Diffusion tensor imaging (DTI) has allowed in vivo demonstration of axonal architecture and connectivity. This technique has set the stage for numerous studies on normal and abnormal connectivity and their role in developmental and acquired disorders. Referencing established white matter anatomy, DTI atlases, and neuroanatomical descriptions, this article summarizes the major white matter anatomy and related structures relevant to the clinical neuroradiologist in daily practice.
Key points
- •
Diffusion tensor imaging (DTI) has emerged as an excellent tool for in vivo demonstration of white matter microstructure and has revolutionized our understanding of the same.
- •
Information on normal connectivity and relations of different white matter networks and their role in different disease conditions is still evolving. Evidence is mounting on causal relations of abnormal white matter microstructure and connectivity in a wide range of pediatric neurocognitive and white matter diseases.
- •
Hence there is a pressing need for every neuroradiologist to acquire a strong basic knowledge of white matter anatomy and to make an effort to apply this knowledge in routine reporting.
Introduction
DTI has allowed in vivo demonstration of axonal architecture and connectivity. This technique has set the stage for numerous studies on normal and abnormal connectivity and their role in developmental and acquired disorders. Referencing established white matter anatomy, DTI atlases, and neuroanatomical descriptions, this article summarizes the major white matter anatomy and related structures relevant to the clinical neuroradiologist in daily practice.
MR imaging of white matter tracts
White matter is seen well on the T1, T2, and fluid-attenuated inversion recovery (FLAIR) sequences used in routine MR imaging. Certain white matter tracts are reasonably well demonstrated particularly on T2 and FLAIR images because of their location and in the pediatric age group, because of the differences in water content and myelination ( Fig. 1 ). However, the use of specific DTI sequences provides far more detailed and clinically useful information.
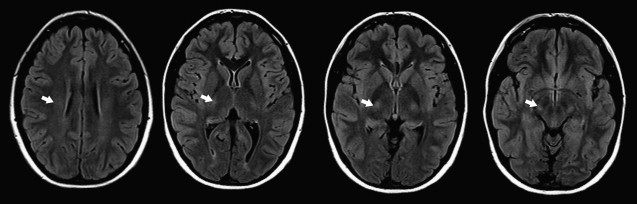
Diffusion tensor imaging: the basics
Using appropriate magnetic field gradients, diffusion-weighted sequences can be used to detect the motion of the water molecules to and from cells. This free movement of the water molecules is random and thermally driven in neurons. In the axons, the axonal membranes and myelin sheaths act as barriers to the random motion of the water molecules, and this motion thus becomes directionally dependent or anisotropic, with the direction of maximum diffusivity aligning with the direction of white matter tract orientation. With DTI, this degree of anisotropy and fiber direction can be mapped voxel by voxel, allowing for the in vivo assessment of white matter tract architecture.
A diffusion tensor is a mathematical model containing diffusion measurements from at least 6 noncollinear directions, from which diffusivity in any direction as well as the direction of maximum diffusivity can be estimated. Using more than 6 encoding directions will improve the accuracy of the tensor measurements, and DTI is more often obtained with 30 to 60 directions. The tensor matrix is ellipsoid, with its principal axis oriented in the direction of maximum diffusivity. Via a linear algebraic procedure called matrix diagonalization, 3 eigenvalues are obtained, which represent apparent diffusivity in the 3 principal axes of the ellipsoid, namely the major , medium, and minor axes, also known as the eigenvectors .
The x- , y- , and z- coordinate system to which the scanner is oriented is rotated to a new coordinate system, dictated by diffusivity information. Fractional anisotropy (FA) is derived from the standard deviation of the 3 eigenvalues and ranges from 0 (isotropy) to 1 (maximum anisotropy). The orientation of maximum diffusivity may be mapped using red, green, and blue color channels and color brightness, modulated by FA, and this can result in the formation of a color map demonstrating the degree of anisotropy and local fiber direction.
The conventional color coding is green for fibers oriented anteroposterior (mainly the association fibers), red for right–left oriented fibers (mainly commissural fibers), and blue for superior–inferior fibers (in particular as projection fibers). In 2D images ( Fig. 2 ), mixed color is seen when fibers overlap, resulting in yellow (green and red), magenta (red and blue), and cyan (green and blue), and with changes in orientation (see Fig. 2 ).
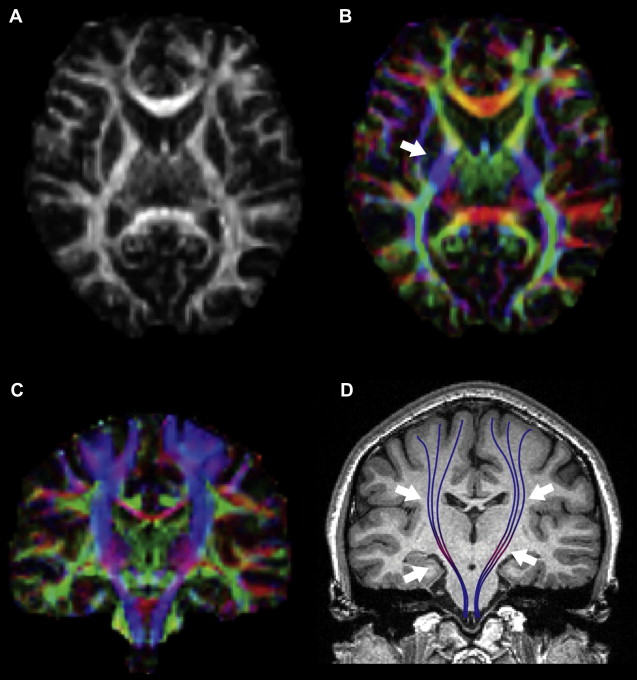
Classification of white matter tracts
The white matter tracts are broadly classified into 3 groups according to their connectivity ( Table 1 ):
- 1.
Projection fibers : These fibers connect the cortical areas with the deep gray nuclei, brainstem, cerebellum, and spinal cord or vice versa. Corticospinal fibers, corticobulbar fibers, corticopontine fibers, thalamic radiations, and geniculocalcarine fibers (optic radiations) are tracts identifiable on DTI.
- 2.
Association fibers : These fibers connect different cortical areas within the same hemisphere. The fibers can be long range or short range, the latter including subcortical U fibers. The major long tracts include cingulum, superior and inferior occipitofrontal fasciculus; uncinate fasciculus; superior longitudinal fasciculus (SLF), including arcuate fasciculus; and inferior longitudinal fasciculus (occipitotemporal).
- 3.
Commissural fibers : These fibers connect similar cortical areas in the 2 hemispheres, including the corpus callosum and the anterior commissure.
White Matter Tracts | Connection | Function |
---|---|---|
Cingulum | Cingulate gyrus to the entorhinal cortex | Affect, visceromotor control; response selection in skeletomotor control; visuospatial processing and memory access |
Fornix | Hippocampus and the septal area to hypothalamus | Part of the Papez circuit; critical in formation of memory; damage or disease resulting in anterograde amnesia |
Superior longitudinal fasciculus | Frontotemporal and frontoparietal regions | Integration of auditory and speech nuclei |
Inferior longitudinal fasciculus | Ipsilateral temporal and occipital lobes | Visual emotion and visual memory |
Superior fronto-occipital fasciculus | Frontal lobe to ipsilateral parietal lobe—name being a misnomer | Spatial awareness, symmetric processing |
Inferior fronto-occipital fasciculus | Ipsilateral frontal and occipital, posterior parietal and temporal lobes | Integration of auditory and visual association cortices with prefrontal cortex |
Uncinate fasciculus | Frontal and temporal lobes | Auditory verbal and declarative memory |
Thalamic radiations | Lateral thalamic nuclei to cerebral cortex through internal capsule | Relay sensory and motor data to precentral and postcentral cortex |
Corticofugal fibers (descending projection fibers) | Motor cortex and cerebral peduncle through internal capsule | Descending motor fibers from primary motor cortex, ventral and dorsal premotor areas, and supplementary motor areas |
Corpus callosum | Corresponding cortical areas of both hemispheres | Interhemispheric sensorimotor and auditory connectivity |
Anterior commissure | Olfactory bulbs and nuclei and amygdala | Integral part of the neospinothalamic tract for nociception and pain sensation |
Other tracts and fibers, which can be seen in DTI maps, include the optic pathway, fornix, and many fibers within the cerebellum and brainstem. These tracts and fibers are described separately.
Projection fibers
Projection fibers are afferent and efferent tracts that interconnect areas of the cortex with the brainstem, deep nuclei and cerebellum, and spinal cord. Of these, the main ones identifiable on DTI include the corticospinal, corticobulbar, corticopontine, and geniculocalcarine tracts (optic radiations).
Corticospinal Tracts
Corticospinal tracts are descending projection tracts connecting the motor area to the spinal cord (see Fig. 2 ; Fig. 3 ). Corticospinal tracts have long been believed to arise from the motor cortex of the precentral gyrus. In a study of 42 healthy children using DTI, Kumar and colleagues showed that the fibers originate in both precentral and postcentral gyrus in 71% of older children, followed by precentral gyrus, and least commonly from the postcentral gyrus. This pattern was not influenced by hand preference.
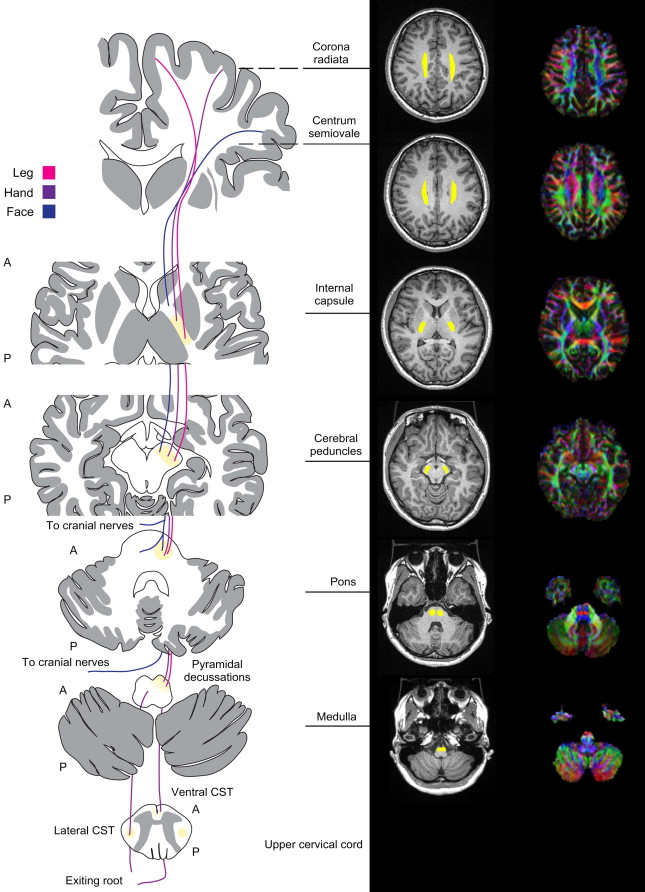
From the cortices, the fibers converge into the corona radiata . Here the more anterior fibers represent those servicing the face and the posterior fibers represent those that connect to the lower limb with fibers in between representing the hand. Fibers then occupy the posterior aspect of the posterior limb of internal capsule (PLIC), beginning more anteriorly at the middle of the PLIC and then shifting more posteriorly as the tracts descend. Fibers representing the hand are anterior to those of the feet.
At the level of the cerebral peduncles, the corticospinal tracts occupy the middle third of the crus cerebri . Here the face fibers run medial to the fibers representing the feet, with the fibers representing the hand once again in between. The fibers travel within the basis pontis before passing through the anterior medulla, forming the medullary pyramids . At the level of the caudal medulla, most of the fibers (75%–90%) in the pyramids cross to the contralateral side, forming the pyramidal decussation of Mistichelli . These fibers then descend as the lateral corticospinal tract within the posterior part of the lateral funiculus of the medulla spinalis . Of the fibers that do not cross, the majority travel in the anterior column on either side of the median fissure as the anterior corticospinal tracts , historically known as the bundle of Türck . These tracts decussate where they terminate, at their respective spinal level within the contralateral anterior horn gray matter.
There are variations in this anatomy with some fibers not crossing to form ipsilateral lateral corticospinal tracts and others decussating to form contralateral anterior corticospinal tracts. Approximately 2% of the corticospinal tract remains truly ipsilateral, running in the ventrolateral funiculus as the bundle of Barnes to supply axial muscles of the trunk and proximal limbs.
Likewise, there may be some asymmetry in the corticospinal tracts. The left corticospinal tract has higher FA values and lower transverse diffusivity because of high myelin content. There is an increase in FA within the corticospinal tracts with increasing age. Partially uncrossed pyramidal tracts are very rare ( Fig. 4 ) and have been described by Alurkar and colleagues and have been described with horizontal gaze palsy and scoliosis by Mori and colleagues in 2005.
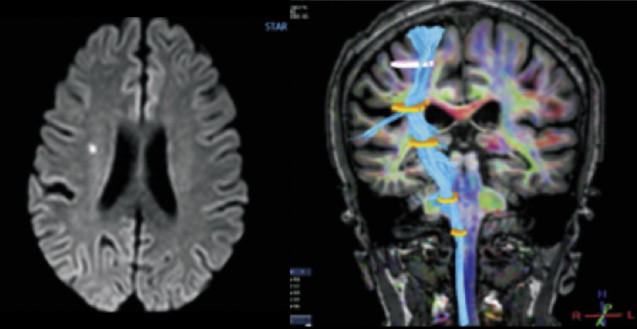
Corticobulbar Tracts
Corticobulbar tracts connect the motor cortex to cranial nerve nuclei in the brainstem. These fibers pass through the corona radiata and genu of internal capsule to run mediodorsal to the corticospinal tracts at the level of the cerebral peduncles.
Corticopontine Tracts
Corticopontine fibers arise from precentral and postcentral gyri with substantial contributions from premotor, supplementary motor, and posterior parietal cortices as well as from prefrontal and temporal cortices. The fibers course through the anterior limb of the internal capsule and medial cerebral peduncle before projecting into the pontine nuclei. Second-order neurons from the pontine nuclei decussate to the contralateral side and give rise to pontocerebellar pathways.
The corticospinal, corticopontine, and corticobulbar tracts run together and cannot be identified separately from each other using DTI but may be parcellated using advanced color maps.
Internal Capsule
The internal capsule is the main conduit for projection fibers and is divided into 3 main sections. The anterior limb of internal capsule lies between the lentiform nucleus and the head of the caudate nucleus and carries the anterior thalamic radiations and frontopontine tracts. The PLIC separates the posterior aspect of the lentiform nucleus and the thalamus and contains corticospinal, corticobulbar, and frontopontine fibers; the superior thalamic radiation; and a smaller number of fibers connecting to the tectal, rubral, and reticular systems.
The PLIC can be further subdivided into thalamolenticular, sublenticular, and retrolenticular segments. Within the retrolenticular portion of the PLIC runs the posterior thalamic radiation (which includes the optic radiation) and the corticotectal, corticonigral, and corticotegmental fibers. The sublenticular portion of the PLIC contains the inferior thalamic radiation, auditory radiation, and temporal and parieto-occipital corticopontine fibers.
The intervening genu of the internal capsule between the anterior and posterior limb contains corticobulbar and corticoreticular fibers as well as some frontopontine and superior thalamic radiation fibers.
Thalamic Radiations
The thalamus is known to have reciprocal connections (corticothalamic and thalamocortical fibers) to wide areas of the cortex. These fibers pass through the anterior and posterior limbs and retrolenticular segment of the internal capsule as the anterior, superior, and posterior thalamic radiations ( Fig. 5 ) and fan out to form the corona radiata.
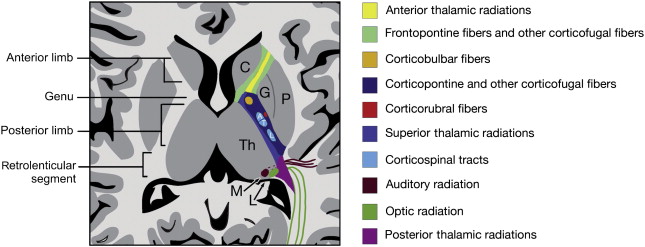
Reciprocal connections include the anterior nucleus and cingulate cortex, ventral lateral nucleus and motor cortex, ventral anterior nucleus and supplementary motor area, ventral posterior nucleus and sensory cortex, lateral geniculate body (LGB) and visual cortex, medial geniculate body and primary auditory cortex, and dorsomedial nucleus and prefrontal cortex ( Fig. 6 ).
