Abstract
Technological advances coupled with a robust evidence base have lead to non-invasive coronary angiography becoming a viable proposition. This chapter reviews CT and MR coronary angiography and the evidence supporting their use for diagnosis and prognosis.
Keywords
Cardiac CT, Calcium scoring, Cardiac MRI, Non-invasive coronary angiography.
7.1
Introduction
Invasive coronary angiography (ICA) is the gold standard for the identification of anatomically obstructive coronary artery disease (CAD). However at many centers, ICA is a limited resource that is expensive and has periprocedural risks (death, cerebrovascular accident, myocardial infarction, and vascular complications). Thus, ICA may not be appropriate for all patients and best reserved for those at highest risk and who likely require coronary revascularization. A noninvasive method that can accurately diagnose and risk stratify patients could function as a gatekeeper for ICA. Technological improvements in computed tomography (CT) and cardiovascular magnetic resonance (CMR) imaging have made non-invasive coronary angiography feasible.
7.2
Coronary artery calcium
Atherosclerosis is a complex pathophysiologic process that begins with endothelial injury. Disruption of the protective endothelial layer increases its permeability and impairs its regulatory system and its ability to respond to stressors. The subsequent deposition of oxidized low-density lipid proteins results in the overexpression of cell adhesion molecules, recruitment of inflammatory cells, accumulation of lipid laden foam cells in the subendothelium, smooth muscle proliferation, and positive remodeling. As part of this process, calcium phosphate hydroxyapatite deposition occurs. Therefore, coronary calcification is a specific marker of coronary atherosclerosis.
Noncontrast enhanced cardiac CT can be used to detect and measure coronary artery calcification (CAC). It is recognized that the composition of coronary atherosclerotic plaque is variable and that calcification is a later process. Therefore, CAC is an indirect measure of total plaque burden and volume. Although noncontrast enhanced CT cannot assess the coronary lumen, there is a relationship between CAC and coronary artery stenosis ( Figure 7.1 ). Patients with high CAC scores have a higher likelihood of having obstructive CAD and major adverse cardiovascular events (MACEs) [ ] ( Figure 7.2 ). However, it is important to recognize that the relationship between CAC and coronary stenosis is not linear ( Figure 7.1 ) [ ].
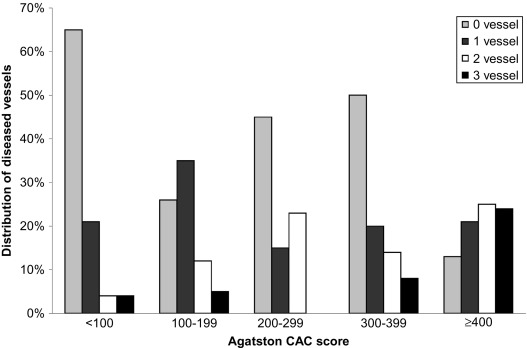
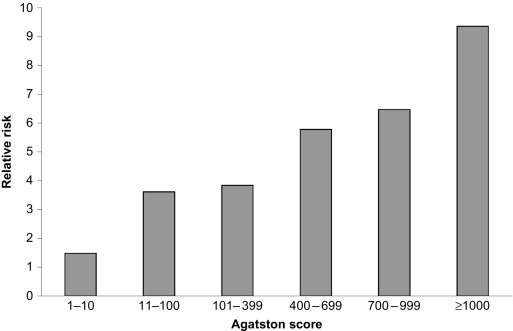
Several methods of quantifying CAC (mass score, calcium volume method, and Agatston score) have been studied [ ]. The Agatston method has been the most frequently used and best validated method for quantifying CAC. Using the Agatston method, calcific coronary plaque is defined as a perivascular lesion ≥ 130 Hounsfield Units (HU) with an area of ≥ 1 mm 2 (three pixels) [ ]. The Agatston score is a unitless score that is calculated as the product of the area of calcium and a multiplication factor representing the maximum attenuation of each lesion ( Table 7.1 ). The Agatston score is considered useful for refining cardiovascular risk of asymptomatic patients and has incremental prognostic value over conventional clinical risk prediction models (such as age, sex, cardiac risk factors) ( Figure 7.3 ).
CT hounsfield unit no. | Weighting factor a |
---|---|
0–129 | 0 |
130–199 | 1 |
200–299 | 2 |
300–399 | 3 |
> 400 | 4 |
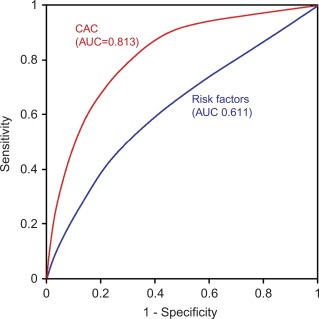
Acknowledging that the correlation between the Agatston score and obstructive CAD is fair, the absence of CAC is associated with a low risk of future adverse events [ ]. In asymptomatic patients, a zero calcium score has a very low prevalence of obstructive CAD (2%) [ , ]. An important distinction is that the data from these studies was derived from patient cohorts referred for coronary angiography, with an inherent higher prevalence of obstructive CAD compared to the group of patients subjected to cardiac CT in clinical practice. Thus, in the asymptomatic population, a zero calcium score effectively rules out obstructive CAD. Currently, according to the AHA/ACCF taskforce guidelines, calcium imaging has a Class IIa recommendation for cardiovascular risk assessment in asymptomatic adults at intermediate risk and a IIb recommendation for low to intermediate risk.
Although a zero calcium score in the asymptomatic population is reassuring, the ability to absolutely rule out obstructive CAD in the symptomatic population is more difficult. Although it may effectively rule out high-risk coronary anatomy (three vessel disease ≥ 70%, two vessel disease involving proximal left anterior descending artery ≥ 70% or left main stenosis ≥ 50%), a zero calcium score cannot be used to exclude CAD as a cause of symptoms [ , ]. In the symptomatic population with a zero calcium score, the prevalence of obstructive CAD (diameter stenosis ≥ 50% by CT or invasive angiography) is still 7%. It is reassuring that in clinical practice, the proportion of patients with obstructive disease is very low (0–2%) after a negative calcium scan [ , ]. The CONFIRM registry reported that less than 1% of patients with a negative calcium scan eventually required revascularization ( Figure 7.5 ) [ ].
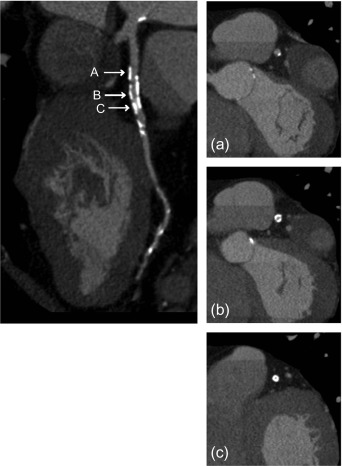
7.3
CT coronary angiography
With the arrival of 64-slice CT scanners came significant advances in both temporal and spatial resolution, which led to the feasibility and the rapid adoption of CT coronary angiography (CTCA) for the assessment of CAD.
Luminal stenosis has been traditionally used as the measure of obstructive CAD severity. Early studies suggested that a threshold of 50% was associated with an abnormal coronary blood flow reserve and 80% threshold was associated with decreased resting coronary flow [ , ]. The Fractional Flow Reserve Vs Angiography in Multi-vessel Evaluation (FAME) trial subsequently showed that the relationship between anatomy and its hemodynamic consequences can be unpredictable [ ] ( Figure 7.6 ). The importance of calcification affecting Cardiac CT interpretation is shown in Figures 7.4 and 7.5 . The increasing extent makes interpretation difficult. Patients with mild lesions (< 50%) remain at risk of cardiovascular events [ ], as studies have reported that > 66% of infarct-related lesions arise from sites with subclinical atherosclerosis [ ].
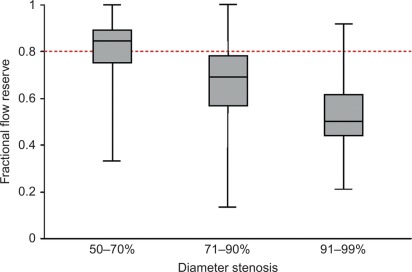
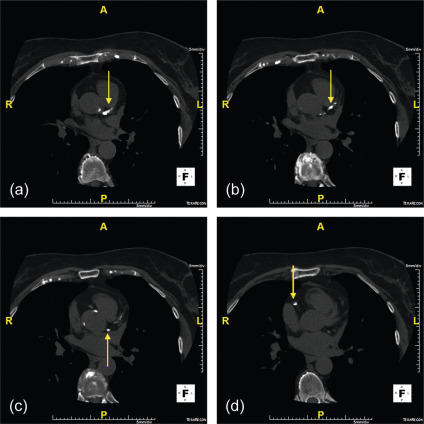
7.3.1
Accuracy
Early single-center studies demonstrated that CTCA has a high sensitivity and specificity for the detection of coronary stenosis ≥ 50% [ ]. Recent meta-analyses have confirmed that the sensitivity (91–99%) and specificity (74–96%) of CTCA is very good [ ]. Not unexpectedly, the diagnostic performance improved as technology evolved with a concomitant reduction in the number of nonassessable segments [ ]. For 64-slice CTCA, a per-segment sensitivity, specificity, positive predictive value and negative predictive value of 86%, 96%, 83%, and 97%, respectively, and 98%, 91%, 93%, and 96%, respectively, has been reported on a per-patient basis [ ]. It is anticipated that the operating characteristics of CTCA will improve in parallel with advancements in software (iterative reconstruction) and hardware (gantry rotation speed, detectors, dual energy acquisition).
When interpreting accuracy studies, one has to be mindful that early single-center studies were subject to multiple biases, such as referral, population, verification, and publication biases. To address this, several multicenter trials have examined the diagnostic accuracy of CTCA compared to ICA ( Table 7.2 ). Although their results confirm those found in single-center studies, these studies were still subject to a population bias since study patients were awaiting ICA [ ].
N | CAD (%) | Sensitivity (%) | Specificity (%) | PPV (%) | NPV (%) | |
---|---|---|---|---|---|---|
ACCURACY [ ] | 230 | 25 | 95 | 83 | 64 | 99 |
CORE 64 [ ] | 291 | 56 | 85 | 90 | 91 | 83 |
Meijboom et al. [ ] | 360 | 68 | 99 | 64 | 86 | 97 |
OMCAS [ ] | 117 | 59 | 89 | 99 | 99 | 87 |
The “Coronary Artery Evaluation Using 64-Row multidetector computed tomography angiography” (CORE 64) trial studied 291 patients with and without a history of CAD, but excluded patients with Agatston score ≥ 600 [ ]. This vendor specific study had lower sensitivity (85%) and negative predictive value (83%) than previous single center studies ( Table 7.2 ).
The “Assessment by Coronary Computer Tomography Individuals UndeRgoing InvAsive Coronary AngiographY” (ACCURACY) was a prospective multicenter trial that examined 230 patients without a prior history of CAD with stable anginal symptoms [ ]. In a population with a low (25%) prevalence of CAD, the core lab evaluated all consecutive patients irrespective of CAC, body size, or heart rate. The sensitivity, specificity, positive predictive, and negative predictive values of the ACCURACY trial were 94%, 83%, 48%, and 99%, respectively, with an area under the curve (AUC) of 0.95.
The largest study (360 patients) was performed by Meijboom et al., and included patients with both acute and stable chest pain. This multicenter and multivendor study showed that CTCA had very high sensitivity (99%) and negative predictive value (97%) in a population with a CAD prevalence of 68% [ ]. The “Ontario Multicenter CT Angiography Study” (OMCAS) was a field evaluation of CTCA accuracy in patients awaiting ICA [ ]. In this study, CTCA interpretation was not performed by a core lab and was left to local centers. The results of this study are thought to reflect accuracy if CTCA were to be widely adopted into clinical practice. Not unexpectedly, there were significant variations in operating characteristics across the different centers, which may be related to patient pretest probability or local experience. Most importantly, the results support the need for identifying appropriate indications that exploit the strengths of CTCA, as well as training guidelines for both technologists and imagers.
The accuracy and applicability of CTCA will grow, as limitations relating to heart rate, arrhythmia, and calcification are overcome.
7.3.2
Plaque characterization
Although intravascular ultrasound (IVUS) is the clinically accepted tool for imaging the arterial lumen and wall, CTCA has the potential ability to visualize and characterize coronary plaque [ ]. The potential importance of identifying vulnerable plaque is underlied by pathology studies that demonstrated that plaque rupture is the most common (60%) cause of acute coronary syndrome (ACS), with plaque erosion and calcified nodules making up the remainder [ , ]. Early studies showed that CT has the ability to distinguish noncalcified, mixed, and calcified plaques [ ], and automated CTCA software have been validated against IVUS and virtual histology IVUS [ ]. The accuracy of CTCA (using IVUS as the gold standard) showed that it has a high sensitivity and specificity (90% and 92%, respectively, with an area under the curve of 0.94 to detect coronary plaque [ ].
Known features of vulnerable plaque, confirmed by histology, IVUS, and CT, are outward vessel remodeling, a large necrotic core (> 25% of plaque volume), vasa vasorum in the plaque, fibrous cap < 65 microns thickness, macrophage infiltration of the fibrous cap, and elevated matrix metalloproteinase expression [ ].
Since the majority of plaque ruptures in ACS occur in coronary plaques that do not cause luminal stenosis, routine stress testing cannot identify vulnerable plaque [ ]. CTCA can visualize and differentiate several plaque components, which have been associated with future adverse cardiac events [ ]. Motoyama et al., comparing patients with stable CAD and patients with ACS, showed that culprit lesions were more likely to occur in plaques with spotty calcification, outward vessel remodeling (remodeling index > 1.1), and presence of low attenuation plaque (< 30 HU) ( Figure 7.8 ). Patients with evidence of outward vessel remodeling and low attenuation plaque had a greater probability (22.2%) of experiencing ACS at two years [ ], while others have shown that ring-like plaque represents thin cap fibrous atheroma [ ]. The pathologic basis of ring-like plaque (the napkin ring sign) is not fully known but could represent revascularization with intraplaque hemorrhage, deep microcalcification, liponecrotic core, or completed rupture with surrounding contrast agent [ ].
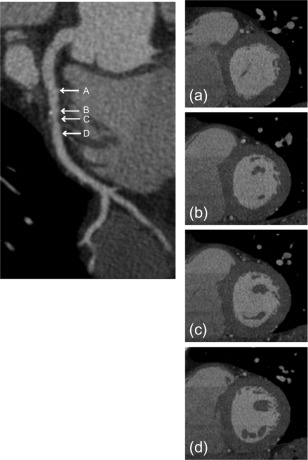
Thin cap fibrous atheroma are predominantly found in the proximal segments of the coronary arteries [ ] with LAD predominance. In a study of 4870 CTCA patients, 474 (65%) of all lesions were subclinical and nonobstructive, with 7% exhibiting high risk features of low attenuation with outward remodeling [ ].
Although CAC has been used as indirect measure of total atherosclerotic burden, it may not accurately identify individual plaque at greatest risk of rupture [ , ]. Since noncalcified plaque appears to be more commonly associated with ACS, noncontrast enhanced CT cannot identify noncalcific plaque with the highest risk features. Similarly, noncontrast enhanced CT may not reliably identify spotty calcification due to the partial volume effect when reconstructing using 2.5 and 3 mm slice thickness. Morita et al. studied 1019 patients with zero calcium score and showed that 4.6% of patients had plaque with ≥ 2 high-risk CT features [ ]. The “Coronary CT Angiography EvaluatioN for Clinical Outcomes: An InteRnational Multicenter” (CONFIRM) registry of 23,854 patients without documented CAD, showed that all-cause mortality was associated with nonobstructive plaque [ ]. Although coronary obstruction remains the most predictive CTCA feature, several studies have demonstrated that the prognostic value of nonobstructive plaque is incremental to clinical risk factors, CAD severity and LVEF [ ].
Although the Framingham risk score (FRS) and the atherosclerotic burden on CT both predict cardiovascular outcome, Pen et al. observed in a large cohort of patients who underwent CTCA that the correlation between FRS and plaque burden was only modest ( r = 0.48) [ , , , ]. A significant proportion of low and intermediate FRS patients had evidence of subclinical coronary atherosclerosis and a small percentage of patients with high FRS had no visible atherosclerosis [ ]. These findings demonstrate the limitations of population-generated clinical risk prediction models and the potential value of imaging to further refine individual patient risk and develop personalized treatment strategies.
While the data supporting CT features that identify plaque at risk of future rupture is quite promising, the ability to detect plaque erosions (which account for approximately 30% of ACS cases) is limited [ ]. Additionally, CT alone cannot be used to detect inflammation, which has been associated with plaque rupture. Currently, there is a paucity of data that links high-risk plaque features with adverse outcomes, and therefore routine plaque screening with CTCA cannot be advocated.
7.3.3
Prognosis by CTCA
The first large prognostic study evaluating 16-slice CTCA in 1127 patients with suspected CAD with a mean follow-up of 15 months [ ] showed that the presence of obstructive CAD, number of vessels with obstructive CAD, plaque distribution, and plaque burden provided independent and incremental value in predicting all-cause mortality. The prognostic value of CTCA in symptomatic patients was independent of age, gender, conventional risk factors, and coronary calcium.
The large multinational, multicenter CONFIRM registry has validated many of the observations made by single centers. The absence of disease on CTCA confers an excellent prognosis with an annualized death rate of 0.6% [ ]. It appears that this excellent prognosis is sustained over time; Ostrom et al. followed patients for over seven years, and the event rate remained very low (0.3%) [ ].
The CONFIRM registry evaluated the incremental contribution of CTCA-defined CAD compared with CACS and conventional risk factors in asymptomatic patients [ ], which showed no improvement in risk stratification observed from information provided beyond CACS. Therefore, CTCA is currently not recommended in the general asymptomatic population.
7.3.4
Percutaneous coronary intervention
With the increasing prevalence of percutaneous coronary intervention (PCI), there is commonly a need to re-evaluate symptomatic revascularized patients. The utility of CTCA in post-PCI patients has been evaluated using EBCT, but without the ability to directly assess the stent lumen. This necessitated the assessment of luminal contrast enhancement distal to the stent as an indirect marker of stent patency. However, the accuracy of this method is unreliable in the presence of retrograde filling by collaterals. The absence of contrast distal to a stent is specific for flow limiting disease but is unlikely to be sensitive and cannot be used to exclude in-stent restenosis.
Factors that have limited stent evaluability include stent composition, luminal size, strut size, and high attenuation seen with overlapping stents. Artifacts, such as partial volume effect and beam hardening artifact, make stent struts appear larger and consequently limit the visibility of the stent lumen. Maintz studied the accessibility of stent lumen and demonstrated that exvivo , in the absence of cardiac motion or patient attenuation, only 50–59% of the lumen was accessible [ , ].
Stent evaluability may be improved by using a sharper kernel image reconstruction by reducing blooming artifact, but it is offset with an increase in image noise. There have been recent improvements in CTCA operating characteristics in post-PCI patients with a reduction in the frequency of nonevaluable coronary stents. Meta-analyses have examined the utility of CTCA for the assessment of coronary artery stents. In 1286 PCI patients, CTCA had a sensitivity, specificity, positive predictive value and negative predictive value of 99%, 89%, 93%, and 100%, respectively (per-patient analysis) [ , ]. Using a segment-based analysis, the sensitivity, specificity, positive predictive value, and negative predictive value were 90%, 97%, 76%, and 99%, respectively. While the sensitivity and specificity appear to be very good, these results may not be generalizable to all stents nor all centers. Stent evaluability is related to stent size with a high proportion of stents < 3.0 mm being unevaluable [ ]. Acknowledging the current limitations to stent evaluation, many centers recommend the use of stress imaging rather than CTCA in stented patients [ ]. ACCF/AHA/ASE/ASNC/HFSA/HRS/SCAI/SCCT/SCMR/STS 2013 Multimodality Appropriate Use Criteria for the Detection and Risk Assessment of Stable Ischemic Heart Disease assigns the designation “maybe appropriate” for the evaluation of symptomatic patients with prior revascularization and “maybe appropriate” in asymptomatic patients with left main stent [ ]. In either situation, evaluation of stents with CTCA may be best left to centers of expertise. Although the role of CTCA in the evaluation of patients with bioabsorbable coronary stents has not been well studied, we would anticipate that diagnostic accuracy be similar prior to stent absorption and superior after reabsorption ( Figure 7.9 ).
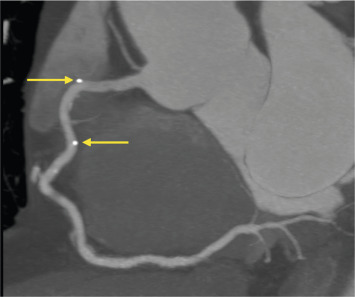
7.3.5
Coronary artery bypass grafts
Imaging coronary artery bypass grafts (CABGs) is feasible due to the fact that grafts are large and less prone to motion artifact ( Figure 7.10 ). Although arterial grafts are smaller than venous grafts and often have surrounding metallic clips, they are usually easily assessed. However, important to the assessment of grafts is the anastomotic site and distal run-off. The anastomotic site can still be affected by cardiac motion and native coronary disease can often be difficult to assess due to diffuse atherosclerosis, coronary calcification, and obstructive CAD. This can limit the utility of CTCA in the CABG population, especially when bypass grafts are occluded and decisions regarding revascularization of the native coronaries are needed. In many cases, ICA is still needed to guide revascularization.
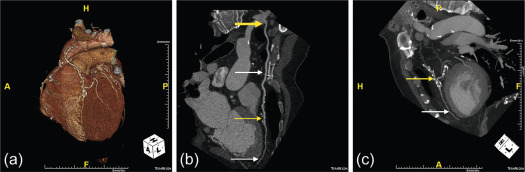
7.3.6
Accuracy
Analyzing 2023 grafts in 723 patients, the accuracy of CTCA in grafts appears to be excellent (sensitivity = 98%, specificity = 97%, positive predictive value = 93%, and negative predictive value = 99%) [ ] and the diagnostic accuracy of CTCA was similar in arterial and venous grafts [ ]. However, many analyses focused on grafts alone and did not include distal run-off, nongrafted vessels, and segments proximal to graft anastomoses. More recent studies have examined the accuracy of CTCA grafts in addition to distal run-offs and native coronaries [ , ]. Nazeri et al. showed that sensitivity and specificity decreased from 98% and 97% (when restricted to analysis of grafts) to 89% and 94% when studying nongrafted and distal runoff segments [ ]. Another study, using a per-segment detection of graft disease, showed sensitivity and specificity of 99% and 96%, respectively, while in the native vessels, sensitivity and specificity were 97% and 86%, respectively [ ]. Acknowledging the potential limitations of CTCA in the CABG population, ACCF/AHA/ASE/ASNC/HFSA/HRS/SCAI/SCCT/SCMR/STS 2013 Multimodality Appropriate Use Criteria for the Detection and Risk Assessment of Stable Ischemic Heart Disease have recommended that evaluation of symptomatic patients is “maybe appropriate” but evaluation of asymptomatic patients “rarely appropriate” [ ].
7.3.7
Prognosis
Acknowledging the potential limitations of diagnostic accuracy in CABG patients, the prognostic value of CTCA in this population has been examined. Using the CONFIRM registry, Small et al. studied 657 CABG patients and demonstrated the incremental value of measures of revascularized and unrevascularized territories, unprotected coronary territories (UCT) and the coronary artery protective score (CAPS) [ ]. The CAPS score appeared to have independent and incremental prognostic value with the ability to appropriately reclassify 27.2% of CABG patients into high and low risk for future all-cause death.
7.3.8
Transluminal arterial gradient and corrected coronary opacification
Transluminal arterial gradient (TAG) and corrected coronary opacification (CCO) have been explored in the efforts to provide functional information that may be incremental to anatomical information. Figure 7.7 shows studies illustrating the discrepancy between the anatomical lesions and their functional significance. TAG uses attenuation samples at 1 mm intervals within the coronary lumen to assess the attenuation change over the coronary artery [ ]. Patients with flow limiting lesions had a more negative slope ( Figure 7.11 ). CCO is a similar method but uses luminal attenuation value normalized to the descending aorta, which is potentially relevant when full coverage of the heart is not possible [ ]. Using this method, patients with abnormal coronary flow (TIMI < 3) had greater CCO differences between pre- and post-stenosis measurements [ ].
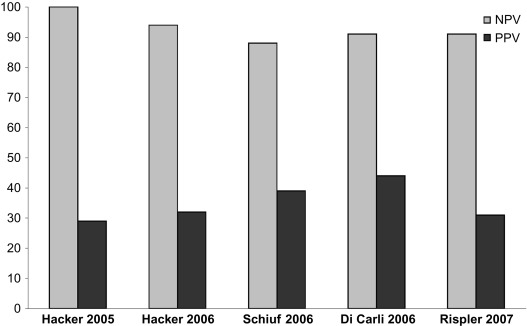
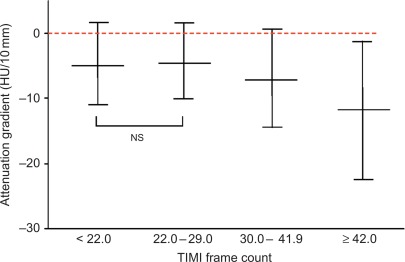
Recognizing that multiple beat acquisitions and dense mural calcifications hamper interpretation [ ], correction algorithms with dephasing of contrast delivery by relating coronary density to corresponding descending aortic opacification (TAG-CCO) or exclusion of dense calcified coronary segments (TAG-ExC) have been devised. It was shown that while CCO in arteries with lesions was lower, there was no significant difference between arteries with and without hemodynamically significant lesions.
Wong et al. studied an integrated approach using TAG, CT-Perfusion, and CTA compared to invasive FFR ≤ 0.80 as the gold standard [ ]. TAG significantly improved both sensitivity and specificity over degree of stenosis alone. Although TAG was shown to be highly reproducible and was better than CCO across a stenosis, however weaknesses of this study and other TAG studies include: small sample sizes, the need for artifact-free images along the arterial course, and the lack of information regarding individual lesions [ ].
7.3.9
Fractional flow reserve with CT
Clinicians have begun to recognize the importance of ischemia testing for guiding revascularization strategies. Although this has been well studied with noninvasive methods, recent studies have supported the use of invasive measures of fractional flow reserve (FFR) [ , ]. Practice guidelines support the use of FFR for the assessment of functional significance of moderate coronary stenoses when noninvasive functional information is not available [ ]. Initial analysis demonstrated that FFR detected ischemic lesions with sensitivity of 88%, specificity of 88%, and diagnostic accuracy of 93% [ , ]. The incorporation of FFR measures with ICA hopes to remove much of the oculostenotic reflex commonly observed with PCI.
While CTCA can detect and characterize anatomic stenoses, its ability to predict ischemia is modest ( Figure 7.7 ) [ ]. Recognizing the limitations of CTCA and anatomical imaging, several groups have examined the potential of CTCA to assess for ischemia [ , ].
A proprietary model using computational fluid dynamics has been used to estimate FFR with CT (FFR-CT) [ ]. “Diagnosis of Ischemia-causing Coronary Stenoses Obtained via Non-Invasive Fractional Flow Reserve” (DISCOVER FLOW) was a prospective trial where 103 CTCA patients were assessed with FFR-CT and compared to invasive FFR [ ]. Using invasive FFR < 0.8 as the gold standard, the per-vessel operating characteristics of FFR-CT were very good with an area under the curve of 0.9 for FFR-CT and 0.75 for CTCA. These results have since been confirmed in subsequent studies ( Table 7.3 ) [ , , , ]. The most recent study was performed with updated software and stricter adherence to imaging protocols and had promising results [ ]. Although encouraging, FFR-CT has limitations that include long processing times (approximately 5 h), and the need for CTCA images that are free of motion and artifact. The utility of FFR-CT in other populations, such as CABG and PCI patients, has not been studied.
