Fig. 62.1
(a) Example of invasive eye fixation by applying two sutures in the rectus muscles and attaching these sutures to the Leksell stereotactic frame. (b) Example of noninvasive eye fixation used for image-guided/gated SRT (From Petersch B, Bogner J, Dieckmann K, et al. Automatic real-time surveillance of eye position and gating for stereotactic radiotherapy of uveal melanoma. Med Phys 2004; 31:3521–3527. Used with permission)
Treatment Planning
A prerequisite for correct treatment planning is good imaging of the target and surrounding structures, especially organs at risk. A typical imaging modality for ophthalmic lesions is MRI, which can be supplemented by computed tomography (CT). Generally, MRI brings better contrast compared with CT when imaging intracranial and intraocular structures. However, whereas CT is supposed to be free of geometric distortions, MRI may produce some geometric image distortions (sometimes a few millimeters) that are not acceptable for SRS or SRT treatment planning. Generally, MRI geometric distortion is mainly a function of the MRI scanner employed, the MRI sequence used, image slice orientation, position in imaged object, and material and geometric parameters of the stereotactic frame [13, 14]. The issue of image geometric distortion is even more crucial in the case of eye imaging when a target and other structures of interest are in a very frontal location. It is essential to check the extent of geometric distortions for each MRI scanner and sequence employed for eye imaging.
Leksell Gamma Knife
Treatment planning for intraocular targets is very similar as for intracranial targets in the case of the Leksell Gamma Knife. Four different collimator helmets (4, 8, 14, 18 mm) are available to create isocenters of essentially spherical dose distributions. To cover optimally the target volume, a combination of all four collimator helmets can be used. Dose distribution can be tailored to the target volume by means of proper isocenter size selection, proper position in the stereotactic space, and proper weighting factor for each isocenter. To protect surrounding healthy tissue and mainly structures at risk, additional shaping of dose distribution can be done by blocking any of the 201 Cobalt-60 beams. Shielding of properly selected number of beams can effectively reduce dose to critical structures such as optic nerve or eye lens. The target volume is usually covered by 50 % isodose line. Treatment planning process is also a compromise between sufficient conformity required for the treated target and treatment time (with increased number of isocenters, total treatment time is increased).
Because of the very frontal location of eye volume, some mechanical difficulties can occur when treating a patient with the Leksell Gamma Knife. Depending on a patient’s head shape and volume, it might be difficult to adjust properly the frame position to reach all Leksell stereotactic coordinates. Specifically, y (posterior–anterior) Leksell stereotactic coordinate can sometimes exceed limits engraved on the Leksell stereotactic frame. Because of this, a special y coordinate extender was developed at Na Homolce Hospital to be able to treat also y coordinates exceeding the limits of the commercially available Leksell stereotactic frame (Fig. 62.2).
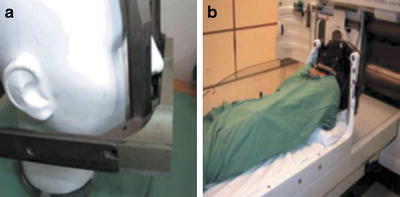
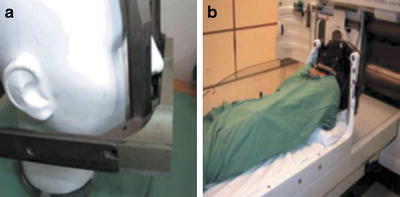
Fig. 62.2
(a) Special y coordinate extender developed at Na Homolce Hospital used for the treatment of eye lesion when y coordinate exceeds the limits of the commercially available Leksell stereotactic frame. (b) Typical treatment of eye lesion on the Leksell Gamma Knife with patient in the prone position
Treatment of eye lesions with the Leksell Gamma Knife requires typically an extremely large neck rake (when supine position is used) that can be hardly tolerated or even impossible to perform for some patients. Consequently, for a patient’s better comfort, the treatment is typically done in the prone patient position (Fig. 62.2).
The most up-to-date system called the LGK PERFEXION was introduced in 2006 [15]. The entire radiation unit was redesigned with a beam geometry different from that of the previous Gamma Knife Models U, B, C, and 4C. A total of 192 Co-60 sources are arranged in a cylindrical configuration in five concentric rings. This differs substantially from the previous hemispherical arrangements and results in a different source-to-focus distance for each ring that varies from 374 to 433 mm. The primary and secondary collimators are replaced by a single large 120-mm-thick tungsten collimator array ring, which eliminates the need for changing different collimator helmets and allows greater flexibility in treatment planning by mixing collimator sizes with each dose of radiation. There are greater time savings in treatment length and improved safety features for patients and practitioners. Improved helmet clearance allows for fewer modifications to treat eccentrically located orbital lesions.
Linear Accelerator
The LINAC is now the most frequently used device for delivery of conventional radiotherapy and radiosurgery. Linear accelerators use electromagnetic waves to accelerate a stream of electrons to nearly the speed of light and then hit a high-density metal target. A small portion of the energy is converted to X-rays through the process of braking radiation and this produces a poly-energetic beam of photons (X-rays) with energies in the MV range. The treatment plans can be individualized for each patient by adjusting the variables of a multiple arc plan. These variables include the number of arcs, the gantry start and end angles of each arc (amplitude), the couch angle (the separation between arcs), different beam energies (arc weighting), and different sized collimators (5–50 mm).
Non-coplanar arcs represent the standard treatment technique. Non-coplanar arcs treatments use circular collimators with fixed diameters. The resulting isodose distribution is essentially spherical and very similar to the one created in the case of the Leksell Gamma Knife. There is usually a wide range (typically 5–40 mm) of circular collimators depending on the manufacturer of the system. Individual dose distribution can be further optimized by combination of proper number and size of circular collimators. However, total number of isocenters is much smaller compared with the Leksell Gamma Knife (usually does not exceed 5).
Another option is non–coplanar conformal static fields. Non-coplanar conformal static fields can be applied using customized blocks or miniature-multileaf collimator with leaf width in the millimeter range. The use of miniature-multileaf collimator has several advantages compared with customized blocks: (1) generating miniature-multileaf collimator shapes takes less time than molding blocks, (2) there is no need to reenter the treatment room during multiple field treatments, and (3) any modification in the shape can be performed easily. Single-isocenter technique with selected number of conformal fields (typically 5–15) is used. Individual fields are shaped using beam’s-eye view technique. Weighting factors of single individual beams, gantry angles, and table angles are used for final dose distribution optimization.
Dynamic non–coplanar arcs are another treatment technique. A selected number of conformal isocentric arcs are used. Beam’s-eye view technique is used for individual beam shaping.
Finally, intensity–modulated SRS or SRT is an option for the treatment planning. A selected number of fields optimized by proper weighting factor, gantry, and table angles are used as in the non-coplanar conformal static fields technique. Inverse treatment planning is used to generate optimal intensity through individual beams.
Georg et al. compared different dosimetric characteristics of treatment plans when using the above-mentioned techniques for the treatment planning of uveal melanomas [16]. They concluded that static conformal and dynamic as treatment techniques that have dosimetric advantages over conventional non-coplanar arcs using circular collimators while being simultaneously highly efficient in treatment planning and delivery. Dynamic arc SRS or SRT combines the homogeneous dose distribution and the high degree of static conformal beams with the steep dose gradients and smeared-out low-dose volumes of arc beam therapy. Intensity-modulated SRS or SRT does not show clear impact on further reduction of doses to organs at risk and depends probably on the target volume location within the eye.
Dosimetry
Because of very eccentric target volume location, some inaccuracies in the treatment planning calculations including relative dose distributions as well as absolute dose calculations (calculation of treatment time or monitor units) can be expected. This issue is even more important for surface structures of eye (e.g., eye lens, eye lid, cornea) than for target volumes itself as these structures are located in depths smaller than buildup of employed photon energies.
Petersch et al. reported excellent agreement between measurements and treatment planning system calculations for uveal melanomas at depth 15–20 mm [8]. However, at small depths (<10 mm), the treatment planning system cannot model the influence of absorption in eye motion monitoring device accurately if beam passed perpendicularly through it. Consequently, Petersch et al. suggested avoidance of frontal beams when doing treatment planning as a solution to reduce significantly dosimetric errors.
Detailed dosimetric measurements including the treatment planning system calculations of target volume relative dose distributions and absolute dose calculations in different depths were compared with experimental measurements. Various treatment plans for different ophthalmic indications (uveal melanoma, glaucoma, retinoblastoma) calculated for the Leksell Gamma Knife were evaluated. There was observed good agreement between treatment planning system relative dose distribution calculations for target volume and performed measurements (Fig. 62.3). For the calculation of absolute doses, there was observed very good agreement at depths 15–20 mm. Typical deviations between treatment planning system calculations and actual measurements were within 3 %. However, measurements performed at depth less than 10 mm showed large deviations of about 15–20 % compared with the treatment planning system calculations. The dose delivered to patient was always smaller than that calculated by the treatment planning system. To improve inaccuracies in absolute dose calculations in surface structures, it was suggested to use proper tissue equivalent built-up material that is attached on the treated eye (Fig. 62.3) and improves treatment planning system calculations.
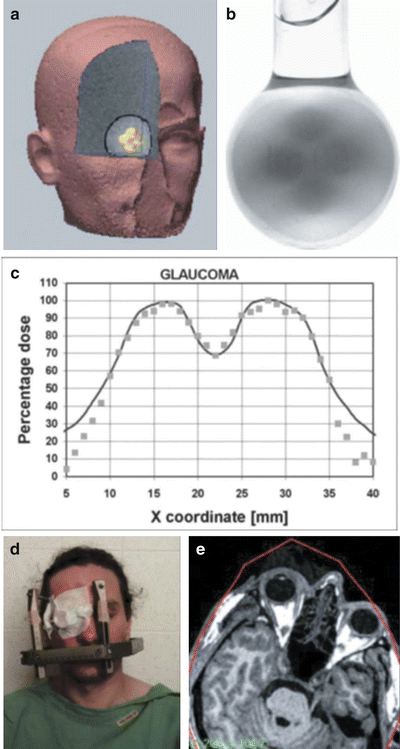
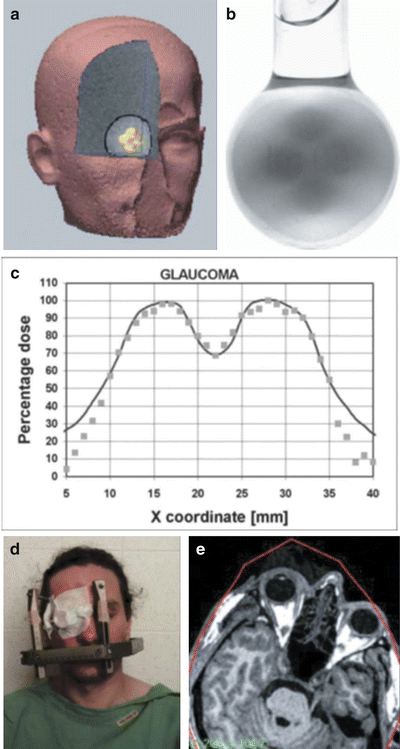
Fig. 62.3
(a–c) Example of relative dose distribution treatment planning system calculations verification with polymer gel dosimeter. (a) Three-dimensional treatment plan in LGP. (b) Polymerization that occurred in the polymer gel. (c) Relative dose profiles (calculated by LGP—solid line, measured with polymer gel—dotted line). (d) Tissue-equivalent built-up material that is attached to the treated eye to improve inaccuracies in absolute dose calculations in surface structures. (e) Corresponding MR localization scan visualized in LGP together with head contour
Clinical Application
Stereotactic radiosurgery or radiotherapy currently plays an important role as primary treatment, adjuvant therapy, or palliation of ocular and orbital tumors. The clinical situation, radiation source, and desired result determine the prescribed radiation dose. The following reviews the published experience with stereotactic radiosurgery or radiotherapy for ocular and orbital lesions (Table 62.1) [3, 4, 17–41].
Table 62.1
Indications and radiotherapy doses for ocular and orbital disease
Pathology | Orbital radiation doses (Gy) |
---|---|
Average or recommended dose (Range) | |
Tumor | |
35 (35–50) | |
Retinoblastoma [35] | 43 (40–50) |
40 (30–40) | |
Lymphoma [39] | 32 (15–46) |
45 (45–54) | |
Optic nerve glioma [56] | 50 (42–54) |
Basal cell carcinoma [57] | 50 (34–50) |
50 (50–64) | |
Sebaceous carcinoma [60] | 55 (45–63) |
45 (32–72) | |
Vascular | |
14.5 (12.5–40) | |
Choroidal haemangiomas [42] | 10 (10) |
Uveal angiomas (Sturge Weber) [63] | 12 (12–30) |
Capillary hemangioma—orbit [43] | 4.5 (1.5–7.5) |
Others | |
12 (12–20) | |
Glaucoma | 15 (7–15) |
Uveal Melanomas
Pathophysiology
Uveal melanoma represents the most common primary malignant tumor of the eye in adults with a peak incidence between ages 55 and 70 years. It affects 6–7 people per million each year, and approximately 50 % of patients with diagnosed melanomas of the chorioid or ciliary body will die from this tumor within 15 years [17]. The incidence is rare in patients younger than 20 years. The incidence of cutaneous melanomas has been increasing in frequency over the past several decades and the dependence on latitude has been observed, reflecting levels of exposure to ultraviolet light. This trend has not been evident for patients with uveal melanomas. Uveal melanomas are neoplasm arising from the uveal tract, which includes the iris, ciliary body, and chorioid. Two types of tumor growth were recognized, nodular and infiltrative. Histologically, the tumor consists of spindle cells, epithelioid cells, and intermediate cells similar to those of cutaneous melanoma, but the prognosis is better than that associated with cutaneous melanomas.
The uveal tract is a vascular structure with no lymphatic drainage. Lymphatic node involvement (auricular, submandibular, and cervical nodes) can be diagnosed on rare occasions when subconjunctival extension of primary tumor has been observed. The major routes of spread are local growth and by bloodstream, with a predilection for hematogenous dissemination to the liver, lung, and brain. Liver metastases are diagnosed in two-thirds of patients with disseminated diseases. Systemic examination is recommended for all patients and includes CT of the chest and abdomen, and MRI of the brain to detect metastases. Wide variations in the grading of malignancy can be observed, from relatively benign types with several years’ survival without dissemination, to other tumors with fast multiorgan dissemination, which lead to the patient’s death within a few months.
Tumor Location
The iris melanomas (incidence about 10 %) are single or multiple elevated lesions with changes of iris color. These types of melanomas are much more benign, smaller in size, readily visible, and early diagnosed. It can be difficult to assess the potential of the malignant cells, even in those with documented growth, because iris nevi may also grow. For this reason, local resection is usually effective whenever it seems to be necessary.
Choroidal melanomas typically grow in nodular form, and in cases of continuing growth many tumors break through the Bruch membrane. The Bruch membrane is a basement membrane–connective tissue complex lying between the retina pigment epithelium and the choriocapillaries. When the melanomas break through the Bruch membrane, they extend into the subsensory retina space, which gives them a mushroom or collar-button shape. These characteristics, as well as the detachment of the retina, are typical for uveal melanomas. This type of tumor growth is sometimes accompanied by vitreous hemorrhage with symptoms of sudden visual loss.
Melanomas involving the ciliary body are rare, carry a poor prognosis, and are often diagnosed late. Melanomas can also have diffuse, infiltrative growth to the ciliary body and a pattern called ring melanoma. These tumors are diagnosed with difficulty and late, because they are accompanied by relatively little visible tumor mass effect and they occur in the diagnostically “silent” eye area. Any extra scleral extension of the tumor usually confers a poor prognosis. The diffuse growing melanomas are usually more aggressive, with more malignant cell types.
Tumor growth is also influenced on the potential doubling time (T pot) which varies widely between 60 and 350 days (the median of T pot being about 70 days) [18]. A longer T pot for a significant proportion of tumors can explain the relatively long survival of these patients and also the relatively slow tumor response after irradiation.
Primary Tumor Diagnosis
Melanoma of the ciliary body and chorioid have typical clinical features, and the correct diagnosis for a majority of patients can be made by taking a history and performing a complete ocular examination. The ophthalmologic examination includes external ocular examination, indirect ophthalmoscopy, fluorescein angiography, and ultrasonography A, B. Magnetic resonance imaging plays a fundamental role in the treatment planning of radiosurgery or stereotactic radiotherapy.
Ultrasonography plays a basic role, not only at the time of diagnosis but during follow-up and is used to obtain precise measurement of the tumor base and height. The height of tumor in particular represents one of the most important factors influencing the treatment decision, and it is used during follow-up to evaluate the tumor response.
Several staging systems have been used, one of the most commonly reported was based on the 2010 American Joint Staging System (Tables 62.2 and 62.3) [19]
Primary tumor (T) | |
TX | Primary tumor cannot be assessed |
T0 | No evidence of primary tumor |
Iris | |
T1 | Tumor limited to the iris |
T1a | Tumor limited to the iris not more than 3 clock hours in size |
T1b | Tumor limited to the iris more than 3 clock hours in size |
T1c | Tumor limited to the iris with secondary glaucoma |
T2 | Tumor confluent with or extending into the ciliary body, choroid, or both |
T2a | T2 with secondary glaucoma |
T3 | Tumor confluent with or extending into the ciliary body, choroid, or both, with scleral extension |
T3a | T3 and secondary glaucoma |
T4 | Tumor with extrascleral extension |
T4a | Tumor with extrascleral extension ≤5 mm in diameter |
T4b | Tumor with extrascleral extension >5 mm in diameter |
Ciliary body and choroid | |
T1 | Tumor size category 1 |
T1a | Tumor size category 1 without ciliary body involvement and extraocular extension |
T1b | Tumor size category 1 with ciliary body involvement |
T1c | Tumor size category 1 without ciliary body involvement but with extraocular extension ≤5 mm in diameter |
T1d | Tumor size category 1 with ciliary body involvement and extraocular extension ≤5 mm in diameter |
T2 | Tumor size category 2 |
T2a | Tumor size category 2 without ciliary body involvement and extraocular extension |
T2b | Tumor size category 2 with ciliary body involvement |
T2c | Tumor size category 2 without ciliary body involvement but with extraocular extension ≤5 mm in diameter |
T2d | Tumor size category 2 with ciliary body involvement and extraocular extension ≤5 mm in diameter |
T3 | Tumor size category 3 |
T3a | Tumor size category 3 without ciliary body involvement and extraocular extension |
T3b | Tumor size category 3 with ciliary body involvement |
T3c | Tumor size category 3 without ciliary body involvement but with extraocular extension ≤5 mm in diameter |
T3d | Tumor size category 3 with ciliary body involvement and extraocular extension ≤5 mm in diameter |
T4 | Tumor size category 4 |
T4a | Tumor size category 4 without ciliary body involvement and extraocular extension |
T4b | Tumor size category 4 with ciliary body involvement |
T4c | Tumor size category 4 without ciliary body involvement but with extraocular extension ≤5 mm in diameter |
T4d | Tumor size category 4 with ciliary body involvement and extraocular extension ≤5 mm in diameter |
T4e | Any tumor size category with extraocular extension >5 mm in diameter |
Regional lymph nodes (N) | |
NX | Regional lymph nodes cannot be assessed |
N0 | No regional lymph node metastasis |
N1 | Regional lymph node metastasis |
Distant metastasis (M) | |
M0 | No distant metastasis |
M1 | Distant metastasis |
M1a | Largest diameter of the largest metastasis ≤3 cm |
M1b | Largest diameter of the largest metastasis 3.1–8.0 cm |
M1c | Largest diameter of the largest metastasis ≥8 cm |
Table 62.3
Anatomic stage/prognostic groups of uveal melanoma (American Joint Staging System, 2010) [19]
Stage | T | N | M |
---|---|---|---|
I | T1a | N0 | M0 |
IIA | T1b–d | N0 | M0 |
T2a | N0 | M0 | |
IIB | T2b | N0 | M0 |
T3a | N0 | M0 | |
IIIA | T2c–d | N0 | M0 |
T3b–c | N0 | M0 | |
T4a | N0 | M0 | |
IIIB | T3d | N0 | M0 |
T4b–c | N0 | M0 | |
IIIC | T4d–e | N0 | M0 |
IV | Any T | N1 | M0 |
Any T | Any N | M1a–c |
Treatment Methods
Treatment modalities strongly depend on the site of the primary tumor and its volume and metastatic extent. Historically, the first known treatment modality was enucleation [20].
Surgical Treatment
Surgical methods include resection of the eye wall (for very small visible melanomas of the iris), enucleation, and orbital exenteration [20]. The role of enucleation in the management of posterior to equator localized melanomas is controversial, but enucleation remains the standard option for most large choroidal melanomas that cause severe secondary glaucoma or are invading the optic nerve.
Radiotherapy
Preoperative or postoperative irradiation is a promising approach under evaluation that could also decrease the chances of malignant dissemination, however recent study observed no benefit [21]. Postoperative external beam radiotherapy has been used in patients with large tumors with extrascleral or extraocular extension. The recommended total applied dose is 60 Gy with daily fractionation.
Brachytherapy
The most frequently used therapeutic system for delivering radiation is brachytherapy (plaque radiotherapy). A number of radionuclides, including cobalt-60, gold-198, iodine-125, ruthenium-106, iridium-192, and palladium-103, have been used for plaque therapy with varying results according to the retrospective studies [22, 23]. A metal shield containing small radioactive seeds is sutured to the outside of the sclera overlying the tumor with radiation doses principally delivered to the base of the tumor. The radioactive plaque is left in place for approximately 5–7 days. Cobalt-60 was one of the first isotopes to be used in brachytherapy, but it is a high-energy gamma emitter and thus there exists a risk of damaging healthy ocular structures several millimeters from the source. Other isotopes have been used: iodine-125 is also a gamma emitter but has lower energy than cobalt-60 and, because of this, it is associated with a lower rate of complications. Brachytherapy leads to long-term local tumor control in 85–90 % of patients and can be used to treat small melanomas [22, 23]. The best results can be achieved in tumors less than 10 mm in height and with a base diameter not exceeding 16 mm. Patients with extrascleral tumor extension, ring melanoma, and tumor involvement of more than half of the eye are not suitable for plaque therapy.
Proton Beam Therapy
Patients with tumors too large for brachytherapy or tumors considered too close to the optic nerve have been recommended for proton beam therapy (PBT). Early studies with PBT recognized that severe damage was caused to the eyelid and the anterior parts of the eye. Patients with tumors localized near the eye surface had a higher incidence of severe toxicity because the sparing effect of Bragg peak was absent. Many of these patients had chronically painful and cosmetically unsatisfactory eyes. Local tumor control in 90–98 % of patients was achieved by this method in a large series [24]. The technique was subsequently improved and the complication rate has dropped accordingly.
Charged Particle Therapy
Helium ion irradiation is uniquely suited to the precise localization of irradiation because of the sharp falloff of the dose at the distal end of the Bragg peak, the sharp lateral edges of the beam, and the ability to tailor the depth penetration and spread by a modulated Bragg peak. Uveal melanomas are indicated for this treatment. When the charged particles are used to treat the uveal melanoma, the normal tissue of the anterior part of the eye can receive a radiation dose comparable with the tumor dose. This fact causes radiation toxicity in the anterior parts of the eye leading to side effects such as eyelash loss, lacrimal damage, conjunctival changes, dry eye syndrome, keratitis, and neovascular glaucoma [25, 26]. Local tumor control is achieved in 96 % of patients and the enucleation rate is 19 % (3 % for local failure and 16 % due to complications) [26].
Stereotactic Radiotherapy
The advantage of stereotactic irradiation using a linear accelerator is the possibility of fractionated treatments compared with the Leksell Gamma Knife. Fractionation may decrease late-radiation-related toxicity, and this fact was the subject of intensive study [8, 9, 11, 16, 27–29]. The reported mean applied minimal dose ranged from 25 to 45 Gy in two fractions and 15 Gy in three equal fractions. Local tumor control, defined as freedom of local progression, was reported in 98 % of patients [29]. Further clinical studies using fractionated stereotactic irradiation are justified to optimize the effective dose and fractionation pattern.
Radiosurgery
The first experiments with radiosurgery for the treatment of uveal melanomas were conducted using the rabbit eye melanoma model, with reported tumor lethal dose range from 60 to 90 Gy applied in a single session. A preliminary study of 11 treated patients with uveal melanomas was published in 1992 and suggested that local tumor control could be achieved with a minimal dose between 60 and 90 Gy in a single dose using the Leksell Gamma Knife. Some observations were made on patients after radiosurgery who had their eyes secondarily enucleated. All eyes with secondary enucleations presented an initial height exceeding 9 mm and also had a large number of ciliary body tumors [30]. Several authors reported the results of radiosurgery in uveal melanomas and some of them observed relatively high radiation-related toxicity [4, 30, 31].
Dinca et al. reported the UK experience in treating ocular melanoma over the last 20 years [3]. One hundred seventy patients who were treated with Gamma Knife radiosurgery were compared with 620 patients treated with eye enucleation. Different peripheral doses (at the 50 % isodose) were employed. There was no significant difference in survival between the 35-Gy, 45-Gy, and 50–70-Gy groups when compared between themselves (p = 0.168) and with the enucleation group (p = 0.454). The 5-year survival rates were: 64 % for 35 Gy, 62.71 % for 45 Gy, 63.6 % for 50–70 Gy, and 65.2 % for enucleated patients. Clinical variables influencing survival for radiosurgery patients were tumor volume (p = 0.014) and location (median 66.4 months for juxtapapillary vs. 37.36 months for peripheral tumors, (p = 0.001). Regarding complications, using 35 Gy led to more than a 50 % decrease in the incidence of cataract, glaucoma and retinal detachment, compared with the 45-Gy dose. Retinopathy, optic neuropathy, and vitreous hemorrhage were not significantly influenced. Blindness decreased dramatically from 83.7 % for 45 Gy to 31.4 % for 35 Gy (p = 0.006), as well as post-radiosurgery enucleation: 23.9 % for 45 Gy vs. 6.45 % for 35 Gy (p = 0.018). Visual acuity, recorded up to 5 years post-radiosurgery, was significantly better preserved for 35 Gy than for 45 Gy (p = 0.0003). It appears that using 35 Gy results in a dramatic decrease in complications, visual loss, and salvage enucleation, while not compromising patient survival.
Sarici et al. reported on 50 eyes from 50 consecutive patients with uveal melanoma that were treated with GKRS and had a median follow-up time of 40 months. They used a dose of 30 Gy, which is an eye-sparing outpatient option for patients with medium- or large-sized posterior uveal melanomas who are not eligible for brachytherapy or particle therapy. The tumor control rate was 90 %, and the eye retention rate was 82 %. Metastases developed in 18 % of patients with 14 % dying of metastases during follow-up. Cataracts (34 %) and radiation maculopathy (30 %) were the most frequent complications, and 14 % of patients developed neovascular glaucoma. Visual acuity (VA) also decreased significantly after treatment (p < 0.0001) [32].
Šimonová, et al. studied a group of 126 patients with uveal melanoma treated using the Leksell Gamma Knife over a period of 8 years with all survivors undergoing minimal follow-up 24 months after the treatment. The late effect of normal tissue (LENT) subjective objective management analysis (SOMA) scoring system is acceptable for measurement of radiation side effects (Table 62.4).
Table 62.4
Toxicity scoring system (RTOG/EORTC LENT SOMA)
Grade 1 | Grade 2 | Grade 3 | Grade 4 | |
---|---|---|---|---|
Cornea | Increased tearing on exam | Noninfectious keratitis | Infectious keratitis, corneal ulcer | Panophthalmitis, corneal scar, ulceration leading to perforation of globe/loss of globe |
Iris | Rubeosis only | Rubeosis, increased intraocular pressure | Neovascular glaucoma, ability to count fingers at 1 m | Neovascular glaucoma without ability to count fingers at 1 m; complete blindness |
Optic nerve | Afferent pupillary defect with normal-appearing nerve | ≤¼ pallor with asymptomatic visual field defect | >¼ pallor or central scotoma | Profound optic atrophy, complete blindness |
Lens | Asymmetric lenticular opacities, no visual loss | Moderate lenticular changes with mild-moderate visual loss | Moderate lenticular changes with several visual loss | Severe lenticular changes |
Retina | Microaneurysms, nonfoveal exudates, minor vessel attenuation, extrafoveal pigment changes | Cotton wool spots | Massive macular exudation, focal retinal detachment | Opaque vitreous hemorrhage, complete retinal detachment, blindness |
Sclera | Loss of episcleral vessels | ≤50 % scleral thinning | >50 % scleral thinning | Scleral or periosteal graft required due to perforation
![]() Stay updated, free articles. Join our Telegram channel![]() Full access? Get Clinical Tree![]() ![]() ![]() |