MRI is currently the best imaging modality to assess myelin maturation in the human brain. Myelin is the insulator for nerves and is present in both the peripheral nervous system and the central nervous system (CNS). In the CNS, it is a modified extension of the oligodendrocyte cell and is made up of multiple sheaths of protein-lipid-protein-lipid-protein. Standard T1-weighted and T2-weighted sequences can be performed on any MR imaging platform and with knowledge of normal age-related myelin maturation, myelin delay can be detected. Myelination progresses in a constant predetermined pattern from bottom to top, central to peripheral and back to front.
Key points
- •
MR imaging is the best noninvasive modality to assess myelin maturation in the human brain.
- •
A combination of conventional T1-weighted and T2-weighted sequences is all that is required for basic assessment of myelination in the central nervous system (CNS).
- •
It is vital to have an understanding of the normal progression of myelination on MR imaging to enable the diagnosis of childhood diseases including leukodystrophies as well as hypomyelinating disorders, delayed myelination, and acquired demyelinating disease.
Introduction
Assessment of the progression of myelin and myelination has been revolutionized in the era of MR imaging. Earlier imaging modalities such as ultrasonography and computed tomography have no current role or ability to contribute to the assessment of myelin maturation or abnormalities of myelin. The degree of brain myelination can be used as a marker of maturation.
The authors discuss
- 1.
Myelin function and structure
- 2.
The MR imaging appearance of myelin
- 3.
The normal progression of myelination on conventional MR imaging
- 4.
Terminal zones of myelination
Discussion
Myelin Function and Structure
To discuss normal myelination in the human brain, knowledge of the purpose and function of myelin and its role in the human nervous system is needed.
Myelin is present in both the CNS and the peripheral nervous system. In the CNS, it is primarily found in white matter (although small amounts are also found in gray matter) and thus is responsible for its color. Myelin acts as an electrical insulator for neurons. Myelin plays a role in increasing the speed of an action potential by 10–100 times that of an unmyelinated axon and also helps in speedy axonal transport. Edgar and Garbern (2004) demonstrated that the absence of a major myelin protein (PLP/DM20) from the oligodendrocyte resulted in major impairments in axonal transport in a mouse model of hereditary spastic paraplegia. It has also been well established that axonal integrity depends on the myelinating cell body for support. Myelin also likely has a role in the regulation of both ion composition and fluid volume around the axon.
Myelination is the formation of a myelin lipid bilayer around an axon. Myelination allows rapid transfer of information needed for cognitive functioning as well as emotional and behavioral functioning and decision making. Myelination begins during fetal life and continues after birth.
Myelin is a modified extension of an oligodendroglial cell process. An oligodendroglial cell is the key cell in myelination of the CNS and is the predominant type of neuroglia in white matter. Myelin sheaths are composed of multiple segments of myelin, which are then wrapped around an axon. This sheath is instrumental in containing an electrical current around an axon and increasing the action potential of an axon because of the nodes of Ranvier, which are sodium channels in between the myelin sheaths that increase the traveling speed of an electric current down an axon ( Fig. 1 ). Thus, myelin is thought to make impulses travel faster by increasing the speed of travel of a current. Myelin is also thought to be symbiotic with the axon. Myelin is metabolically active and involved in the turnover of its own components and contains a large number of myelin-intrinsic enzymes. Myelin also has a role in ion transport, which contributes to its own maintenance, and in the buffering of ions around the axon.
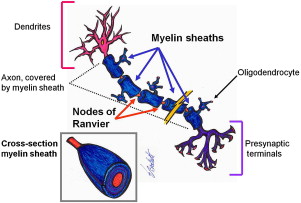
A single oligodendrocyte may be responsible for the production and maintenance of up to 40 fibers. Myelin has a high lipid content, having approximately 70% lipid and 20 to 30% protein. The main proteins that play a part in myelin structure are myelin basic protein (30%), proteolipid protein (50%), and cyclic nucleotide phosphodiesterase (4%). Other proteins involved include myelin-associated glycol protein and myelin oligodendrocyte protein. Lipids that contribute to myelin ultrastructure include cholesterol, phospholipids, and glycosphingolipids.
Electron microscopy has demonstrated that myelin is composed of multiple sheaths wrapped around the axon and that the sheaths are made up of a “protein-lipid-protein-lipid-protein” structure. Compaction of these sheaths or processes gives rise to apposition of extracellular and cytoplasmic surfaces, which represents alternating extracellular and intracellular spaces. The lipid bilayer is composed of phospholipids, glycolipids, and cholesterol. Most of the glycolipids (sulfatide and cerebroside) and cholesterol are in the outer layer and exposed to the extracellular space, whereas the phospholipids (plasmalogen) are in the inner layer and are hydrophobic. The formation of compact myelin is required for the growth and maturation of the axon.
The MR Imaging Appearance of Myelin
There is no technique that has been developed to view the myelin lipid bilayer directly with imaging. Myelin is assessed qualitatively. The commonly used MR techniques include conventional anatomic imaging, that is, T1-weighted and T2-weighted sequences as well as MR spectroscopy and diffusion tensor imaging (DTI). Other techniques that have been used include magnetization transfer imaging and T2 relaxation separation. In clinical practice, conventional anatomic imaging is the mainstay because it can be easily performed. Quantification of myelin can be achieved in multicomponent relaxation (MCR) analysis. MCR is a volume-weighted summation of distinct microanatomic water compartments. This analysis has revealed 2 water subdomains, namely, a slow relaxing species with free intracellular and extracellular water and a faster relaxing species of molecules from the water trapped between the lipid bilayer sheath.
Currently, standard MR imaging techniques do not specifically have the ability to quantitate myelin. Instead, these techniques study a combination of the following: changes in axonal size and density, changes in membrane structure including lipid and protein content, and water and macromolecule content. DTI is not a reliable indicator of the total amount of myelin, but it can give some information on changes in myelin.
As reviewed by Barkovich there are 2 distinct populations of water molecules that play a direct role in the signal characteristics of myelin on MR imaging. These populations are water located within the myelin sheath and that located outside the myelin sheath. On conventional imaging, mature myelin is hyperintense to the gray matter cortex on T1 and hypointense to the gray matter cortex on T2. However, on T1, this increase in signal is most likely because of increasing glycolipids, predominantly galactocerebroside and cholesterol, within the myelin membranes. The T2 hypointensity is thought most likely due to the reduced water content as more myelin is laid down with greater maturation of the myelin sheath, and tightening of the myelin spiral around the axon.
The Normal Progression of Myelination
The general rule of progression of normal myelination as outlined by Barkovich is that myelination begins in the fifth fetal month and continues throughout life. Myelination commences with the cranial nerves, which makes sense because we need these to rely on for survival. Generally, myelination progresses from bottom to top (caudocranial), back to front (posterior to anterior), and central to peripheral (deep to superficial) ( Fig. 2 ). It therefore makes sense that the brainstem and cerebellum myelinate before the cerebrum and that the basal ganglia and thalami commence myelination before the white matter. In addition, the posterior limb of the internal capsule myelinates before the anterior limb, splenium before the genu, and the central corona radiata before the subcortical regions.
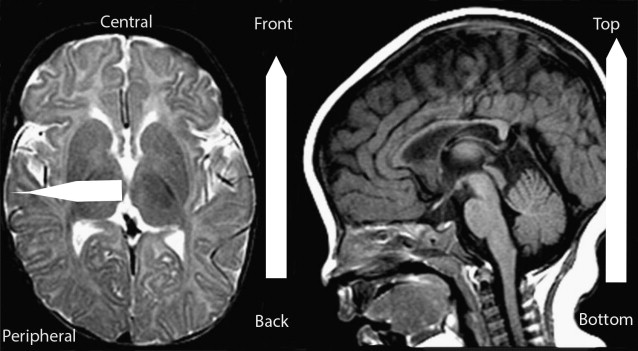
Counsell and colleagues described myelination in the very preterm infant and confirmed myelination in the cerebellar vermis, vestibular nuclei, cerebellar peduncles, dentate nucleus, medial longitudinal fasciculus, medial geniculate bodies, subthalamic nuclei, inferior olivary nuclei, ventrolateral nuclei of the thalamus, medial and lateral lemnisci and inferior colliculi, as well as gracile and cuneate nuclei and fasciculi. The investigators did not find any new myelin sites between 28 and 36 weeks, after which there were again new myelin sites at the posterior limb of internal capsule, corona radiata, and the corticospinal tracts of the precentral and postcentral gyrus.
Histologic studies demonstrate myelination at birth in the brainstem, cerebellar white matter, and posterior limb of the internal capsule with extension to the thalamus and basal ganglia.
Bird and colleagues (1989) reviewed 60 patients on MR imaging and found that there was wide variation in the rate, onset, and appearance of changes associated with myelination. The investigators studied marker sites for certain ages in determining normal myelin. For example, at birth (term), there was mature myelination in the posterior limb of the internal capsule, the cerebellar peduncles, and the corona radiata around the central sulcus. The slowest areas to myelinate were the central white matter of the supratentorial lobes. The investigators again consistently confirmed progression of myelination in the posterior limb before the anterior limb, splenium before genu, and central corona radiata before poles in all subjects. This posterior to anterior sequence has been seen in autopsy subjects.
Paus and colleagues (2001) described 3 developmental patterns seen with respect to gray–white matter differentiation in the first 12–24 months of life. These patterns are the infantile pattern for less than 6 months with a reversal of the normal adult pattern, the isointense pattern (8–12 months) in which there is poor differentiation between gray and white matter, and the early adult pattern (greater than 12 months) in which gray matter signal is greater than that of white matter on T2 and less than that of white matter on T1. This change is thought to be related to changes in relaxation times with rapid shortening in the first 12 months because of a rapid decrease in the water content of gray and white matter.
Welker and Patton (2011) recently published a table of age-specific progression of myelination on MR imaging with reference to T1, T2, Fluid-attenuated inversion recovery (FLAIR), and DTI sequences ( Figs. 3–11 ). The reader is referred to this article for further detail.
