Fig. 1
Conceptual representation of connectivity and dynamics in healthy and challenged brains. Central ellipse represents thalamus, wavy boxes represent specific cortical regions, connecting lines represent both structural and functional connectivity. Green is healthy, red is damaged or dysfunctional, and represents slow or otherwise compromised functional connectivity. Black arrows represent subcortical inputs to thalamus. a Healthy human brain intact structural, functional and temporal connectivity (shown in green) with intact cognition and full consciousness and awareness of external and internal world. b Cognitive disabilities slight alterations in functional (perhaps structural) connectivity and slight partial slowing (indicated in red), otherwise globally intact structural, functional and temporal connectivity, with full consciousness and noticeable but not severe perceptual or cognitive alterations. c Neurology severe partial and persistent slowing and alterations in structural, functional and temporal connectivity (shown in red), mostly related to sensory, motor and cognitive brain areas (TBI patients are excluded here and will be shown in detail in Fig. 2); affecting single or multiple brain networks (probably via inhibition or de-afferentation at thalamic level on TC networks, with additional alterations at CC level). Ranging from intact to altered consciousness depending on pathology, with various and often severe cognitive and sensory-motor alterations. d Psychiatry severe partial and persistent slowing and alterations in structural, functional and temporal connectivity (shown in red), mostly related to limbic and frontal brain areas; affecting single or multiple brain networks (probably via inhibition at thalamic level on TC networks, with additional alterations at CC level). Ranging from intact to distorted consciousness or/and altered emotions depending on pathology, with various and often severe cognitive and sensory-motor alterations and distortions
Local alpha oscillations are thought to reflect cortical inhibition (Klimesch et al. 2007) and/or idling (Pfurtscheller et al. 1996), as local alpha power reductions have been observed following stimulus presentation in various sensory modalities (Klimesch et al. 2007) and during cognitive tasks such as selective attention (Snyder and Foxe 2010; Banerjee et al. 2011). Local desynchronization of alpha rhythms over primary cortex is also associated with active motor control (Pfurtscheller and Neuper 1994) and perceptual processing (Hanslmayer et al. 2011). Such results indicate that alpha oscillations reflect fundamental mechanisms of cortical idling and inhibition that directs information flow within brain networks across diverse contexts (Jensen and Mazaheri 2010). It has also been reported, however, that increases in alpha synchronization among cortical regions may be related to the establishment of long-range networks and further relating to memory retention (Doesburg et al. 2010, 2011a; Palva et al. 2010).
Local increases in gamma-band activity have been linked to active processing within cortex across numerous contexts, supporting the view that they play a critical role in cortical sensory and cognitive processing (Ribary 2005; Fries 2009), including attention and memory (Jensen et al. 2007). Sensory stimulation produces gamma activation (Pantev et al. 1991), as does sensory perception, object recognition and short-term memory retention (Fell et al. 2002; Supp et al. 2007; Tallon-Baudry et al. 1998). Processes that have been reported to reduce local alpha activity are also associated with increased local gamma activation (Fries et al. 2001; Doesburg et al. 2008). As alpha-desynchronization occurs across large areas of cortex relative to the more spatially complex coincident increases in gamma power (Jerbi et al. 2009), transitions of local population dynamics from alpha toward a gamma oscillatory state may mediate segregation of neural populations into functional assemblies relevant for required cortical processing and connectivity.
Further, local theta rhythms have been related to task-dependent processing in both hippocampus and neocortex (Kahana et al. 2001). The relationship between cortical theta oscillations and active processing is perhaps best documented in working memory paradigms (Sauseng et al. 2010). Local theta activity has also been implicated in other cognitive processes including long-term memory processes and selective attention (Osipova et al. 2006; Green et al. 2011). In particular, the coupling of gamma to theta frequency bands has been reported in a variety of cognitive and perceptual processes based on noninvasive recordings (Sauseng et al. 2008; Doesburg et al. 2009, 2012; Griesmayr et al. 2010).
Given the fact that all these oscillations indicate specific individual signatures within the same sensory or cognitive contexts, it is likely that task-dependent activation within a cortical region involves quantifiable cross-frequency relations and connectivity across the entire brain space supported by TC network dynamics, relating to cognition and consciousness (Doesburg, Ward and Ribary, in prepartaion). More discoveries and reports specifically on cortical oscillatory synchronization and connectivity underlying sensory and cognitive functions in the human brain will be further discussed by several other authors in various other chapters of this book.
3 Persistent Partial Slowing and Altered Functional Network Dynamics in Neurology and Psychiatry
Llinás, Jeanmonod and Ribary (Llinás et al. 1999) suggested that a slowing of spontaneous oscillations and alteration of the functional connectivity dynamics within thalamo-cortical systems could contribute to a better understanding of the brain dysfunctions underlying various pathological behavioral symptoms. While general slowing in clinical populations is well known, earlier MEG results, combined with findings based on electrical recordings from human thalamus (Jeanmonod et al. 1996, 2001; Sarnthein et al. 2003) and physiological findings on animals (Jahnsen and Llinás 1984; Llinás et al. 2002), indeed indicated that a severe and sustained slowing together with dysrhythmic thalamo-cortical interactions could be related to various positive symptoms observed in a subset of neurological and psychiatric patients (Schnitzler and Gross 2005; Llinás et al. 1999; Volkmann et al. 1996; Llinás et al. 2001; Schulman et al. 2005, 2011; Timmermann et al. 2003; Sarnthein and Jeanmonod 2007, 2008). Compared to control subjects, patients showed increased low frequency theta rhythmicity in conjunction with a widespread and marked increase of power correlation among high and low frequency oscillations, consistent with other reports (John et al. 1988). Such dysrhythmias can be explained by either excess inhibition or disfacilitation on the TC system in those patients, inducing the generation of low threshold calcium spike bursts by thalamic cells as seen in animals (Llinás et al. 2001) and humans (Jeanmonod et al. 1996). The presence of these thalamic bursts then directly relates to thalamic cell hyperpolarization and low frequency oscillation generation within thalamus (Jahnsen and Llinás 1984). This produces a slowing of theta/delta activity as a result of a resonant interaction between thalamus and symptom-specific cortical areas. The etiology of many of these neuro-psychiatric pathologies which express these core characteristics of thalamocortical dysrhythmia are of course quite different in nature, but what they all have in common is either an increased inhibition at the thalamic level (i.e. in Parkinson’s Disease via pallido-thalamic tract) or a dysfacilitation at the thalamic level (i.e. in amputee patients with neurogenic pain). Such pathological changes in thalamic inhibition or disfacilitation result in such TC slowing, which has been well characterized through animal neurophysiology, and is best observed at the cortical level using MEG or EEG imaging technologies.
Global slowing has been well characterized in the healthy human brain in the context of transition to sleep. Such slowing has also been demonstrated in animal studies, as the brain slows down from resting alpha band frequency (8–12 Hz) to lower frequency theta (6–8 Hz) and then to delta band (2–4 Hz) during deep sleep with a large global coherence across the entire brain (Steriade et al. 1993b). In states of thalamocortical dysrhythmia (TCD) however, partial slowing is persistent with ongoing theta-range (4–8 Hz) thalamic activity, serving as the trigger for cortical dysfunction in which a core region of cortex functions at lowered frequency, surrounded by a region of activation in the normal waking gamma (25–50 Hz) range (Llinás et al. 1999, 2002). In addition, the altered connectivity of the thalamocortical system not only maintains this pathological dynamic, but also causes it to become distributed throughout wide areas of cortex representing a large-scale coupling, which allows such activity to constrain thalamocortical dynamics very efficiently. This strengthens considerably the idea that some of the mild cognitive deficits could arise from varying degrees of a slight dysrhythmia (Llinás et al. 1998b; Ribary 2005).
The challenge for future dynamic brain imaging studies using MEG or EEG technologies is then (i) to precisely localize these persistent slowing TC areas or symptom-specific networks, best observed at the cortical level during “resting state” (ii) to specify the alterations in “resting” connectivity and subsequent induced altered task-specific activations in functional cortical connectivity dynamics across all frequency bands in precise detail, and (iii) to correlate such altered connectivity and dynamics with clinical symptoms (see conceptual figure: Fig. 1c–d).
4 Slight Partial Slowing and Altered Functional Connectivity Dynamics in Cognitive Disabilities
Altered functional connectivity dynamics, as described in neuropsychiatric populations above, also provides a conceptual framework for evaluating slight alterations in resting brain activity in the normal aging brain or in some cognitive disabilities (see Ribary 2005). These alterations are expected to be minimal compared to severe dysrhythmia in neurological or psychiatric conditions (see conceptual figure: Fig. 1b). Recent behavioral and functional brain imaging studies of some cognitive disabilities indeed suggest slight perceptual and cognitive alterations including slowing in the underlying neurophysiological network connectivity, despite an average or even above average IQ in some of these individuals. In children born very preterm (≤32 weeks gestational age), for example, Grunau, Doesburg, Ribary and colleagues have recently shown a significant alteration in synchronization and network connectivity dynamics within the alpha frequency band (Doesburg et al. 2011a). In particular, a slight slowing of resting alpha band activity towards theta (6–8 Hz), has been correlated with selective developmental difficulties in this group (Doesburg et al. 2011b).
An alteration of precise timing of network dynamics and connectivity has also been reported earlier to be associated with altered behavioral patterns, namely with altered perception of sensory input (Ribary et al. 1999; Ribary 2005). Earlier MEG studies suggested a slight dysrhythmia within thalamo-cortical systems in subjects with Language-Based Learning Disabilities (Llinás et al. 1998a; Nagarajan et al. 1999). Functional imaging data by Ribary, Llinás, Tallal, Miller and colleagues have shown that the minimal interstimulus interval required to identify two separate sensory events was altered and delayed in subjects with Language-Based Learning Disabilities (LLD or dyslexia) (Llinás et al. 1998a; Ribary et al. 2000). This relationship is observable independently by either through psychological tests or by functional MEG imaging. Also, the results from these two different measurement techniques were highly correlated, indicating that MEG imaging can be used as an objective measure of normal and slightly altered sensory cognitive experience, including improvements during intervention (Nagarajan et al. 1999).
Gamma-band connectivity abnormalities were further suggested to be one of the neurophysiological correlates of the temporal deficits recorded in Language-Based Learning Disabilities (LLD or dyslexia) (Llinás 1993). This dyschronia is in fact consistent with other functional and temporal brain imaging findings concerning LLD (Tallal et al. 1993; Tallal 2004; Salmelin et al. 1996; Salmelin 2007; Helenius et al. 1999; Simos et al. 2000; Heim et al. 2000; Benasich and Tallal 2002; Gabrieli 2009), including the remediation by cognitive interventions (Tallal et al. 1996; Merzenich et al. 1996). MEG findings further suggested the existence of two different sub-groups of LLD subjects, possibly relating to either a delay or an interruption of gamma band connectivity dynamics (Ribary 2005).
Present research on the diagnosis and treatment of dyslexia has accelerated with an increasing focus on identifying biological substrates and potential early precursors (see Benasich and Fitch 2012). A tighter emphasis on precursors, referred to as “predyslexic” populations, should enable earlier identification of those children at highest risk for dyslexia and provide insight into the etiologies, common pathways, neurobiological correlates, and behavioral phenotypes of language learning disorders. Such identification of populations at highest risk is particularly important, given that current remediation relies exclusively on interventional therapies that are most effective at a young age. Therefore, more comprehensive research on cognitive disabilities using structural and functional brain imaging technologies is necessary for better understanding the alterations in structural and functional connectivity and especially the oscillatory connectivity dynamics across subcortical and cortical brain networks (see conceptual figure: Fig. 1b).
5 Alteration and Loss of Functional Network and Connectivity Dynamics in TBI
Schiff, Ribary and colleagues earlier reported a massive dissociation among TC-CC-CT networks in traumatic brain injury in persistent vegetative patients (PVS) who had suffered severe thalamic injury and were unconscious for several months to several years (Schiff et al. 2002). In a series of studies on these PVS patients, using MRI (magnetic resonance imaging), PET (positron emission tomography) and MEG, they reported massive structural damage within thalamo-cortical systems, combined with a massive shutdown of cortical and sub-cortical brain metabolism, and a massive reduction in functional dynamics indicating abnormal, delayed and incomplete brain activity and gamma band responses (Schiff et al. 1999, 2002; Plum et al. 1998) (see conceptual Fig. 2f). These studies were in accordance with other functional brain imaging studies, demonstrating consistently diffuse and uniformly reduced cerebral metabolic activity (Levy et al. 1987; DeVolder et al. 1990; Tomassino et al. 1995; Rudolf et al. 1999; Laureys et al. 1999), and a selective disappearance of sensory midlatency responses and early-evoked potentials in comatose patients (Pfurtscheller et al. 1983) and during anesthesia (Madler et al. 1991).
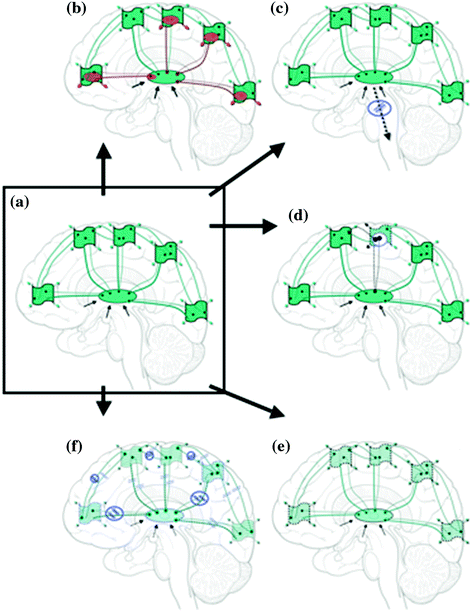
Get Clinical Tree app for offline access
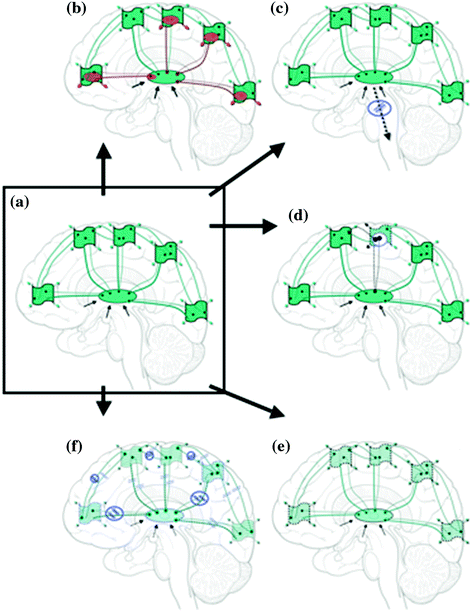
Fig. 2
Effects of traumatic brain injury (TBI). Central ellipse represents thalamus, wavy boxes
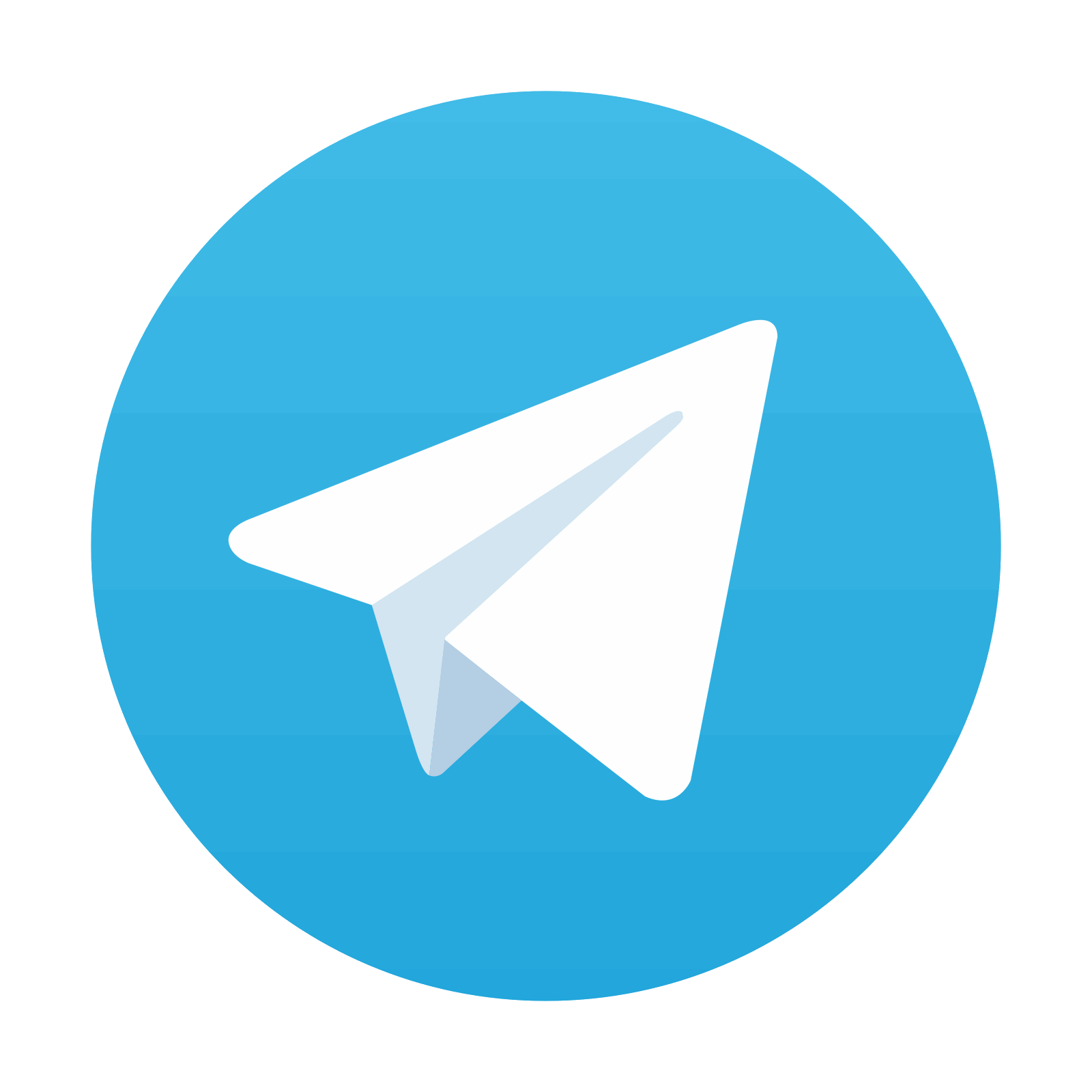
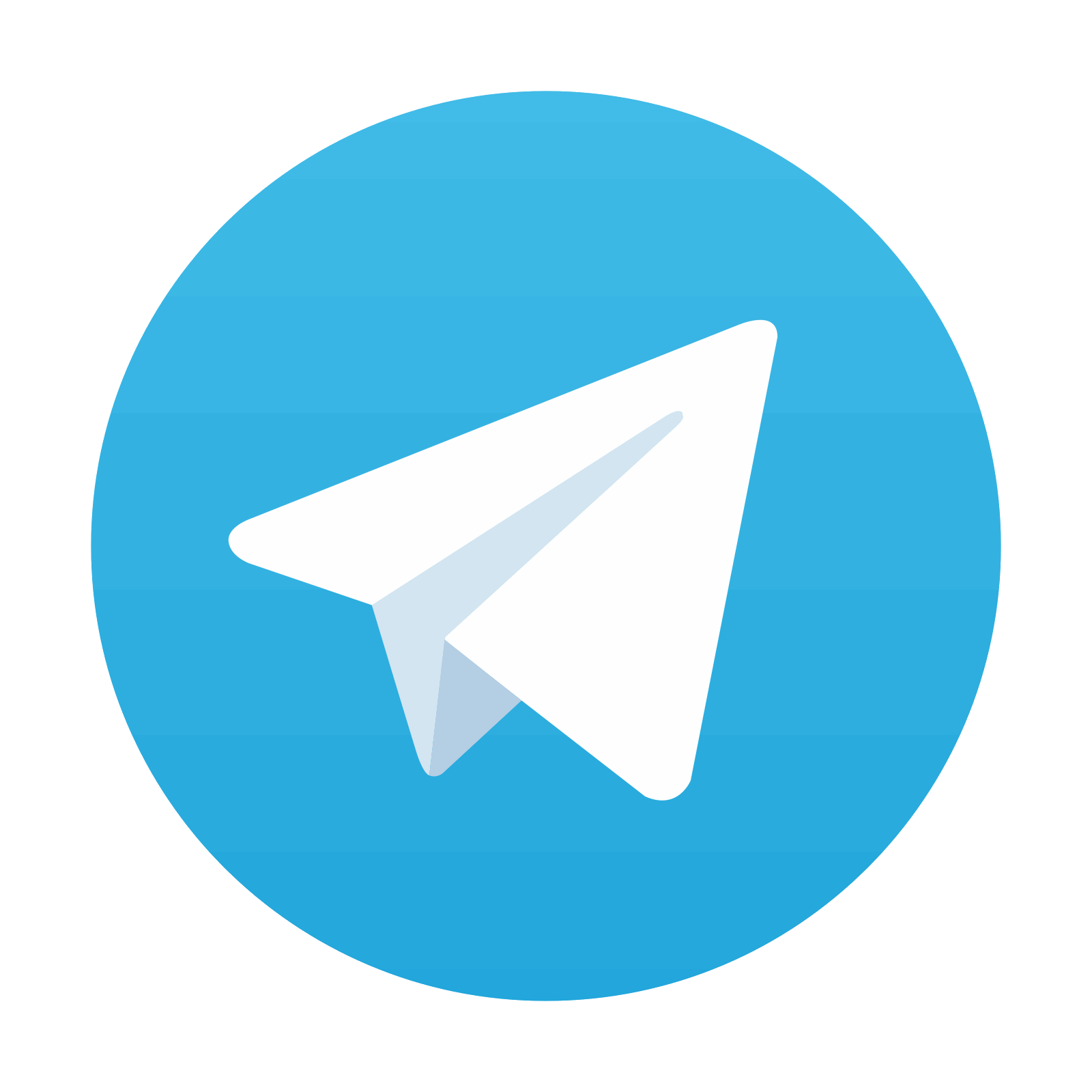
Stay updated, free articles. Join our Telegram channel

Full access? Get Clinical Tree
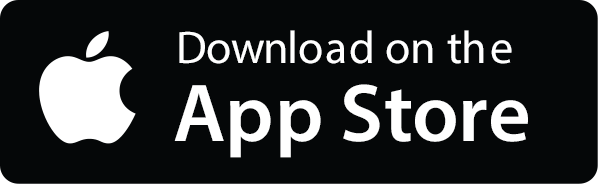
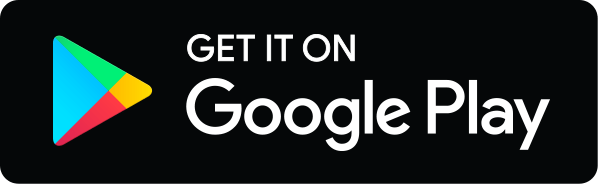
