Fig. 50.1
Neuromembrane events in TBI. Scheme demonstrating the complex cellular and vascular pathophysiological interactions occurring following TBI and the central role of mitochondrial failure that relates to all neuromembrane events (Bigler and Maxwell 2012)
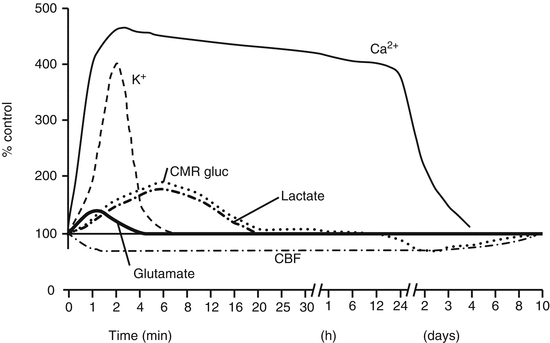
Fig. 50.2
Neurometabolic cascade following experimental concussion. K+ potassium, Ca2+ calcium, CMRgluc oxidative glucose metabolism, CBF cerebral blood flow (Giza and Hovda 2000)
Immediately after the mechanical injury of the brain, there is a cellular response with a widespread release of excitatory neurotransmitters (i.e., glutamate) and an uncontrolled ion flux. The binding of glutamate to its receptors leads to further neuronal depolarization, with efflux of potassium (K+) and influx of calcium (Ca2+), sodium (Na+), and chloride (Cl−). This ionic shift causes a failure to generate and propagate action potentials, leading to acute and subacute changes at cellular level. This process will end in a secondary axotomy 4 h to 12 weeks after the mTBI (with a minimum of 2 h in animals and 12 h in human beings).
In the acute stage, the sodium-potassium (NA+-K+) pumps increase their function by trying to restore the neuronal membrane potential, with the corresponding increment in production of adenosine triphosphate (ATP). Triggering of the glucose metabolism into a diminished cerebral flow state creates a mismatch between glucose supply and the demand of energy that ends in a cellular energy crisis and failure of the ATP-dependent membrane pumps.
In the subacute phase, after the initial period of increased glucose consumption, the brain undergoes a period of depressed metabolism. The reduction in ATP production creates a failure in ATP-dependent membrane pumps, with a reverse pumping of Na+/Ca2+ exchangers. This leads to a marked increase of Ca2+ concentration in the cytoplasm, which is sequestrated by the mitochondria, causing damage to it and worsening the energy crisis. This process starts a cascade of reactions including oxygen radical production, disruption of protein phosphorylation, formation of proteases and free fatty acids (e.g., arachidonic acid), depolymerization of microtubules, collapse and loss of neurofilaments, and, in later stages, separation of myelin lamellae.
It is important to understand that this complex pathophysiologic sequence of events and metabolic changes occurs prior to what can be visualized using conventional CT and MRI, while it can be the target of the more specific nuclear imaging techniques aiming to visualize particular metabolic processes.
50.3 SPECT
50.3.1 SPECT in TBI
SPECT is a functional imaging technique extensively used in mTBI, essentially to determine the cerebral blood flow (CBF) based on technetium-99m-hexamethylpropyleneamine oxime (99mTc-HMPAO) or technetium-99m-ethyl cysteinate dimer (99mTc-ECS). From the aforementioned SPECT perfusion tracers, 99mTc-ECD has been proven to distinguish more and especially smaller functional deficits in mTBI than 99mTc-HMPAO (Otte et al. 1997).
SPECT scans are available at most major hospitals with a relatively low cost of about US $800 (Anderson et al. 2005). Different reviews on this neuroimaging modality outline the high negative predictive value of the perfusion SPECT scans during the acute phase in mTBI (Cihangiroglu et al. 2002; Davalos and Bennett 2002; Belanger et al. 2007; Lin et al. 2012).
Other additional radiopharmaceuticals have also been utilized to study concussion. CBF measures with iodine-123 N-isopropyl-p-iodoamphetamine (123IMP) in conjunction with iodine-123 iomazenil (123IMZ), to measure neuronal integrity by binding to benzodiazepine receptors, were used to study nine chronic mTBI patients. In all of these a significant increase in benzodiazepine receptor uptake of the prefrontal cortex could be shown as compared with matched control subjects (Hashimoto et al. 2007). Finally, a study with cobalt-57 chloride SPECT (57CoCl2) (Audenaert et al. 2003), which is suggested to target calcium in the brain, was performed in 8 mTBI patients 2 days after the injury. In this study, a 57CoCl2 accumulation in frontal and temporal lobes, with additional accumulation in the posterior parietal occipital region, could be shown in accordance with hypoperfusion measured by 99mTc-HMPAO. By contrast, CT and EEG did not detect (structural) lesions in any of these cases. The aforementioned studies are only pilot studies, and with the small number of subjects included in them, it is indeed difficult to determine the general validity of their findings. However, they open new avenues to make the SPECT technique a valid alternative for studying mTBI, both for clinical practice and for research.
50.3.2 SPECT in Sports-Related Head Injuries
Although SPECT on concussion in the acute stages of sports-related head injuries is not available to date, some studies have explored the chronic stages of repetitive concussions in boxing and American football. In a 99mTc-HMPAO SPECT study, Kemp and colleagues (1995) have compared the CBF between active amateur boxers and a control group of healthy athletes. They reported that out of 34 boxers, 14 (41 %) had abnormal cerebral perfusion when compared with an “atlas of normality” (database of images obtained from healthy controls) (Houston et al. 1994), while abnormalities were observed only in 5 (14 %) of the controls. They also reported significant correlations between behavior deficits and abnormalities seen by SPECT. The same group of amateur boxers was reanalyzed by Houston et al. (1998), including other groups (undersea divers, schizophrenic patients, and Alzheimer’s disease patients) for comparison. Boxers exhibited large regional CBF abnormalities (1.05 % of cortical voxels) and presented at least one large lesion (>10 voxels, each with a side length of 0.64 cm) in eight of the nine regions of interest, including the left and right frontal, parietal, and inferior temporal lobe, right inferior frontal lobe, and occipital region.
More recently, three studies by Amen and colleagues have investigated the chronic stages of repetitive concussion in retired American football players. In their first experiment (Amen et al. 2011a) 100 subjects were recruited from the National Football League (NFL), representing different teams and all positions, and compared with healthy matched controls. The study, using 99mTc-HMPAO SPECT, revealed a global decrease in CBF, especially in the prefrontal, temporal, and occipital lobes, anterior and posterior cingulate gyrus, hippocampus, and cerebellar region (Fig. 50.3). The use of Z scores to analyze the results, instead of the generally used number of hypoperfusion regions, makes it difficult to compare the data with other SPECT studies (Lin et al. 2012). However, their findings seem to correspond with CBF changes found in other general studies about mTBI. In a second study, Amen et al. (2011b) examined 30 retired NFL players before and after an intervention based on weight loss and multiple supplements, such as fish oil, vitamins, ginkgo biloba, acetylcholine, and antioxidants. Initial SPECT scans were compared with the follow-up scans using a paired t-test. They found increased brain perfusion in the prefrontal cortex, parietal and occipital lobes, anterior cingulate gyrus, and cerebellum. Unfortunately, there was no control group and no randomization in the study, so it is rather difficult to answer the question of whether or not the results are due to the intervention. In the last study (Willeumier et al. 2012), and using the same data pool, the CBF of 38 overweight (waist-to-height ratio = 58.7 ± 4.7) retired NFL players was compared with the same number of normal-weight players (49.34 ± 2.8). The study revealed that overweight athletes present a decreased CBF in the dorsolateral prefrontal cortex (Brodmann areas 8 and 9), the anterior prefrontal cortex (Brodmann area 10), and the left temporal pole. These brain regions are involved in attention, reasoning, and executive function.
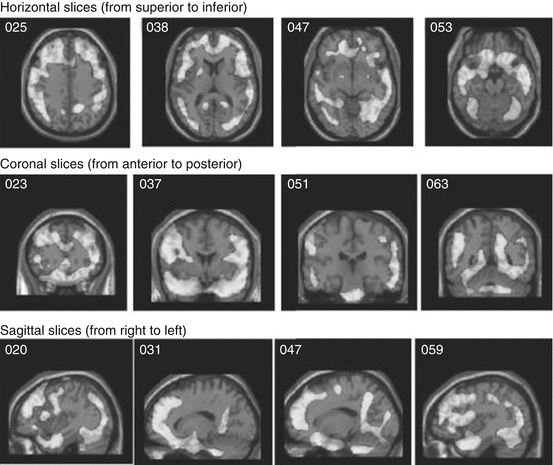
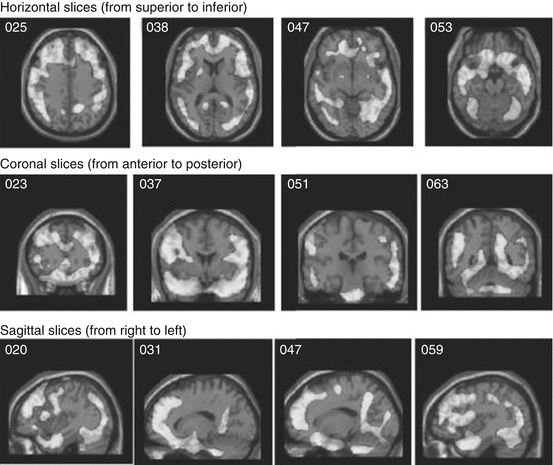
Fig. 50.3
Global brain HMPAO SPECT decrease in NFL players versus healthy subjects. Light areas indicate decreased perfusion in the NFL players versus healthy-brain comparison subjects at p < 0.0001, family-wise error. No increases were seen (Amen et al. 2011a)
50.3.3 SPECT: Discussion and Future Perspective
Advances in SPECT detector systems and hybrid SPECT-CT, development of new tracers, the application of modern methods of image analysis, and the relatively low cost of each scan make the SPECT an interesting technique for the study of concussion. Nevertheless, some issues still remain to be resolved. First, while studies have shown that SPECT is more sensitive in TBI than CT or MRI (Ichise et al. 1994), it may not be highly specific, as there might be other conditions showing changes in the same brain regions (Wortzel et al. 2008). And second, SPECT image analysis tends to rely on the comparison of regions of interest considered “normal,” but due to the diffuse nature of the concussion, it may be difficult, if not impossible, to obtain an area not affected. Therefore, implementation of new methods to process the data is needed, such as the use of voxel-based analysis with statistical parametric mapping (SPM, Wellcome Department of Cognitive Neurology, University College London) (Friston et al. 1991, 1995).
The understanding of concussion with the help of SPECT would also benefit with the use of different gamma-emitting radioligands. For example, the combination of 123I-2-β-carbomethoxy-3-β-(4-iodophenyl)tropane (β-CIT) and 123I-iodobenzamide (IBZM): In moderate and severe TBI, these two tracers have shown a nigrostriatal dysfunction, although the striatum was structurally preserved (Donnemiller et al. 2000). Another recent animal study has used a newly developed radiotracer, N′,N′-diethyl-6-chloro-(4′-[123I]iodophenyl)imidazo[1,2-a]pyridine-3-acetamide (123I-CLINDE), to monitor in vivo neuroinflammation (Mattner et al. 2011). Especially the development of new radioligands may be helpful for the understanding of the pathomechanism of concussion and mTBI.
50.4 PET
One of the main advantages of PET over SPECT is its higher sensitivity that allows generating images of greater resolution (e.g., Otte and Halsband 2006). The usefulness of this technique in the study of traumatic brain injuries was shown in several clinical settings (e.g., Hattori et al. 2003). However, no meta-analyses have clarified its importance due to the heterogeneity of the published studies (differences in the time elapsed between trauma and scan, differences in the TBI classification, use of different radiotracers, or different methodologies for the image analysis). Despite the large number of these studies including moderate and severe injuries, not much research has been done to address expressly the mild injuries or the concussions in sports. Apart from availability and the need for a nearby cyclotron, this can also be explained by the high cost of PET exams, usually ranging between US $1,000 and $3,000 (Rushing et al. 2012).
50.4.1 PET in TBI
The most common radiotracer used in clinical PET imaging is 2-deoxy-2(18F)-fluoro-d-glucose (FDG), with its first studies applied to TBI patients dating back to the 1980s and 1990s (Rao et al. 1984; Langfitt et al. 1986; Alavi 1989; Alavi et al. 1997; Tenjin et al. 1990; Yamaki et al. 1996; Fontaine et al. 1999). In these studies, it was pointed out that abnormalities can be detected by FDG more extensively than those observed by structural images, such as CT, and this applied even to early stages after mTBI, where CT or MRI was still negative. Since these first experiments, the brain metabolism in acute phases of TBI was studied, and the existence of a triphasic pattern was found in the cerebral metabolic rate of glucose (CMRglc), measured by FDG PET (Bergsneider et al. 1997, 2000, 2001). These phases, observed also in animal models, are divided into:
1.
Hyperacute increase of metabolic activity
2.
Prolonged period of reduced metabolism, of about a month
3.
Recovery of stable levels within normal limits
It is important to notice that these phases are independent of the TBI severity and the level of consciousness measured by the Glasgow Coma Scale (GCS) (Bergsneider et al. 2001). Therefore, most of what will be described below can be applied to mild head trauma injuries or concussions, although not many specific researches have been undertaken on this group of subjects.
In the acute phase after the trauma, the whole brain maintains a low metabolic state. While brain CMRglc values cannot reflect the level of consciousness, more recent experiments using a new generation of PET scanners with better spatial resolution have demonstrated a direct association between the level of consciousness measured by GCS and particular CMRglc values in the thalamus, brain stem, and cerebellum (Hattori et al. 2003). Some of the subsequent studies have included the radiotracer H2 15O to evaluate also the cerebral blood flow (CBF), clarifying the role of glucose metabolism in the acute phases of TBI. A reduction of hexokinase activity in the whole brain was demonstrated, including apparently undamaged brain areas, while glucose transport and CBF were only reduced in pericontusional areas (Hattori et al. 2004). Moreover, a selective reduction of CMRglc and hexokinase activity was found in the gray matter and not in the white matter. Interestingly, this reduction was not observed in the CBF, measured with H2 15O, and was not related with any evidence of damage based on conventional MRI images (Wu et al. 2004a, b).
While many individuals are capable to recover back to stable functional levels after the acute phase, others develop more chronic stages. The wide variety of chronic cognitive, emotional, and behavioral alterations that configure the so-called post-concussive syndrome cannot be solely explained by these initial changes in glucose metabolism. In this context, it was shown that initial oxidative metabolic dysfunction can lead to chronic brain atrophy, especially in brain areas not directly damaged during trauma (Xu et al. 2010). Neural network connecting cortical and subcortical regions are also crucial to maintain normal cognitive function (Bassett and Bullmore 2009). As previously mentioned, impaired function in the thalamus or brain stem can alter the normal status of these networks, reflected, for example, in the differences in loss of consciousness after a TBI. This disconnection or malfunction between subcortical and cortical levels may persist in more chronic stages. Several studies have shown bilateral hypometabolism in the prefrontal medial region, medial frontobasal region, anterior and posterior regions of the cingulate gyrus, and the thalamus. Each of these areas is an essential part of the cognitive networks (Nakayama et al. 2006). In addition, subsequent studies have shown metabolic deficits in both the temporal lobe and right cerebellum (Kato et al. 2007) and medial region of cingulate gyrus (Nakashima et al. 2007). Like in acute phases, abnormal patterns of the metabolism are similar in patients with or without structural lesions observed by CT or MRI (Lull et al. 2010; Zhang et al. 2010; García-Panach et al. 2011).
50.4.2 PET in Sports-Related Head Injuries
Unlike SPECT, only few PET studies have focused on mTBI. Most of them report similar diffuse cortical-to-subcortical abnormal patterns as those presented in moderate and severe trauma. This includes alterations in glucose metabolism in the frontal, temporal, and parietal lobes, prefrontal cortex, and cingulate gyrus.
Three studies have examined the effect of multiple head injuries as a consequence of boxing using PET. In the first study, published by Turjanski et al. in 1997 (Turjanski et al. 1997), six patients (five boxers and one jockey), who were presumed to have posttraumatic parkinsonism, were investigated with 18F-DOPA PET. Results were compared with a healthy group and a group of patients with idiopathic Parkinson’s disease without history of head trauma. In the posttraumatic group, a 40 % reduction of mean 18F-DOPA uptake was found in the caudate and putamen compared with controls. Their mean putamen uptake was significantly higher than in the Parkinson’s disease group, while mean caudate uptake was lower (Fig. 50.4). The authors concluded that – although posttraumatic parkinsonism shares clinical features with idiopathic Parkinson’s disease – the uniform loss of nigrostriatal dopaminergic function in the posttraumatic subject suggests a different underlying pathology.
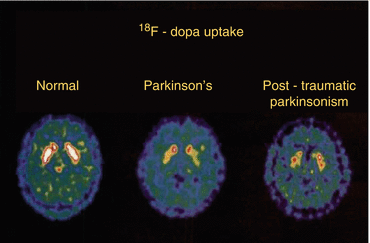
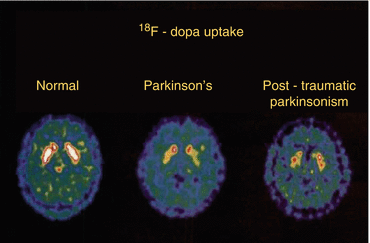
Fig. 50.4
Dopaminergic function in posttraumatic parkinsonism. Transaxial images of striatal 18F-dopa activity accumulated over the last 60 min of the study in a control subject, a patient with Parkinson’s disease, and a patient with posttraumatic parkinsonism. Note that putamen uptake is impaired in both patients, while the posttraumatic parkinsonism patient also shows reduced uptake in the caudate (Turjanski et al. 1997)
It was not until recently that new PET imaging research was performed in athletes: Provenzano et al. (2010) have examined with FDG the effects of repetitive head injury in a group of 17 boxers compared with those of seven controls. Images were analyzed using both SPM and regions of interest. Both methods showed that boxers have bilateral hypometabolism in the posterior cingulate cortex, parieto-occipital cortex, frontal lobes (Broca’s area) bilaterally, and the cerebellum as compared with controls (Fig. 50.5). While these results present a unique pattern of decreased metabolism, the authors suggest that this could be the result of a singular signature of brain injury in boxing. These results partially overlap with the findings of hypometabolism in the cerebellum, vermis, pons, and medial temporal lobe after repeated blast exposure in Iraq war veterans (Peskind et al. 2011). Besides, the reported hypometabolism in the parieto-occipital region interestingly matches with the finding often seen in whiplash injury (Otte et al. 1995; Otte 2012).
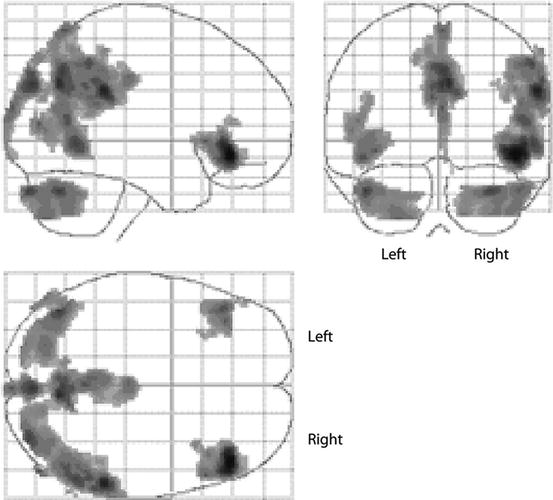
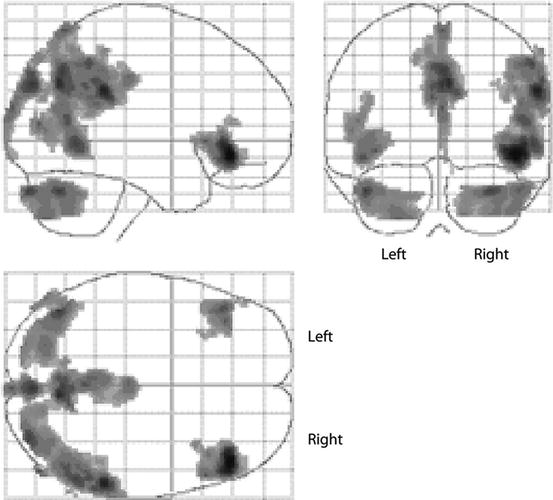
Fig. 50.5
FDG PET imaging of chronic traumatic brain injury in boxers. Statistical parametric mapping analysis showing the group differences between boxers and controls. Regions of decreased FDG uptake displayed on a glass brain are seen in the posterior cingulate cortex and the cerebellum, parieto-occipital, and frontal lobes (Broca’s area) bilaterally (shaded areas) (Provenzano et al. 2010)
The last published study was by Bhidayasiri et al. (2012). They compared dopaminergic function of three retired Thai boxers with parkinsonism with another three patients with idiopathic Parkinson’s disease, having no history of significant head injury. In the posttraumatic parkinsonism patients, a higher uptake in putamen was found compared with the Parkinson’s disease group. Furthermore, boxers had a significantly lower uptake in the ipsilateral anterior putamen and the contralateral posterior putamen than the Parkinson’s disease group (considering the side of predominant symptoms). However, no differences were observed in the caudate nucleus of the two groups. These different findings compared with the previous study of Turjanski et al., where lower uptake was found in the boxers’ group compared to the Parkinson’s disease group, were explained by the shorter disease duration of the posttraumatic parkinsonism in the Thai boxers (range 1–3 years compared to 6 years in the previous study). While both studies support the idea that cumulative chronic head trauma in boxers is an additional insult to the dopaminergic system, more research needs to be done in this context.
50.4.3 PET: Discussion and Future Perspective
The number of PET studies focusing on the consequences, at short or long term, of concussion in sports is very limited. Most of the research is reduced to the well-established FDG scans. However, the potential to investigate specific cellular processes involved in the pathophysiology of a disease is one of the main advantages of the PET technique and should be used in the future to achieve a better understanding of mTBI, particularly in sports, where people are at high risk to receive repeated concussions. In the last years several papers of interest have been published using different PET radiotracers for TBI, which can be considered as the starting point for its application also in the study of concussion in sports, i.e., mTBI.
One of these tracers is 11C-flumazenil (FMZ), a marker for central benzodiazepine receptor expression. The binding of FMZ is used as an indicator of neuronal viability (Heiss et al. 1998). In the study of Shiga et al. (2006), the existence of lesions showing low uptake of FMZ was confirmed. This damage was always paired with abnormalities on the cerebral metabolic rate of oxygen, measured with 15O-labeled gas ([15O]CO, [15O]CO2, and [15O]O2) PET scan, without showing abnormal MRI scans. Areas with alterations in the cerebral metabolic rate of oxygen were also found without relation to FMZ. This suggests that FMZ PET could be useful to differentiate the hypometabolism caused by neuronal loss from the hypometabolism produced by other factors. In a more recent study significant bilateral reduction of FMZ uptake was found in the frontal medial gyrus, anterior cingulate gyrus, and the thalamus (Kawai et al. 2010). While these results are promising for the detection of selective neuronal loss, still more research is needed.
Due to the characteristics of symptoms in chronic stages of PCS and CTE, another interesting cell process to research about is the cholinergic system. A recent study has used [methyl-11C] N-methylpiperidyl-4-acetate (11C-MP4A) in patients with cognitive deficits in a chronic TBI stage (Östberg et al. 2011). 11C-MP4A uptake, which reflects the activity of acetylcholinesterase, was shown to be reduced in several areas of the neocortex, more pronounced in the parieto-occipital region. It would be interesting to study athletes exposed to repetitive concussions to evaluate if cholinergic dysfunction correlates with the clinical symptoms of the patients.
Probably one of the most important cellular processes taking place in the pathophysiology of TBI and concussion is neuroinflammation. Microglia and astrocytes react in the acute phases of trauma and can become chronically activated. The role of activated microglia is crucial in the neuroinflammatory cascade after trauma and therefore was suggested as a sensitive biomarker for neuroinflammation detected with nuclear neuroimaging techniques (Cagnin et al. 2007). The most frequently used radioligand for this purpose is (R)-11C-1-[2-chlorophenyl]-N-methyl-N-[1-methylpropyl]-3-isoquinoline carboxamide (11C-PK11195). In recent studies with chronic TBI patients (up to 17 years after the initial trauma), significant whole brain increase of 11C-PK11195 binding was found bilaterally in the frontal lobe, thalamus, left parietal lobe, right frontal lobe, hippocampus, putamen, midbrain, and pons (Folkersma et al. 2011). In another similar experiment, significant increases were located in the thalamus, putamen, occipital cortex, and posterior limb of the internal capsule (Fig. 50.6) (Ramlackhansingh et al. 2011). Based on the results of these studies, it seems that the use of microglia markers, such as 11C-PK11195 or similar radioligands, could be an attractive method for detecting secondary damage after head trauma and serve as an important marker to evaluate interventions toward inflammatory processes.
