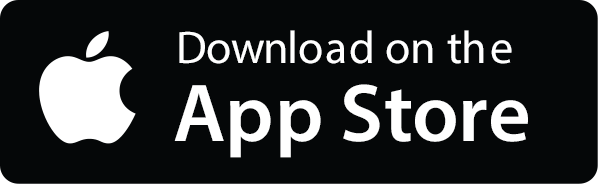
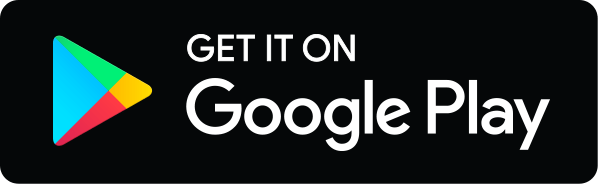