Conventional MR imaging is, at present, the most important paraclinical modality for assessing the risk of MS in patients with acute demyelinating ON and for monitoring the progression of disease. However, there are limitations of conventional MR in imaging the optic nerve. Newer strategies, MT MR imaging, DT MR imaging, and OCT, show significant promise. Future investigations, including the use of nonconventional MR imaging techniques coupled with OCT and functional measures of anterior visual pathway function, will further assist in the early detection of clinical impairment. Serial analysis will allow for monitoring of disease progression, predict accumulation of disability, and ascertain the effects of candidate neuroprotective therapies.
Multiple sclerosis (MS) is a disease characterized by demyelination and axonal injury, disseminated in time and space. Definitive diagnosis requires the documentation of discrete clinical events, with neurologic symptoms or neuroimaging studies demonstrating involvement of different areas within the central nervous system (CNS). Over time, it has been recognized that a significant degree of discordance exists between radiographic lesions and clinical disability. Therefore, the link between structure (as assessed by conventional imaging techniques) and function is incomplete. Moreover, it is logical to infer from this observation that the progression of MS is, in large part, subclinical, further emphasizing the need for novel means of detecting disease evolution in susceptible individuals.
Despite significant variability in the clinical presentation of MS, vision loss attributable to acute demyelinating optic neuritis (ON) is often the initial manifestation. Similarly, MS is thought to be the most common cause of ON. Unfortunately, an episode of ON is not sufficient to diagnose MS; indeed, some patients will recover and never again develop neurologic symptoms. A particular challenge remains unsolved: how to identify those patients with ON who will subsequently develop MS, and, once identified, how to longitudinally monitor them to ensure clinical stability. Thus far, conventional neuroimaging techniques (including magnetic resonance imaging [MR imaging]) have been used in conjunction with clinical measures to determine the risk of developing MS for patients with ON. As a consequence of these investigations, much has been learned about the pathophysiology, and the natural history, by which these two conditions are associated.
Unique features of the optic nerve, including its frequent involvement early in the course of MS, and the relative ease by which both structure and function can be analyzed, make the anterior visual pathway a compelling model for the evaluation of inflammatory-demyelinating disease elsewhere within the central nervous system (CNS). It has now been established that patients with MS manifest subclinical alterations in optic nerve function; these dynamic changes lend themselves to longitudinal monitoring.
Seeking to capitalize on the accessibility and vulnerability of the optic nerve in MS, various nonconventional imaging techniques have been developed. As with any structural assessment, effective implementation requires validation by reliable and useful clinical outcomes. Through the correlation of these novel imaging strategies with established measures of anterior visual pathway function, advances in the early detection and serial monitoring of demyelinating disease are rapidly emerging. Moreover, the opportunity to assess here-to-fore unseen changes as a result of disease evolution allows for more sensitive analysis of the efficacy of candidate neuroprotective agents.
Specific quantitative imaging techniques relevant to the optic nerve include measurement of optic nerve atrophy, magnetization transfer (MT) MR imaging, diffusion tensor (DT) MR imaging, and optical coherence tomography (OCT).
Optic nerve anatomy
The paired optic nerves, each 50 mm in length, serve to transmit visual information from the retina to the optic chiasm. Each optic nerve, from the globe to the chiasm, is composed of four parts: intraocular (optic nerve head, 1 mm), intraorbital (25 mm), intracanalicular (traversing the optic canal, 9 mm), and intracranial (stretching from the canal to the optic chiasm, 4 to 16 mm). Each is composed of a bundle of nerve fibers that maintain a topical arrangement along their course from the retina through the optic chiasm. The optic nerves are, histologically and phylogenetically, extensions of brain, and are surrounded by cerebrospinal fluid (CSF). These unique characteristics allow the optic nerve to serve as a model for disease processes occurring elsewhere within the CNS, including demyelination and axonal loss.
Optic neuritis: clinical presentation
In the absence of other symptoms of MS, acute demyelinating ON is referred to as one of the clinically isolated syndromes (CIS) suggestive of MS. Young to middle-aged persons (second through fourth decade) are most commonly affected. ON is approximately three times more common in women than in men. Patients present with vision loss that is acute or subacute. In greater than 90% of patients, pain exacerbated by eye movements is reported. The degree of vision loss is variable, with median visual acuity of 20/60 at presentation. Most patients improve within several weeks of onset. The clinical examination should disclose the presence of an afferent pupillary defect. In two thirds of the patients, optic nerve appearance will be normal. Almost any visual field defect can occur, although central scotoma or diffuse field loss is the most common. If visual function worsens beyond a 2-week period, or does not improve within 4 weeks, alternate etiologies should be considered. Improvement in acuity can be hastened through the use of intravenous corticosteroids. Other aspects of vision, including color perception, low-contrast letter acuity, and depth perception, often remain deficient. Uhthoff’s phenomenon (a transient worsening of vision following exercise or exposure to heat) may occur.
Optic neuritis: clinical presentation
In the absence of other symptoms of MS, acute demyelinating ON is referred to as one of the clinically isolated syndromes (CIS) suggestive of MS. Young to middle-aged persons (second through fourth decade) are most commonly affected. ON is approximately three times more common in women than in men. Patients present with vision loss that is acute or subacute. In greater than 90% of patients, pain exacerbated by eye movements is reported. The degree of vision loss is variable, with median visual acuity of 20/60 at presentation. Most patients improve within several weeks of onset. The clinical examination should disclose the presence of an afferent pupillary defect. In two thirds of the patients, optic nerve appearance will be normal. Almost any visual field defect can occur, although central scotoma or diffuse field loss is the most common. If visual function worsens beyond a 2-week period, or does not improve within 4 weeks, alternate etiologies should be considered. Improvement in acuity can be hastened through the use of intravenous corticosteroids. Other aspects of vision, including color perception, low-contrast letter acuity, and depth perception, often remain deficient. Uhthoff’s phenomenon (a transient worsening of vision following exercise or exposure to heat) may occur.
Conventional MR imaging: lesions of the optic nerve
The diagnosis of acute, monosymptomatic ON is often based on the appropriate clinical history and supportive examination findings, without the need for neuroimaging. However, data from the Optic Neuritis Treatment Trial (ONTT) and the Longitudinal Optic Neuritis Study (LONS) have highlighted the utility of brain MR imaging in predicting the subsequent risk of developing MS. In atypical cases, MR imaging of the orbits should be obtained to exclude an alternate diagnosis, such as a compressive lesion.
In acute monophasic ON, the most effective conventional MR imaging sequences are (1) short tau inversion recovery (STIR), (2) fast spin-echo (FSE) T2-weighted images, and (3) spin-echo T1 pre- and post-gadolinium fat-suppressed sequences. With these techniques, the causative lesion can frequently be identified ( Fig. 1 ). Typical findings include dilation of the optic nerve sheath immediately posterior to the globe on fat-saturated FSE sequences, and optic nerve sheath enhancement (a high-signal ring corresponding to the optic nerve sheath) on gadolinium-enhanced images. Although previously thought rare in patients with acute ON, a study by Hickman and colleagues has suggested that optic nerve sheath dilatation (45%) and optic nerve sheath enhancement (75%) are common findings. However, these findings are nonspecific, and cannot distinguish ON from ischemic or infectious causes of optic neuropathy. Moreover, collective clinical and experimental evidence has repeatedly shown a poor correlation between MS lesions detected by conventional MR imaging, and functional disability. Therefore, the use of MR imaging as an outcome metric for assessing disease progression, and response to disease modifying therapies, is imperfect. It is because of these and other limitations that the development of nonconventional imaging techniques has become necessary.
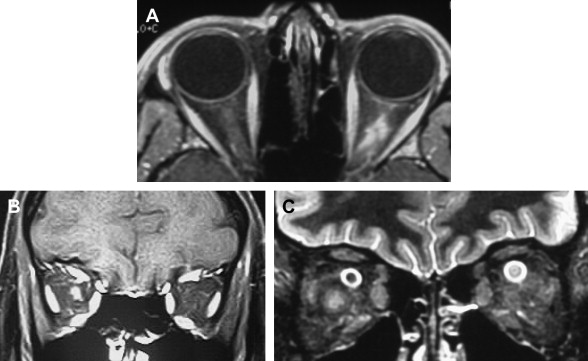
Optic nerve atrophy
While classically considered an inflammatory-demyelinating process, both acute and chronic phases of MS have been shown to result in axonal injury; this is the basis for progressive and permanent neurologic dysfunction as a consequence of demyelinating disease. Following the acute phase of ON (when the inflammatory process results in edema of the optic nerve), ongoing demyelination results in axon loss and atrophy. This process was first documented using conventional optic nerve imaging in patients with ON. With more recent improvements in MR imaging techniques, including fat- and CSF-suppressed sequences, analysis of optic nerve atrophy has been reliably correlated with functional impairment. Hickman and colleagues described a fat-saturated short echo fast fluid-attenuated inversion-recovery (sTE fFLAIR) sequence to overcome the high signal from orbital fat and CSF surrounding the optic nerve. Using this technique, an in-plane resolution of 0.5 mm 2 and 3-mm slice thickness was achieved, allowing for the measurement of optic nerve atrophy following a single episode of ON. Equally important was the observation that the degree of atrophy was related to disease duration, further underscoring the impact of ongoing axonal degeneration as a consequence of demyelination. Progressive atrophy was associated with poor visual recovery and reduced visual evoked potential (VEP) amplitude. In yet another series of 21 patients, mean optic nerve diameter in eyes with acute ON was reduced compared with contralateral eyes and healthy controls. These data suggest that measurement of optic nerve atrophy may be a useful strategy for determining the stage of ON, and for following axonal loss over time.
Magnetization transfer MR imaging
Magnetization transfer MR imaging (MT MR imaging) provides an analysis of the degree of tissue damage, both within and surrounding demyelinating lesions. By measuring macromolecular density, MT MR imaging is superior to conventional MR imaging in its specificity for detecting irreversible demyelination and axonal injury. The studies completed to date suggest that MT MR imaging correlates with functional outcome measures, and may be a useful technique for the longitudinal monitoring of patients with MS.
The principle of MT MR imaging is predicated on differences in the interactions of free and bound protons when exposed to off-resonance irradiation. The magnetization of bound protons becomes saturated; these protons then transfer magnetization to more mobile protons, resulting in reduction of the tissue signal. The difference in signal intensity before and after the pulse allows for calculation of the MT ratio (MTR); a low MTR is indicative of tissue damage resulting from demyelination and axon loss. Conversely, recovery of MTR may reflect remyelination, or resolution of acute inflammation/edema. In a serial imaging study of four patients with MS, decreases in MTR were most prominent at lesion appearance, and during the first 2 months thereafter. Of the 15 lesions documented, MTR then returned to normal in five of them, and eight showed a slower rate of decline over subsequent months. These findings suggest that normalization of MTR is attributable to resolving edema, while progressive declines may be a marker of further demyelination and axonal loss. As an additional confirmatory measure, values obtained by MTR have been correlated with axonal injury and conventional MR lesions through postmortem studies.
Once again, application of this imaging strategy in ON has yielded compelling in vivo data documenting the initial and longitudinal effects of MS. In 39 patients with acute ON, the mean intraorbital MTR measured 30.6 percent units (pu) in optic nerves demonstrating either a high-signal T2 lesion or contrast enhancement, compared with 41.1 pu in healthy controls. In another study, 20 patients analyzed between 3 months and 16 years after a course of unilateral ON were similarly found to have decreased mean MTR in the affected intraorbital optic nerve when compared with the contralateral optic nerve and healthy controls. The reduction in MTR was found to be inversely correlated with VEP latency, suggesting a relationship between decreased MTR and demyelination. Another cohort of patients with prior ON demonstrated a correlation between MTR and visual acuity.
To overcome the limitations of two-dimensional gradient-echo imaging (specifically, the need for manual placement of a region-of-interest [ROI] of 4 to 8 voxels that may exclude data if the optic nerves are swollen, or include partial-volume pixels containing CSF or orbital fat if the optic nerves are atrophic), Hickman and colleagues used a high-resolution gradient echo sequence to prospectively evaluate optic nerve MTR in a cohort of patients with acute unilateral ON. At the onset of symptoms, when vision was most affected, MTR values were unchanged (compared with acute brain lesions, which demonstrate a rapid initial decrease in MTR). This finding was attributed to two factors: in the initial stages following axonal transection, Wallerian degeneration may increase MTR as a result of increased exposure of myelin fragments. In addition, structural features limiting the development of vasogenic edema in the optic nerve may lessen the effect of blood–optic nerve barrier breakdown on MTR. During the period of visual recovery, mean MTR continued to decline until reaching a nadir at approximately 8 months. This finding, the authors suggest, indicates that there are factors involved in visual recovery that are not detected by MTR, and that tissue changes within the optic nerve continue for several months following the initial ON event, and following visual recovery. Finally, lower time-averaged MTRs were associated with worse visual outcomes.
MT MR imaging is emerging as another nonconventional MR strategy to circumvent the barrier of discordance between MR imaging appearance and neurologic dysfunction. Furthermore, MTR can be measured in different segments along the whole of the optic nerve, thus providing information concerning the progressive nature of demyelination and remyelination over time. This particular feature is of potential value as the effects of disease-modifying therapies are evaluated.
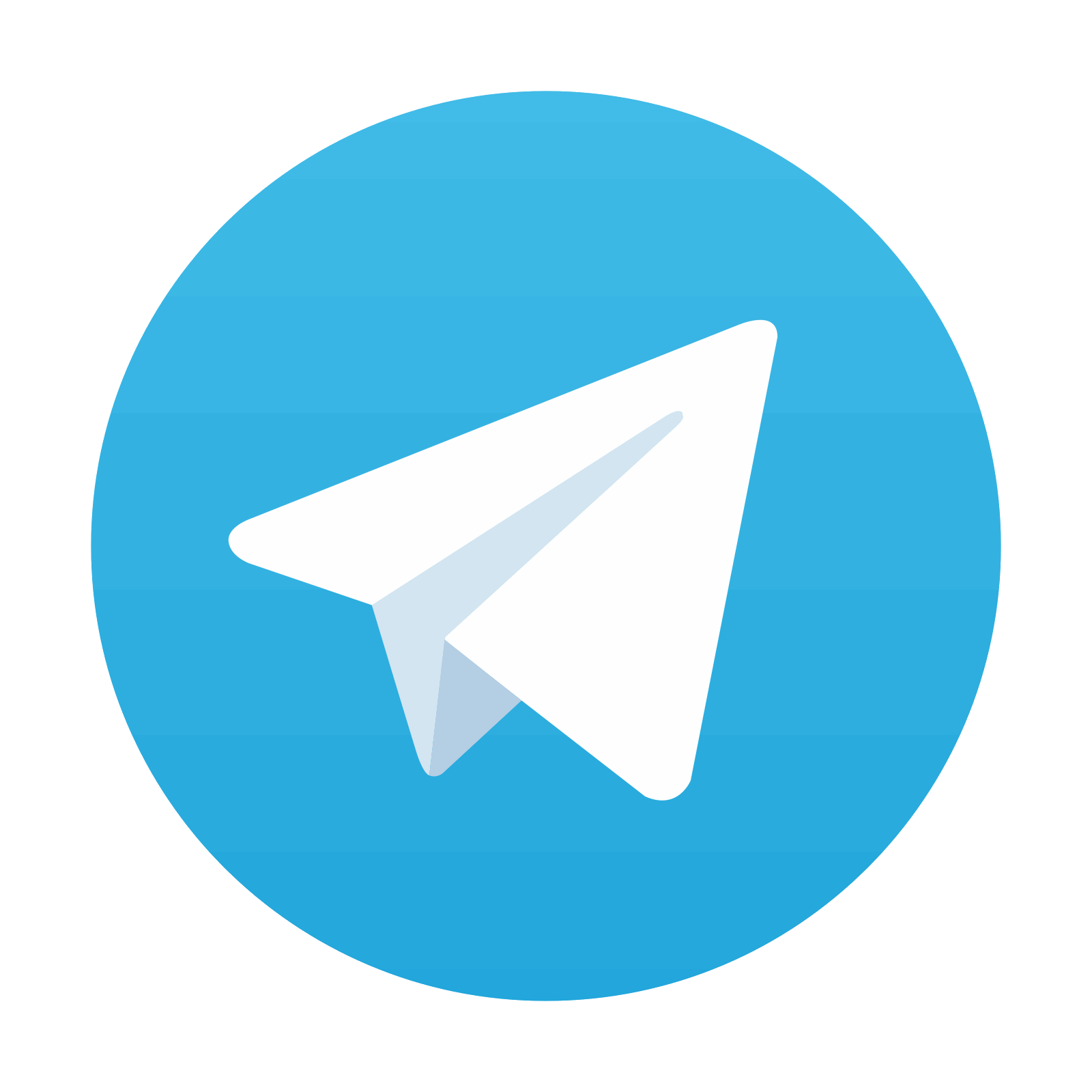
Stay updated, free articles. Join our Telegram channel

Full access? Get Clinical Tree
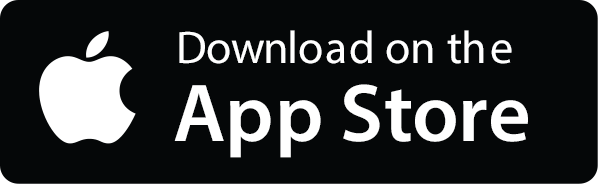
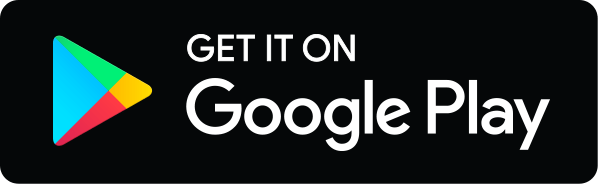