Normal Anatomy, Imaging Technique, and Common Variants
Anatomy of the Normal Spine
There are seven cervical vertebral bodies and eight cervical nerves. C1 is the atlas, a bony ring. C2 is the axis, with the dens extending superiorly. From C3 to C7 there is a gradual increase in size of the vertebral bodies. The uncinate processes are bilateral superior projections from the C3 to the C7 vertebral bodies that indent the disk and vertebral body above (posterolaterally), forming the uncovertebral joints. Laterally, the transverse foramen lies within the transverse process, and contains the vertebral artery. There is a normal slight increase in spinal cord size extending from C4 to C6. The neural foramina course anterolaterally at a 45-degree angle with a slight inferior course. These thus lie oblique to the standard sagittal and axial imaging planes. In the cervical region, the epidural venous plexus is prominent, with sparse epidural fat, the opposite to some extent of that in the lumbar spine. In regard to dermatomes, the hand is innervated by C6 (the thumb), C7 (the middle finger), and C8 (the little finger). Within any specific neural foramen, the nerve within the foramen is that corresponding numerically to the level below—for example, the C5 nerve lies within the foramen at C4–5.
There are 12 thoracic vertebral bodies. The ribs articulate with the vertebrae both at the disk level and at the transverse process (although for the latter only at T1–T10). The exit foramina for the basivertebral veins (part of the internal vertebral venous plexus) lie posteriorly within the mid-vertebral body, with the channel within the vertebral body itself often visualized as a Y in the axial plane (in both the thoracic and lumbar regions). In the thoracic region, epidural fat is often prominent posterior to the thecal sac and dura. Transitional type vertebrae are not uncommon at the thoracolumbar junction.
In the lumbosacral spine, there are five lumbar segments (vertebral bodies), five (fused) sacral segments, and four coccygeal bones (the coccyx). At all levels in the spine, the intervertebral disks are composed of a central gelatinous core (the nucleus pulposus, high signal-intensity [SI] on T2-weighted scans) with surrounding dense fibrous tissue (the annulus fibrosus, with low SI on T1- and T2-weighted scans). Because of the increased size of the intervertebral disks in the lumbar region, differentiation of the hydrated nucleus from the annulus fibrosus is easily recognized in normal disks. The bony parts of the lumbar vertebrae include the pedicles, transverse processes, articular pillars (pars interarticularis, superior and inferior articular facets), laminae, spinous processes, and vertebral body. The facet joints are diarthrodial synovial-lined and richly innervated.
On axial imaging the superior articular facet forms a “cap” anterolaterally with the inferior articular facet posteromedial connecting to the lamina. The ligamenta flava (bilateral paired ligaments, which connect the lamina of adjacent vertebrae, and are present from C2 to S1) extend from the anterior aspect of the upper lamina to the posterior aspect of the lower lamina. The epidural venous plexus is less prominent than in the cervical spine. In regard to dermatomes, the foot is innervated by L4 (medial big toe), L5 (midfoot), and S1 (little toe). Within any specific neural foramen (for both the thoracic and lumbar spine), the nerve within the foramen is that corresponding numerically to the level above—for example, the L4 nerve lies within the foramen at L4–5. Transitional vertebrae at the lumbosacral junction are quite common, with an incidence near 10%.
A single anterior spinal artery and two paired posterior spinal arteries supply the spinal cord. These vessels are fed by anterior and posterior radiculomedullary arteries. The major anterior radiculomedullary artery in the thoracic and lumbar region is the artery of Adamkiewicz, which usually arises between T9 and L1. There are typically no substantial anterior radiculomedullary feeders inferior to the artery of Adamkiewicz.
In the sagittal plane, the conus is commonly described in textbooks and in the anatomy literature to be located at L1–2. This statement is somewhat misleading. Looking specifically at the location of the tip of the conus, from published studies, this ranges in normal individuals from 10% occurring at the upper third of L1 to 10% occurring at the upper third of L2, with the maximum percentage (25%) occurring at the lower third of L1. A common clinical statement from magnetic resonance (MR) is that the conus in normal patients should lie at the T12–L1 level, with this statement referring to the conus itself and not specifically the tip. The posterior longitudinal ligament (which extends from the body of the axis to the sacrum) lies posterior to the vertebral bodies and anterior to the thecal sac, being in the lumbar region about 1 mm thick (anteroposterior [AP]) and 5 mm wide (left to right). The facet joints of the upper lumbar spine are oriented predominantly in the sagittal plane, while those of the lower lumbar spine are oriented more in the coronal plane.
On off-midline sagittal images, the dorsal root ganglion (and ventral root) is seen to lie in the superior portion of the neural foramen. In the lumbar region in particular, the sagittal plane is important for evaluation of foraminal narrowing, equal or greater in value than the axial plane. In regard to the neural foramen, the margins are composed of the disk and vertebral body anteriorly, the pedicles superiorly and inferiorly, and the facet joints posteriorly. In the axial plane, the bony canal and the thecal sac are well visualized, with the vertebral body anteriorly, pedicles laterally, and lamina posteriorly.
Imaging Technique
Because of its complexity, a description of the normal appearance of the spine on MR, specifically looking at the different sequences and parameter weighting, is important. T1-weighted images depict fat (and specifically the vertebral body marrow) as high SI, the spinal cord and intervertebral disks as intermediate SI (on very high quality images, gray and white matter within the cord can be distinguished on the basis of SI, on both T1- and T2-weighted scans), and cerebrospinal fluid (CSF) as low SI. Normally hydrated (nondegenerated) disks will appear slightly hypointense to the vertebral marrow. The normal ligamentum flavum is well seen, with intermediate SI. On thin section axial imaging, particularly in the lumbar spine, positioning of the slice relative to the vertebral body, end-plate, and disk can be differentiated on the basis of signal intensity, which changes from slightly high signal intensity (the fatty marrow of the vertebral body), to low (the endplate), to intermediate (the intervertebral disk). These structures can also be differentiated in a similar fashion on axial T2-weighted scans. Sagittal T1-weighted images (obtained without any obliquity) depict the neural foramina poorly due to their oblique orientation (except in the lower lumbar spine). T1-weighted scans typically can be acquired in a short scan time (2 to 4 minutes), with high signal-to-noise ratio (SNR) and high spatial resolution. Their general utility is for detection of structural abnormalities, marrow infiltration, degenerative disease, and abnormal contrast enhancement (following administration of a gadolinium chelate).
On T2-weighted images (specifically as typically acquired today, with fast spin echo technique), CSF and hydrated disks will have high SI, spinal cord intermediate SI, muscle low SI, and fat (including specifically that within the vertebral body marrow) intermediate to high SI. In the sagittal plane in adults, a central band of low SI is seen in normal disks, best visualized in the lumbar region due to the size of the disks. This structure, the “intranuclear cleft,” is due to fibrous transformation and is observed in all normal disks in patients over the age of 30. Lack of visualization of the intranuclear cleft should be considered abnormal, with such absence common in early degenerative disk disease. For improved visualization of soft tissue and marrow abnormalities on the basis of T2 changes, fat saturation should be employed in combination with T2-weighted fast spin echo imaging. The thecal sac is well assessed on T2-weighted images, with this scan technique also important for detection of spinal cord abnormalities (edema, gliosis, demyelination, and neoplasia).
Low flip angle gradient echo imaging is commonly employed in the cervical spine for axial T2-weighted imaging. On these scans, CSF and the intervertebral disks have high SI, the cord intermediate SI, and the marrow usually low SI (due to magnetic susceptibility effects, unless the scan is acquired with three-dimensional [3D] technique and a very short echo time [TE]). Good gray–white matter differentiation is seen within the cord. The utility of these scans is for detection of degenerative changes (disk herniation, canal compromise, foraminal stenosis) and evaluation of intrinsic cord abnormalities (multiple sclerosis [MS], tumors, edema, and hemorrhage). There may be a slight exaggeration of canal and foraminal stenosis due to magnetic susceptibility effects, in particular if the TE is substantially greater than 10 msec. This technique is (as with all gradient echo techniques) sensitive to, and commonly markedly degraded by, the presence of metal.
On MR there is a complex temporal evolution of vertebral body and disk appearance, in the infant, which is beyond the scope of this textbook. In the time frame from young adulthood to advanced age, the appearance of vertebral body marrow on MR is determined by the combination of red and yellow marrow, the former hematologically active marrow with lower T1 signal intensity when compared to yellow (fatty) marrow. With normal aging, there is a conversion from red to yellow marrow, which is reflected by higher signal intensity on T1-weighted scans of the vertebral marrow. With increasing age, both diffuse and focal replacement of red by yellow marrow occurs. Focal fat, as the latter is termed, is easily seen (due to its high signal intensity), and commonly encountered, on T1-weighted scans. Focal fat is seen more often in the elderly, and is identified as such by its isointensity to fat on all MR pulse sequences.
Attention should be paid to a few additional specifics in regard to MR acquisition technique, in order to obtain high-quality images. For both sagittal and axial acquisitions, if the phase encoding gradient is in the AP dimension, placement of a coronal saturation pulse (pre-saturation slab) is necessary to eliminate motion artifacts that might otherwise substantially degrade the image. In the cervical spine this includes motion of the mouth and swallowing. In the thoracic spine, cardiac motion can otherwise be a substantial problem. And in the lumbar spine motion artifacts originate from the aorta, vena cava, internal organs, and anterior abdominal fat (with respiration). For spine imaging the maximum slice thickness that should be acquired in any plane and any portion of the spine is 3 mm ( Fig. 3.1 ). On fast spin echo T2-weighted scans, signal loss within the thecal sac is common due to CSF pulsation, with this problem being most prominent in the cervical region (on axial images) and to a lesser extent in the thoracic region (on both axial and sagittal images). Axial images for optimal clinical interpretation should be angled parallel to the disk space, with state-of-the-art MR systems offering accurate and automatic vertebral body numbering as well as angling of axial images parallel to the disk space in both the AP and right to left dimensions. Image normalization is advocated in the lumbar spine in particular for routine use on axial images. Because of the use in MR of surface coils for spine imaging, structures located superficially, and thus close to the coil, will have artifactual high SI. When the window and center are chosen for display of the spinal canal, the posterior soft tissue structures are often obscured, being depicted with very high signal intensity due to their proximity to the surface coil. Image normalization is a post-processing feature that takes into account the sensitivity of the coil, and enables the visualization of structures both close and distant relative to the receiver coil, providing more homogeneous signal intensity across the field of view. It is important also to realize that today receiver coil coverage is integrated and continuous, allowing imaging of the spine in its entirety without gaps or image registration problems. Signal intensity drop offat the edge of the field of view, in the craniocaudal dimension, should not occur, and is indicative of an operator error. Thus, anatomic regions that were difficult to image in the past due to technical issues, including specifically the cervicothoracic and the thoracolumbar junctions, are well visualized on modern scanners ( Fig. 3.2 ).
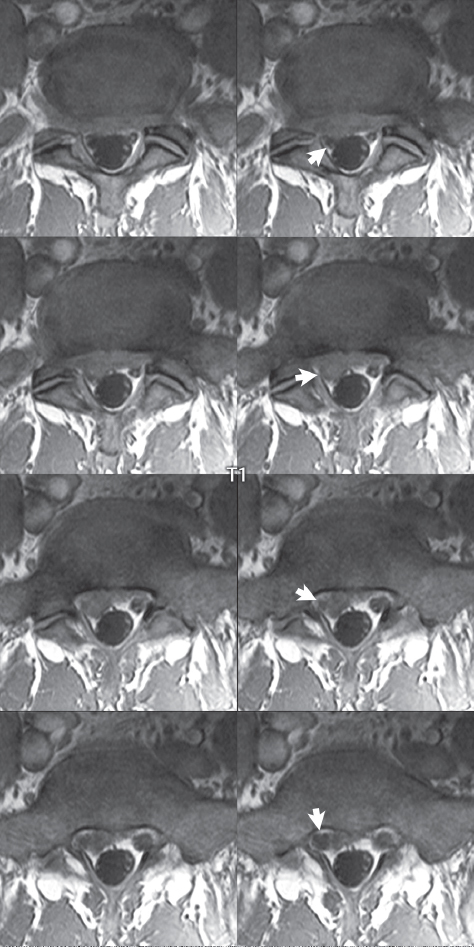
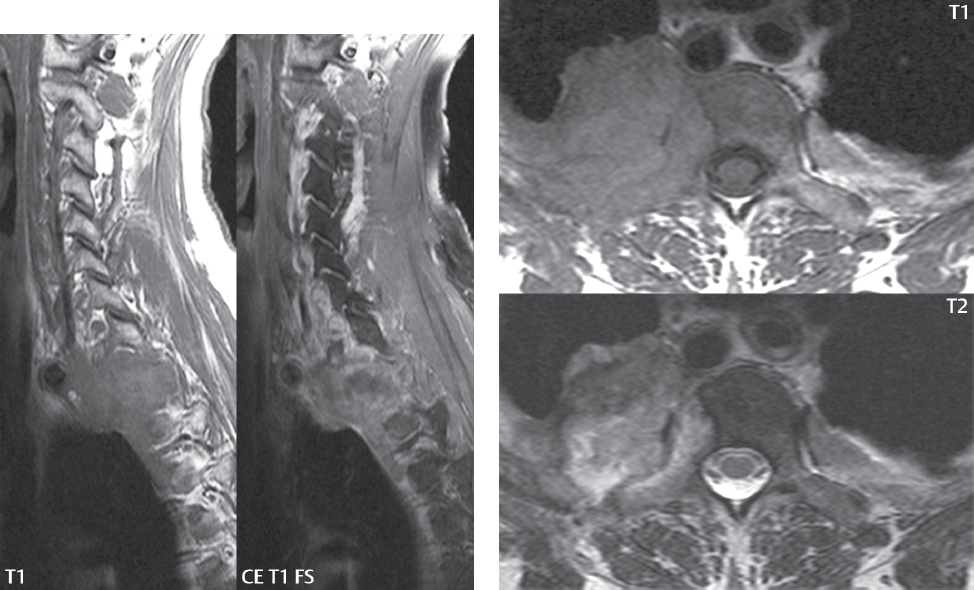
Following intravenous contrast enhancement, normal enhancing structures include, in particular, the venous plexus. The external vertebral plexus is a network of veins along the anterior vertebral body, laminae, and spinous, transverse, and articular processes. The internal vertebral plexus is a network of veins in the epidural space (the “epidural venous plexus”), both anteriorly and posteriorly. The basivertebral veins, seen on sagittal imaging centrally within the vertebral body, drain posteriorly into the anterior internal vertebral plexus. The anterior plexus is larger, with longitudinal veins on each side of posterior longitudinal ligament, which taper at the disk space level. Displacement and engorgement often accompany a disk herniation. These venous structures all drain via intervertebral veins that accompany spinal nerves within the neural foramina. An additional normal enhancing structure is the dorsal root ganglion.
The most common indication for administration of intravenous contrast in spine MR imaging is in the postoperative back (predominantly the lumbar spine) for the differentiation of scar from disk. On scans obtained within 20 minutes following contrast injection, scar enhances whereas recurrent or residual disk material does not. There will be prominent enhancement of the normal epidural venous plexus, which also improves depiction of the neural foramina and abnormal soft tissue therein. Contrast enhancement is also used routinely for evaluation of intradural, and soft tissue extradural, neoplastic disease. For extradural neoplasia, acquisition of post-contrast scans using fat saturation markedly improves recognition of abnormal contrast enhancement. Contrast enhancement is not normally administered when the clinical question is that of vertebral metastatic disease. This pathology is well-delineated in most cases without intravenous contrast administration. In certain instances, contrast administration can improve the depiction of vertebral metastatic disease. However, when used for this indication, scans must be performed with fat saturation. Otherwise the low signal intensity of a metastatic lesion (on a T1-weighted scan) may enhance to near isointensity with the adjacent high signal intensity of fatty marrow, reducing conspicuity. Contrast enhancement is also routinely used for other disease processes that involve the spinal cord, in particular ischemia and demyelination. Infection, whether intradural or extradural, is an additional major indication for contrast administration on MR.
Imaging of the spine in CT is typically performed without intravenous contrast administration. Depending on clinical symptoms and indications, intrathecal contrast administration may be performed prior to CT for improved depiction of the thecal sac, cord and nerve roots therein, and nerve root sleeves.
It should be kept in mind that spine imaging by both CT and MR is acquired in non-load bearing and on occasion may not reflect the positioning and pathology that would be present if the patient were imaged in an erect position. Flexion and extension views, which are extensively used in plain radiography, could be obtained with both computed tomography (CT) and MR but only in a limited fashion, and are not part of routine clinical practice.
It is also important to be familiar with the following terminology in spine imaging, which applies to lesion localization. An extradural (epidural) mass is one that is located outside the dura, and thus can cause, when of sufficient size, compression of the thecal sac. An intradural–extramedullary mass is one located within the thecal sac, but external to the cord. Such a lesion produces a filling defect in the CSF, outlined by a sharp meniscus above and below, with the ipsilateral subarachnoid space enlarged up to the level of the mass and the spinal cord deviated away from the mass. An intramedullary mass is one that involves (arises within) the spinal cord, and produces smooth cord enlargement with gradual effacement of the subarachnoid space. A block, which is an old term originating from myelography, is a level at which the subarachnoid space is obliterated. Depending on the location of the lesion causing the block, the thecal sac may be displaced (if extradural), and there can be cord compression (if the compression occurs at the level of, or above, the conus).
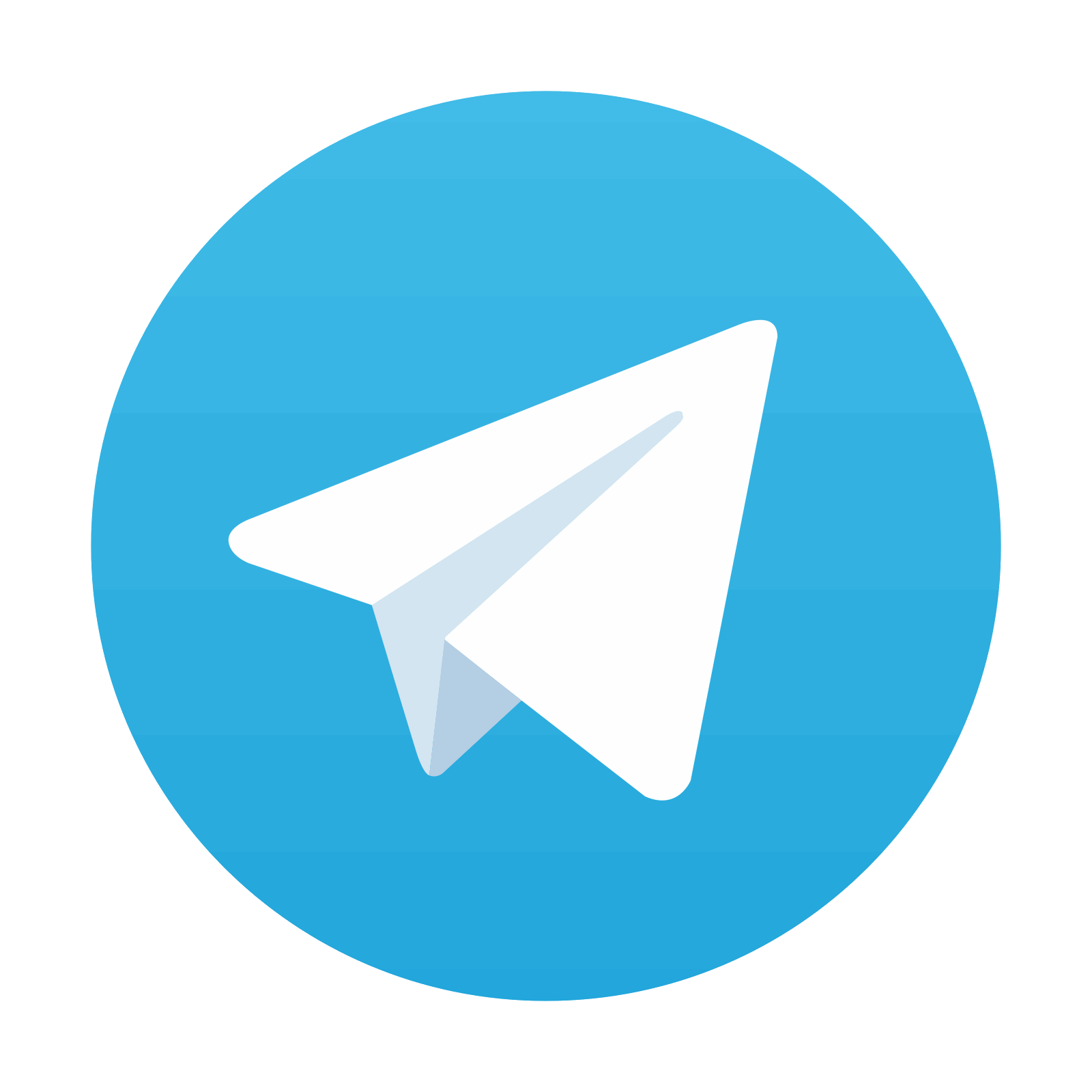
Stay updated, free articles. Join our Telegram channel

Full access? Get Clinical Tree
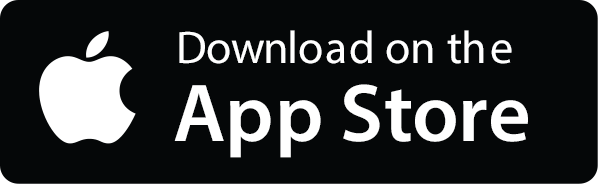
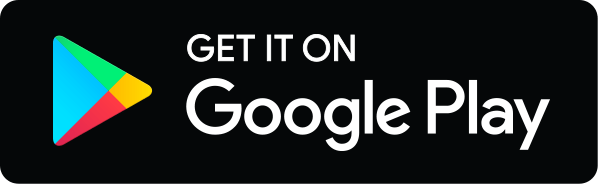