Juan Manuel Cepparo, MD
Laurent Vandenbusche, MD
Ramon Gheno, MD
Jader José da Silva, MD
Anne Cotten, MD, PhD
Ultrasound is a wonderful imaging modality for the assessment of the soft tissues. It is noninvasive, cost-effective, and widely available. It allows real-time dynamic assessment of muscles, tendons, and nerves and can generate images with greater spatial resolution than that obtained with standard clinical MRI techniques. The aim of this chapter is to present the usefulness of ultrasound in the assessment of the main soft tissue disorders.
ULTRASOUND TECHNIQUE
General Aspects
The development of compact real-time ultrasound systems in the 1980s and the subsequent availability of broadband high-frequency linear-array transducers in the 1990s have dramatically improved the accuracy of clinical assessment of musculoskeletal disorders.1 The choice of transducer frequency depends on the overall size of the structure, its position relative to the skin (deep or superficial), and the conspicuousness of the intervening soft tissue planes. Superficial structures require small-sized probes with high frequencies (7.5–20 MHz), while deep structures require low-frequency probes (3.5–5 MHz).1
The brightness of the images depends on the degree of reflection of the ultrasound waves and is defined as echogenicity. The echogenicity of a tissue depends on the characteristics of that tissue and on the transducer frequency. The terms used to describe such characteristics include hyperechoic (white signal), isoechoic, hypoechoic, and anechoic (black) echogenicity. In general, bones and gas-like structures are most echogenic and fluids, such as joint effusion, and are less echogenic.1
Advantages
Assessing soft tissue disorders via ultrasound has many advantages as compared with MR imaging:1–5
• It is well tolerated and has no contraindication, unlike MR imaging.
• It can effectively image patients with orthopedic hardware that causes significant artifacts in MR imaging.
• It is noninvasive and does not require intravenous contrast administration in the clinical practice, as it can provide information about blood flow and the degree of tissue vascularity thanks to the use of color Doppler and power Doppler.1–3,6
• It is cost-effective and widely available. An ultrasound examination of an extremity costs about three times less than a magnetic resonance imaging (MRI) examination.7
• It allows real-time dynamic assessment of muscles, tendons, and nerves. Using passive and active movements, or isolated muscle contractions, is fundamental for the depiction of tendon and bursal impingements (such as subacromial impingement), nerve impingements, tendon and nerve instability and muscle herniation, as well as to improve the depiction of small lesion such as tears.8,9
• It facilitates bilateral comparison. A wide degree of anatomic variability exists in the musculoskeletal system. Consequently, the detection of bilateral asymmetry can be useful when deciding whether an abnormality is present.
• It has a more flexible field of view than MR imaging and is the preferred imaging technique for structures with a long course in the body, such as peripheral nerves.
• It can generate images with greater spatial resolution than that obtained with standard clinical MRI techniques and is particularly useful for the assessment of tiny structures (such as tendons and nerves) or calcifications.
• It allows the placement of the probe precisely at painful points; this is particularly useful when several abnormal features are present in the same area, as well as for interventional treatments, such as infiltration.
• It can be used to guide a wide range of musculoskeletal interventions, such as joint aspiration, synovial or soft tissue biopsy, and joint or tendon sheath injection.10
Limitations
However, ultrasound has some limitations that must be kept in mind:
• Analysis of the bone is limited to its surface.
• Analysis of joint spaces is limited to their superficial part.
• Detailed analysis of deep structures can be difficult, particularly in overweight people.
• Each patient has variable echogenicity.
• Rereading the images is difficult.
• A detailed knowledge of anatomy is mandatory for the correct assessment of soft tissue disorders.
TENDONS
Normal Appearance
In the longitudinal plane, tendons have a fibrillar pattern with parallel hyperechoic lines (collagen fibers) in a structure evidencing intermediate echogenicity (matrix proteoglycan). In the transverse plane, they have a hyperechoic round to ovoid appearance and contain bright stippled clustered dots (Figure 19-1).4,10
Figure 19-1. Normal calcaneal tendon (Achilles tendon). (A) Long axis demonstrating the fibrillar pattern of the tendon (arrow) with parallel hyperechoic lines. (B) Transverse plane demonstrating an ovoid tendon (arrow) containing bright stippled clustered dots.
Tendons are strongly anisotropic structures, and fibrillar echoes can be efficiently demonstrated only when the ultrasound beam is perpendicular to them. Even a slight obliquity of the angle of incidence can result in artifactually decreased echogenicity, which may obscure textural details and even mimic tendon disease (Figure 19-2).11 They must therefore be studied in two orthogonal planes in order to differentiate lesions from anisotropy. Tendons should also be examined with the patient at rest and during active and/or passive motion. Normal tendons do not contain significant Doppler signal.
Figure 19-2. Normal biceps tendon (arrows). Long axis of a normal long head of the biceps tendon (arrow) with the ultrasound beam perpendicular to it (A) showing its fibrillar aspect, and with a slight obliquity of the ultrasound beam (B) resulting in artificially decreased echogenicity, which may mimic tendon disease.
Disorders
Ultrasound is probably the best imaging modality for the assessment of tendon disorders, as this technique allows the assessment of the following:
Tendinosis: This is a degenerative change of the tendon related to aging or repeated microtrauma. Ultrasound demonstrates diffuse or focal tendon thickening with areas of intratendinous hypoechogenicity and loss of fibrillar echoes, reflecting a disorganized structure of the collagen bundles (Figure 19-3).12
Figure 19-3. Supraspinatus tendinosis. Long axis of the supraspinatus tendon, demonstrating tendinosis characterized by a diffuse thickening with areas of intratendinous hypoechogenicity and loss of fibrillar echoes, reflecting a disorganized structure of the collagen bundles (arrows). GT = greater tuberosity.
Calcifying tendinopathy: Any tendon can be affected, but this disorder is particularly frequent at the rotator cuff. The pathogenesis of calcium deposition in tendons is not completely understood. It has been suggested that the calcification goes through phases. The initial deposition is followed by a latent-period formative phase, during which the calcification is hard (type I). Ultrasound demonstrates an intratendinous hyperechoic focus, with well-defined acoustic shadowing (Figure 19-4A). Most type I calcifications are asymptomatic, unless they are large enough or superficially located in the tendon and impinge on the adjacent structures during movement (one example is impingement on the acromion while abducting the arm in internal rotation). These calcifications may undergo chronic and asymptomatic reabsorption or acute reabsorption, with excruciating pain for several days or weeks. Ultrasound demonstrates slurry fragmented calcifications with a faint (type II) (Figure 19-4B) or absent (type III) shadow (Figure 19-4C). Adjacent bursitis can be demonstrated, particularly during the acute phase. Adjacent soft tissue hyperemia is typically encountered during acute painful reabsorption of a calcification.8,11
Figure 19-4. Calcific tendinosis. Long axis of the supraspinatus tendon demonstrating (A) intratendinous hyperechoic focus (arrows), with well-defined acoustic shadowing (arrowheads) (type I); (B) slurry calcification (arrows) with a faint shadowing (arrowheads) (type II); (C) Ill-defined calcification (arrows) with no shadow (type III).
Partial tears: These are defined by incomplete interruption of the tendinous fibers. When they involve the short axis of the tendon, ultrasound demonstrates focal defects filled with fluid or blood (liquid, anechoic areas) (Figure 19-5A).1,12 In the rotator cuff, when the partial tear involves the bursal aspect of the tendon, focal herniation of the adjacent hypoechoic bursal fluid or hyperechoic peribursal fat within the defect can be seen (Figure 19-5B).11 When the tear involves the long axis of the tendon, ultrasound demonstrates hypoechoic or anechoic longitudinal splits that separate the tendon into two distinct parts (Figure 19-5C). This kind of tear is frequently seen in ankle tendons.4
Figure 19-5. Partial articular surface tear of the supraspinatus tendon. Long axis of the supraspinatus tendon demonstrating (A) partial rupture of its articular surface, filled with fluid (anechoic area, arrow); (B) partial tear of its bursal aspect with focal herniation of the adjacent hyperechoic peribursal fat (arrow) and deltoid muscle into the gap. There is also a partial tear of its articular surface, filled with fluid (*); (C) Partial tear of the articular surface of the tendon, filled with fluid (*), extending proximally along the long axis of the tendon (arrows). The bursal aspect of the tendon is indicated by small arrowheads. GT = greater tuberosity.
Complete tears: Depending on the age of the rupture and the degree of retraction of the proximal part of the tendon, ultrasound may demonstrate a focal interruption of all the fibers (anechoic gap), a proximal retracted tendon, or a lack of visualization of the tendon. At the rotator cuff, a prominent reflection of the ultrasound beam at the interface between fluid and articular cartilage, commonly called “uncovered cartilage sign” or the “cartilage interface sign,” can be demonstrated (Figure 19-6).11–12 It is important to measure the dimensions of the gap, describe the appearance of the tendon edges bordering on the tear, and look for associated lesions, such as osseous avulsions that manifest as hyperechoic focus with posterior acoustic shadow.
Figure 19-6. Complete rupture of the supraspinatus tendon with retraction. (A) Complete rupture of the supraspinatus tendon with retraction of its proximal part (*). Notice the surface of the articular cartilage, characterized by a bright linear echo, the “cartilage interface sign” (arrows). The dotted line shows the length of the tear and retraction. (B) Normal supraspinatus tendon on the contralateral side for purposes of comparison (arrowheads). GT = greater tuberosity.
Tenosynovitis: Some tendons, especially those of the hands and feet, have a synovial sheath represented by a thin anechoic layer, indicating a small amount of synovial fluid.13 Tenosynovitis, which can have mechanical or inflammatory causes, is characterized by a widening of the tendon sheath that typically encircles the tendon (Figure 19-7).13 This peritendinous ring corresponds to a thickened synovium and/or an effusion within the tendon sheath. Compression with the probe during the examination can help differentiate between these two components (the effusion can be compressed).13 The use of Doppler (color or power) allows the detection of hyperemia within the synovium, indicating active synovitis.13 In the presence of tenosynovitis, the tendons may be either normal or abnormal.
Figure 19-7. Finger flexor tendons of a patient with rheumatoid arthritis presenting tenosynovitis. (A) Long axis demonstrating a widening of the sheath (arrows) encircling the tendons (*). (B) Short axis of the tendons (*) demonstrating the hypoechoic ring (arrows are showing fluid in the tendon sheath). M = metacarpus, P = phalanges.
Tendon instability: This mainly affects the long head of the biceps tendon and the fibular tendons.4 Congenital hypoplasia of the bone groove and congenital laxity or traumatic lesions of the fibrous roof of a tendon tunnel may predispose to tendon instability. Ultrasound demonstrates the abnormal location of the tendon and the degree of instability (intermittent or permanent subluxation, dislocation), as well as associated findings (tenosynovitis or tendinous lesions due to abnormal friction of the displaced tendon against a bony edge).4 Transverse planes allow a better assessment of tendon dislocation, because both the empty tunnel and the displaced tendon can usually be seen in a single image (Figure 19-8).4 Dynamic examination is fundamental in order to enhance the detection of intermittent subluxation and assess the possibility of spontaneous reduction.11,14
Figure 19-8. Subluxed extensor tendon of the finger. Transverse ultrasound images of a finger extensor tendon subluxation (arrow) lying in an ulnar instead of a central position (*). MH = metacarpal head.
Peritendinopathy: Inflammatory changes of the soft tissues, particularly the fat surrounding the tendons, can be observed in association with all the above tendon disorders.12
Paratendinopathy: This disorder is mainly demonstrated at the calcaneal tendon. Instead of a synovial sheath, this tendon has a connective tissue envelope, or paratenon, which may be injured, thickened, or hypoechoic, with increased vascularity in the lesions affecting the tendon (Figure 19-9).15
Figure 19-9. Achilles tendon. Short axis of a calcaneal tendon (Achilles tendon) demonstrating (A) normal paratenon that appears as a hyperechoic envelope (arrows) in continuity with the subcutaneous fat; (B) paratendinopathy characterized by a thickened, hypoechoic paratenon (arrows).
LIGAMENTS
Like tendons, ligaments have a fibrillar pattern, but their fibers may run in different directions.1 Ligamentous injuries pose a challenge when using traditional imaging modalities, as they require a truly dynamic assessment. Ultrasound is now firmly established here, as it can provide a rapid noninvasive and dynamic assessment of these injuries, particularly those affecting the lateral ligament complex of the ankle and the thumb-stabilizing ligament. Partial or complete tears, sequelae of rupture (such as increased thickness, calcifications, or ossifications), and ligament instability can be evidenced by dynamic movements (Figure 19-10).16
Figure 19-10. Normal anterior talofibular ligament. Long axis of a normal anterior talofibular ligament running from the lateral malleolus to the talus, with a fibrillar aspect (arrows). This ligament is the one most often damaged in ankle injuries.
Ultrasound is also an excellent method for the analysis of other connective tissue structures such as pulleys and retinacula.
ENTHESIS
Enthesis is the point of union between bone and a tendon, ligament, aponeurosis, or capsule.11 This structure is mainly injured in overuse disorders (mechanical enthesopathy). However, it is also the preferred site of development of inflammatory changes (enthesitis) in spondyloarthritis.13 Ultrasound demonstrates increased thickness, hypoechogenicity (Figure 19-11), and hyperemia of the enthesis on power Doppler.17 With time, erosions and/or hyperostosis of the adjacent bone and calcifications or ossification at the site of insertion of the tendon may be seen.13,18
Figure 19-11. Normal plantar fascia. Insertion of the plantar fascia (arrows) into the calcaneus (*), demonstrating the normal aspect of an enthesis.
MUSCLES
Muscles must be examined from origin to insertion. A systematic evaluation of the enthesis, musculotendinous junction, intramuscular septa, and epimysium (the dense fibrous tissue surrounding the muscle belly) must be performed in both the longitudinal and transverse planes.3
Normal Appearance
Muscle fibers are hypoechoic. The perimysium (the loose areolar tissue surrounding the muscle fibers) appears as linear echogenic bands within the muscle belly on longitudinal images and as echogenic dots, called “starry night” appearance, on transverse images (Figure 19-12).3 Like tendons, muscles are subject to anisotropy artifacts, as the orientation of their fibers is variable. Muscle fibers are usually arranged in parallel fashion, forming a sort of pennate structure. Muscles can be unipennate, bipennate with a central aponeurosis or circumpennate.19 This aspect is well differentiated by ultrasound.
Figure 19-12. Normal medial head of the gastrocnemius muscle. (A) Longitudinal aspect showing multiple hyperechoic lines (arrows) consistent with perimysium. Note the oblique course of these echoes. (B) Transverse aspect showing the perimysium as echogenic dots (arrows) inside the hypoechoic muscle.
Traumatic Lesions
Muscle injuries account for approximately one-third of all sports-related injuries3 Ultrasound is mainly useful in order to demonstrate the precise location and severity of the injury and guide the return-to-activity time frame. Indeed, the absence of abnormality on ultrasound is commonly accepted as a prerequisite marker of healing sufficient for the resumption of physical activity.3
Muscle injuries may be grouped into two main classes:
Intrinsic injuries (strains): These most often result from powerful stretching when the muscle is contracted and can lead to disruption of muscle fibers near the myotendinous junction, which is considered to be the weakest area of the muscle-tendon-bone unit.11 Depending on the severity of the lesions, different features may be observed; on the basis of these, various grading systems have been developed, as there is no single universally accepted classification system (Table 19-1).3 In the daily practice in sports medicine, however, it is not grading that is crucial, but rather distinguishing between muscle lesions without any bundle tears and lesions with bundle tears and intramuscular hematomas. The former heal in 1–2 weeks, while the latter ones require at least a 4-week interruption of sports activities.19
Table 19-1. Grading Muscle Strain Injuries Using Ultrasound3
Extrinsic injuries: These result from external trauma (contusion or penetrating injury, i.e., laceration) and are typically located at the actual site of the trauma.11
In both intrinsic and extrinsic lesions, the injured muscle is enlarged and demonstrates an edematous/hemorrhagic hyperechoic infiltration. The area of the rupture is occupied by a more or less echogenic hematoma. Hematoma is the key sign of a muscle tear; its appearance depends on the time elapsed between the trauma and the ultrasound examination. In the vast majority of cases, it appears as a hypo- or anechoic circumscribed lesion (Figure 19-13). However, there are some exceptions that must be kept in mind. In the very first hours following the trauma, the hematoma may still be diffuse and not collected and appear either as a hyperechoic area of the muscle or as an abnormal gap between muscle bundles. Other subtle signs must then be looked for, such as retraction of the fibers or presence of a subcutaneous ecchymosis on the patient’s skin.3,19 When large enough, hematomas may be compressive, resulting in compartment syndromes and/or compression of adjacent vessels and nerves. In such cases, a needle evacuation of the hematoma can be proposed.19
Figure 19-13. Muscle contusion tears and hematomas. (A) Partial tear of the semimembranosus muscle (SM) close to its aponeurosis (*), with an anechoic hematoma (arrow) (transversal axis), B = biceps muscle. (B) Rupture of the distal portion of the medial gastrocnemius (MG) that is retracted proximally; a fluid collection (arrow) distal to the avulsed medial head is observed (longitudinal axis). (C) Extensive contusion of the vastus intermedius (VI) muscle, with a large and heterogeneous hematoma (the tear is measured by the dotted line) (longitudinal axis). (D) Contusion over the VI and lateralis (VL) muscles in the anterolateral portion of the thigh, demonstrated by an ill-defined hyperechoic area (arrows) (longitudinal axis). This aspect is related to edema and hemorrhagic changes.
Muscles injuries can lead to residual lesions:
Fibrous scars: The persistence of fibrous scars is common and should not prevent the resumption of sports activities. They appear as hyperechoic areas, sometimes accompanied by a posterior acoustic shadow (small calcifications) (Figure 19-14). They represent areas of weakness, which may foster a further rupture.
Figure 19-14. Fibrous scar inside the muscle. Fibrous scar demonstrated by a well-defined hyperechoic area inside the femoral biceps muscle, following a past injury (arrow).
Calcifications and ossifications: These hyperechogenic areas with posterior acoustic shadowing are also areas of weakness and may represent an evolution of fibrous scars.
Encysted hematomas: These hypoechoic or anechoic lesions are compressible and floating. They may be surrounded by a peripheral pseudocapsule. When necessary, they can be punctured.19
Muscle herniation: Ultrasound demonstrates interruption of the hyperechoic fascial layer and focal extrusion of muscle tissue within the subcutaneous fat. Muscle herniations are better characterized by resorting to dynamic maneuvers (muscle contraction).11,20
Muscle Atrophy and Fatty Replacement
These changes can occur after tendon or muscle injuries, muscle denervation, or disuse.3 Ultrasound demonstrates reduction in the volume of the muscle and fatty replacement, which increases the muscle’s echogenicity. Comparison with the contralateral side is useful, particularly in the case of minor changes (Figure 19-15).3
Figure 19-15. Long axis of the infraspinatus muscle (arrow). (A) Normal appearance. (B) Contralateral side showing atrophy and increased echogenicity related to a tendinous rupture (arrow).
NERVES
Normal Appearance
Nerves have a fascicular pattern consisting of multiple hypoechoic parallel linear areas (neuronal fascicles) running longitudinally within the nerve, separated by hyperechoic bands that correspond to connective tissue layers (interfascicular epineurium) (Figure 19-16A). On transverse scans, nerves assume a honeycomb-like appearance with hypoechoic rounded areas embedded in a hyperechoic background (Figure 19-16B).4 Systematic scanning in transverse planes is preferred in order to follow nerves contiguously throughout a limb (“lift technique”).11 Nerves are less subject to anisotropy than tendons. Color Doppler can help differentiate hypoechoic nerve fascicles from adjacent hypoechoic small vessels.4
Figure 19-16. Normal median nerve in the forearm. (A) Long axis (arrow) demonstrating multiple hypoechoic parallel linear areas (neuronal fascicles) running longitudinally within the nerve, separated by hyperechoic bands (interfascicular epineurium). (B) Short axis (arrow) demonstrating a honeycomb-like appearance.
Traumatic Lesions
These may be acute or chronic, and derive from nerve stretching or direct nerve contusion or laceration (i.e., penetrating wound).4 When the trauma is significant, focal hypoechoic changes with loss of the fascicular pattern and increased local blood flow are seen in association with a hematoma.4 Transection of a nerve results in a discontinuous nerve that may show swelling at the ends during the acute phase.5
Traumatic neuromas are related to non-neoplastic proliferation of the proximal end of a severed, partially transected, or injured nerve. They may arise 1-12 months after the trauma and are variable in size. Ultrasound demonstrates either a fusiform mass or enlargement with an entering and exiting nerve, or an entering nerve ending in a bulbous shape. The neuroma appears homogeneously hypoechoic, sometimes with small hyperechoic internal bands. It does not contain any Doppler signal (Figure 19-17).21
Figure 19-17. Injured radial nerve. Injured radial nerve in the forearm presenting a fusiform, homogeneously hypoechoic mass corresponding to a posttraumatic neuroma (*). Note the proximal normal nerve (arrows).
Entrapment Syndromes
In entrapment syndromes, neuropathy is related to extrinsic compression. It is more common at anatomic sites where the nerve courses through nonextensible osteofibrous tunnels. Imaging is used to confirm or support a clinical diagnosis of nerve entrapment and identify the abnormality causing the entrapment, such a mass, abnormal muscle, fibrous band, or bone deformity.4,5,22 Typical findings include a deformation of the nerve at the site of compression, swelling of the nerve immediately proximally, and possible neuroma formation or distal nerve atrophy.5 The most frequent entrapment neuropathy is carpal tunnel syndrome. The classic diagnostic triad on ultrasound includes palmar bowing of the flexor retinaculum (<2 mm beyond a line drawn between the hamate–pisiform and the trapezium–scaphoid), distal flattening of the median nerve, and proximal enlargement of the nerve (Figure 19-18A).18,22,23 This enlargement is demonstrated when the cross-sectional area of the nerve in the carpal tunnel exceeds 12 mm2 (Figure 19-18B), or when the difference between the cross-sectional areas of the nerve in the carpal tunnel and at the level of the pronator quadratus muscle exceeds 2 mm2.22,23
Figure 19-18. Abnormal median nerve in a patient with carpal tunnel syndrome. (A) Long axis demonstrating a distal flattening at the site of the reticulum (arrow) and a swelling immediately proximally (*). (B) Short axis demonstrating the nerve’s enlargement, characterized by a cross-sectional area of 15 mm2 (normal <12 mm2).
Morton neuroma can also be considered to be a consequence of an entrapment syndrome. It is a non-neoplastic fusiform enlargement of the plantar digital nerve, with perineural fibrosis, local vascular proliferation, edema of the endoneurium, and axonal degeneration. The plantar digital nerve in the third intermetatarsal space is most commonly affected, although involvement of the second intermetatarsal space is not uncommon. Pain radiating from the mid-foot to the toes is the main symptom. With ultrasound, the neuroma appears as a hypoechoic intermetatarsal mass (Figure 19-19A). Identification of the presumed plantar digital nerve in continuity with the mass improves diagnostic confidence (Figure 19-19B). No internal vascularization is usually detected at duplex study. The maneuver used for the clinical Mulder sign (palpable click when pressure is applied to the sole of the foot and the metatarsals are subsequently squeezed together) is performed to improve the depiction of small Morton neuromas.21
Figure 19-19. Morton neuroma in the third intermetatarsal space. (A) Transverse appearance demonstrating a well-defined hypoechoic mass (*) between the third and fourth metatarsal heads (arrows). (B) Longitudinal appearance (*) demonstrating the neuroma’s continuity with the corresponding plantar digital nerve (arrow).
Nerve Instability
This is mainly observed at the elbow. The cubital tunnel is an osteofibrous tunnel formed by a groove between the olecranon and the medial epicondyle and bridged by the Osborn retinaculum. Dynamic scanning during full elbow flexion can allow continual depiction of the intermittent dislocation of the ulnar nerve over the medial epicondyle if the retinaculum is loose or absent. This abnormal nerve translation has been reported in 16–20% of asymptomatic individuals, but is reported to be a risk factor for dynamic ulnar neuropathy. The nerve may be enlarged and hypoechoic.4,22
Nerve Tumors
The group of peripheral nerve sheath tumors (PNST) includes benign (schwannoma and neurofibroma) and malignant (MPNST) tumors. Detection of a soft tissue mass associated with neurological symptoms and muscle atrophy in a typical nerve distribution is the main clinical feature. Sonographically, nerve tumors usually present as a hypoechoic fusiform mass, with well-defined margins, oriented longitudinally along the nerve axis, revealing tapered ends that are in continuity with the nerve of origin (Figure 19-20). Neurofibromas develop as centrally located masses, whereas schwannomas tend to grow eccentrically to the nerve; however, this parameter seems to be more reliable when discrimination between these lesions is made in larger nerves.4,21
Figure 19-20. Schwannoma. Schwannoma appearing as a hypoechoic fusiform mass (*), with well-defined margins, oriented longitudinally along the nerve axis (arrows).
Schwannomas are much more frequently associated with central necrosis, cyst formation, and calcification than are neurofibromas.21 However, these phenomena occur especially in long-standing schwannomas. Unfortunately, ultrasound is not able to provide a confident differentiation between benign and malignant neurogenic masses. The main signs of malignancy are large tumor size (<5 cm) and a heterogeneous structure with central necrosis.4,21
Abnormalities of the muscle supplied by the nerve (atrophy, fatty infiltration) can be demonstrated with ultrasound, whatever the nature of the nerve lesion.
JOINTS
Ultrasound can easily explore many joints, including those that are difficult to examine clinically (i.e., hip and shoulder). However, its clinical value largely depends on the anatomy of the joints and on the size of the acoustic window. Bone profile and cartilage are the main landmarks in the assessment of joints.10
Joint Effusion
Ultrasound can reliably identify and quantify intra-articular effusions. However, it cannot always differentiate accurately between inflammatory, infectious, microcrystal, and bloody effusions (Figure 19-21). Aspiration of joint fluid, which is more successful with ultrasound guidance, remains the gold standard.18,24 Cartilaginous or osseous loose bodies are sometimes demonstrated in the joint fluid, mainly in degenerative conditions.
Figure 19-21. Fracture and hemarthrosis of the elbow. Posttraumatic hemorrhagic effusion in the elbow due to a fracture (longitudinal section). Note the accumulation of fluid in the olecranon fossa (arrows), with fluid–fluid levels (hemarthrosis*). Notice the insertion of the triceps (TR) onto the olecranon (OL).
Synovitis
In normal states, the synovial membrane is too thin to be clearly discriminated with ultrasound. It does not contain any color or power Doppler signals.1,11 In contrast, synovitis (synovial inflammation) is associated with synovial thickening and hyperemia on color Doppler, at least in the acute and subacute stages (Figure 19-22). This assessment is especially useful in rheumatoid arthritis, particularly in two circumstances:
Figure 19-22. Synovitis and synovial thickening in rheumatoid arthritis of the wrist. Longitudinal aspect of the dorsal portion of the wrist in a patient with rheumatoid arthritis, showing the synovial thickening characteristic of synovitis (arrow). R = radius, L = lunate, C = capitate.
1. At the early stage of the disease, ultrasound demonstrates more synovitis than the clinical examination.18,25,26,27 The demonstration of synovitis of the wrist, metacarpophalangeal (MCP), and metatarsophalangeal (MTP) joints is particularly useful for the early diagnosis of rheumatoid arthritis.
2. In assessing the effectiveness of treatment, there is no development or worsening of joint destruction when there is no synovitis of the joint.27–29
Marginal Bone Erosions
The depiction of bone erosions in joints is a recent indication of ultrasound. Indeed, joint erosions on radiographs represent a key diagnostic criterion and a measure of outcome in rheumatoid arthritis. Recent studies suggest that, at least in some joints, ultrasound can detect more erosions than radiographs (but less than MR imaging).10,26–28,30
In clinical practice, the ultrasound depiction of marginal bone erosions at the ulnar styloid process, at the MCP joints or at the 5th MTP joints, in association with synovitis, strongly supports the diagnosis of rheumatoid arthritis.10,26,27 These erosions appear as well-defined breaks, oval or rounded, of the articular surface, with an irregular floor (Figure 19-23). They must be visualized in two orthogonal planes.1,26,27
Figure 19-23. Erosion of metacarpal head in rheumatoid arthritis. Metacarpophalangeal joint in a rheumatoid arthritis patient demonstrating bone erosions of the metacarpal head (arrow). MTC = metacarpus, P = phalanx.
Hyaline Cartilage
Normal hyaline cartilage is hypoechoic, with well-marked margins (Figure 19-24A).1,18 Degenerative changes of the cartilage (thinning, ulcerations, increased echogenicity), erosions (focal or extensive defects), chondrocalcinosis (a hyperechoic area in the middle-third of the cartilage) (Figure 19-24B), or cartilaginous urate deposits (a hyperechoic line along the cartilaginous surface) can be demonstrated with ultrasound.1,11 However, one of the major limitations of the technique is the incomplete evaluation of the cartilage surface. This is due to the limited capacity of ultrasound for the study of the intra-articular space and limits the usefulness of this technique in this indication.11,18
Figure 19-24. Trochlear cartilage. (A) Normal hypoechoic trochlear hyaline cartilage, with well-marked margins (arrows) covering the bone surface (hyperechoic adjacent line). (B) Chondrocalcinosis, with hyperechoic areas in the middle-third of the trochlear cartilage (calcifications, arrows).
BONES
Normal Appearance
Ultrasound has intrinsic limitations in the assessment of bone, as the ultrasound waves do not go through the cortical bone. The bone surface is represented by a regular hyperechoic line topping an acoustic shadow.1,11,31 Therefore, this technique can only be used for the assessment of the outer surface of bony structures. Its main indication is the depiction of minor fractures that cannot be demonstrated or asserted on radiographs.31
Fractures
They appear as a focal break or irregularity of the hyperechoic line, usually in association with an adjacent subperiosteal hematoma (Figure 19-25), soft tissue infiltration, or joint fluid (hemarthrosis).11,16,18 This technique is particularly useful in children, but open growth plate, canals for perforating vessels, or grooves for nutrient arteries and accessory ossicles must be recognized, as they may appear as interruptions of the continuity of the bone surface, thus mimicking fractures.16,31
Figure 19-25. Proximal radius in a child. (A) Normal aspect. (B) Fracture of the proximal radial diaphysis (arrow). Notice the hypoechoic aspect of the epiphysis, corresponding to nonossified cartilage (*), which is normal in children.
SOFT TISSUE MASSES
The main role of ultrasound is to differentiate liquid masses (synovial cysts such as popliteal cysts, mucoid cysts, bursitis) (Figure 19-26), which are hypo- or anechoic without any internal vascularity, from solid ones. In the latter group, some lesions can be recognized (lipomas, vascular malformations, adenopathies, abscesses, tophus, rheumatoid nodules) (Figure 19-27), but most of them are associated with nonspecific findings and require complementary MR imaging. However, ultrasound can be particularly useful in order to guide the puncture, aspiration, infiltration or biopsy of the mass, and/or follow-up over time.
Figure 19-26. Synovial cyst of the wrist. Perfectly anechoic synovial cyst (*) of the wrist (longitudinal section). R = radius, L = lunate, C = capitate.
Figure 19-27. Inguinal adenopathy. Inguinal adenopathy characterized by hypoechoic well-defined nodules (*).
Finally, ultrasound has emerged as the method of choice for the detection and localization of radiolucent soft tissue foreign bodies such as wooden splinters, toothpicks, plastic, fishbones, glass fragments, and cactus spines. It can also be used to guide their removal.1,32 All these materials are echogenic, but the degree of echogenicity of a specific foreign body is proportional to the differences in acoustic impedance at the interface between it and the surrounding tissue.24 These materials can be identified either by their bright reflections or by the associated acoustic shadowing that occurs when sound transmission is blocked. Metal objects have a specific comet tail artifact that is recognizable as a series of bright echoes trailing the initial bright echo.1 The associated inflammatory response may be seen as an anechoic fluid collection or as a hypoechoic area resembling a halo around the foreign body.1
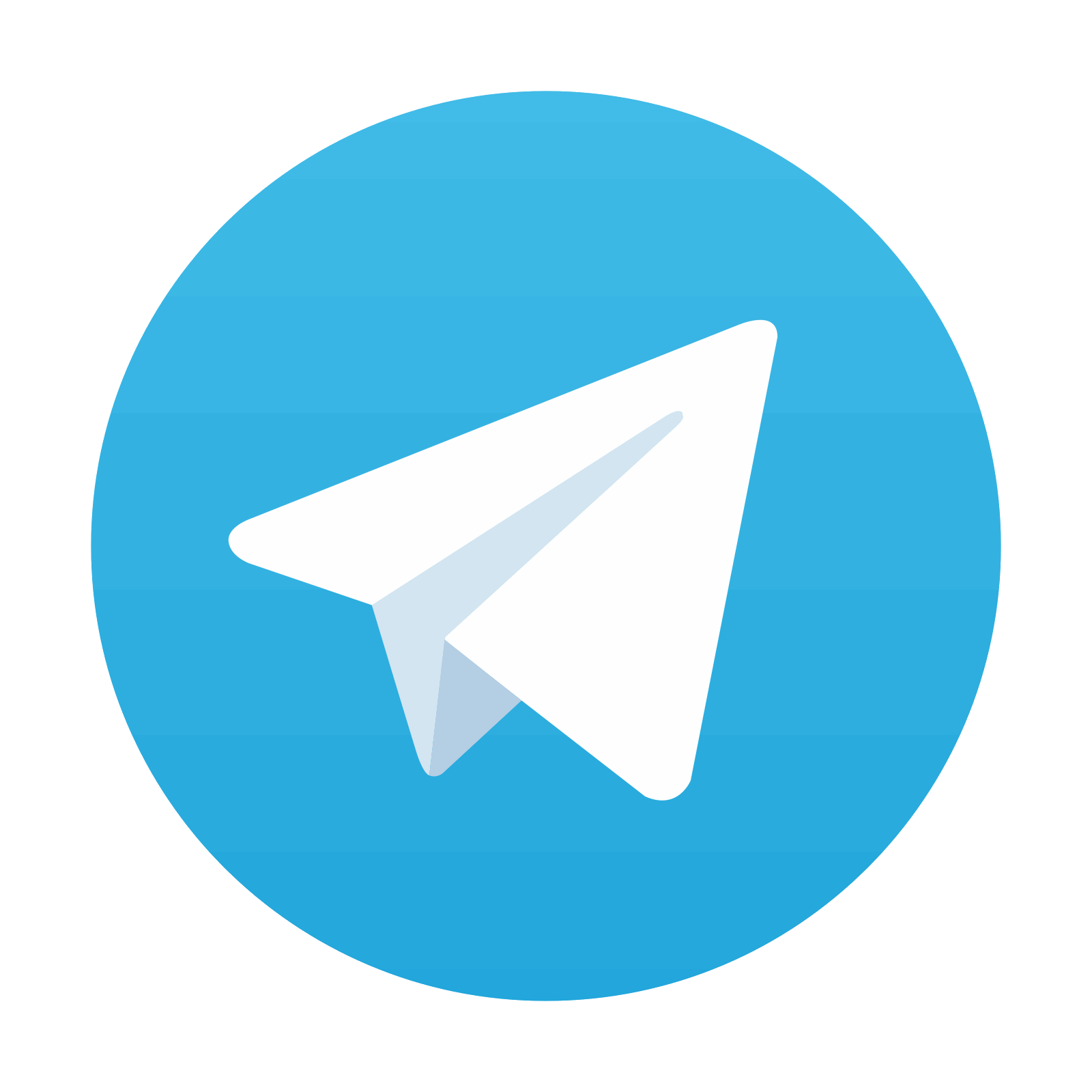
Stay updated, free articles. Join our Telegram channel

Full access? Get Clinical Tree
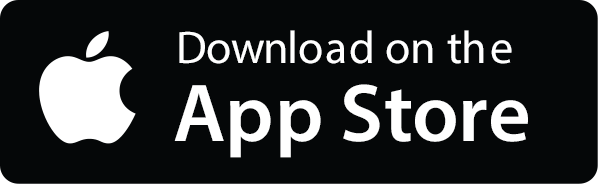
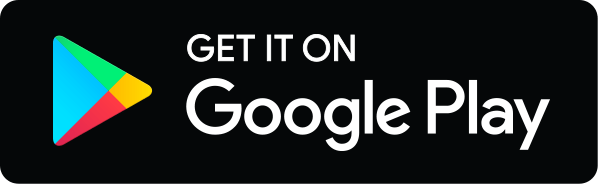