Fig. 1
Studies published by year involving schizophrenia and MEG. A search of pubmed using the terms (“magnetoencephalography”, “MEG”, “neuromagnetism”, OR “neuromagnetic”) AND (“schizophrenia”, “schizoaffective disorder”, “hallucinations” OR “psychosis”) was performed (line labeled MEG + Schizophrenia). This was compared to all schizophrenia publications over the same time period
3 Evoked Magnetic Fields in Schizophrenia
3.1 Cerebral Lateralization of the Location of Auditory and Somatosensory Evoked Fields
Some of the earliest studies employing MEG in schizophrenia focused on an asymmetry between the left and right hemisphere location of the auditory components of the evoked response. The M100 (also termed the N100 m) is generated within the auditory cortices on the supratemporal plane (Pantev et al. 1998; Reite et al. 1994) and its location is generally relatively more anterior in the right compared to the left hemisphere (Nakasato et al. 1995; Mäkelä et al. 2004). Reite et al. (1989) reported in a preliminary study of 6 male patients and 6 controls that the schizophrenia group exhibited reduced interhemispheric asymmetry compared to controls. Although the original study used repeated measurements of a single-channel MEG instrument, the finding has been replicated using larger array devices, both by the original research group and independently by other investigators using different MEG devices (Tiihonen et al. 1998; Rojas et al. 2002; Rockstroh et al. 2001; Edgar et al. 2006; Reite et al. 1997). Reduced M100 asymmetry has been compared directly between patients with schizophrenia and those with schizoaffective disorder, with both patient groups exhibiting reduced asymmetry compared to controls, but these groups were not significantly different from each other (Teale et al. 2000).
Two studies have compared reduced M100 location asymmetry in schizophrenia directly to samples of persons with dyslexia, a developmental disorder defined by reading disability (Edgar et al. 2006; Heim et al. 2004). In both studies, reduced anterior-posterior asymmetry in both clinical groups was reported relative to control samples, suggesting that the asymmetry reduction might reflect a non-specific neurodevelopmental biomarker. Supportive of this interpretation, reduced asymmetry has also been observed in other neurodevelopmental disorders, including Fragile X syndrome (Rojas et al. 2001) and autism (Rojas et al. 2008; Schmidt et al. 2009).
It has also been suggested that the reduced asymmetry in schizophrenia may be a finding specific to males (Reite et al. 1997; Rojas et al. 1997). However, other investigators examining gender differences have not observed changes in location in schizophrenia, but have noted gender specific alterations in M100 dipole orientation instead (Hajek et al. 1997a, b). Gender differences, if any, may be important in schizophrenia because of the observation of later onset and possibly less severe psychopathology in women with the disorder (Goldstein 1988; Aleman et al. 2003).
The abnormality in asymmetry is not limited to source locations based on the M100, or even to auditory evoked field locations. The very first schizophrenia paper published suggested reduced asymmetry of the location of the auditory M50 source (Reite et al. 1988), and more recently there has been evidence published that the auditory-steady state source also exhibits this phenomenon in schizophrenia patients (Teale et al. 2003). Outside of the auditory cortex, reduced location asymmetry of the somatosensory M20 and M50 components have been described in schizoaffective disorder and schizophrenia, respectively (Reite et al. 1999b, 2003).
Reduced laterality of the location of auditory and somatosensory responses has also been examined in two studies of early onset psychotic disorders including schizophrenia. Wilson et al. (2008) did not find significant differences in lateralization of the auditory steady state response in children and adolescents with a heterogeneous sample of various early onset psychoses. In a separate study, however, somatosensory M50 dipole location asymmetry was found to be reduced in study of children ages 8–16 years with various early onset psychotic disorders including schizophrenia, mood disorders with psychotic features and psychosis NOS (Wilson et al. 2007). In that study, the location asymmetry for the M50 dipole was left more anterior than right hemisphere for both groups, but this left to right shift was reduced in the psychotic group. Although the increased symptomatic heterogeneity in combined psychotic disorder samples may lead to increased variability in sources, a study in bipolar disorder, directly comparing currently euthymic patient samples with and without a history of psychosis, found that reversed somatosensory M20 anterior-posterior localization was specific to the patients with a positive history psychosis, operationally defined as a history of hallucination and/or delusions (Reite et al. 1999a). In contrast to healthy control subjects and non-psychotic bipolar groups, both of which exhibited right hemisphere locations anterior to the left hemisphere locations, the psychotic bipolar group exhibited right hemisphere M20 locations posterior to the ones in the left hemisphere. This suggests that the dimension of psychosis rather than the specificity of the diagnosis is a key factor. The developmental trajectory of location lateralization for auditory and somatosensory cortices has not yet been studied, however, and more evidence is needed to define both the development and the symptom dimension associations. No studies have yet reported significant correlations between various symptoms of schizophrenia/psychosis and location asymmetry. Reduced M100 amplitude asymmetry to monaural stimulation, between the contralateral and ipsilateral responses, has also been reported (Rockstroh et al. 1998).
Evidence of altered cerebral lateralization in location of auditory and somatosensory function from MEG is part of a larger literature on changes in structural and functional lateralization in schizophrenia. For example, a meta-analysis of various aspects of lateralization in the schizophrenia indicates increased odds ratio of non-right-handedness, increased odds of reduced right-ear advantage in dichotic listening to consonant-vowel sounds, and increased odds of reduced asymmetry of brain structure in schizophrenia, especially in temporal lobe/Sylvian fissure regions, compared to controls (Sommer et al. 2001). It should be noted, however, that abnormality of the localization of function does not necessarily imply an underlying structural abnormality. In one study comparing locations of the auditory M100 to the location of Heschl’s gyrus, asymmetry (right anterior to left) in the location of Heschl’s gyrus was observed in both schizophrenia and control samples (Rojas et al. 1997). Although there were no significant differences between groups in anterior-posterior position of the structure, M100 location asymmetry was significantly different between groups (Rojas et al. 1997). MEG information on location of function may be additive, rather than simply a reflection of an underlying anatomical difference.
3.2 MEG Studies of Auditory Processing
Deficient auditory processing is consistently observed in behavioral studies of schizophrenia. Frequency-matching performance, for example, is commonly reported to be worse in subjects with schizophrenia than in comparison groups (Holcomb et al. 1995; Rabinowicz et al. 2000; Javitt et al. 2000). There is an extensive body of EEG and MEG research on impairment of the mismatch negativity (MMN) in schizophrenia (reviewed in Naatanen and Kähkönen 2009). Similar to the widely replicated reduction in MMN observed in schizophrenia patients using EEG, MEG studies of the magnetic analog (variously termed MMNm or MMF), have also revealed smaller mismatch responses in patients (Kircher et al. 2004; Kreitschmann-Andermahr et al. 1999; Pekkonen et al. 2002; Kasai et al. 2003; Jordanov et al. 2011).
Some MEG studies, however, have tended to capitalize more on source localization strategies than EEG studies, and some interesting results have emerged. Kircher et al. (2004) reported that for duration mismatch responses, schizophrenia patients were significantly less right lateralized compared to healthy controls. Pekkonen et al. (2002) found that while patients with schizophrenia had significantly reduced MMNm amplitudes in both hemispheres compared to control subjects, MMNm latency was only significantly delayed in the left hemisphere. Simple dipole analyses of MMNm, however, may not be as likely to succeed for individual patients with schizophrenia as with healthy control subjects, probably due to a reduction in signal strength in the patient group (Yamasue et al. 2004; Ahveninen et al. 2006). To avoid this, one study fixed the dipole location for the MMNm using a priori information on the location of the primary auditory cortex (Thonnessen et al. 2008). In this latter study, MEG and EEG mismatch responses were directly compared using the fixed dipole locations and MEG sources outperformed the EEG sources in terms of significant group differences across a number of experimental manipulations (Thonnessen et al. 2008). In a recent study, Dima et al. (2012) examined connectivity and MMNm in schizophrenia, employing a dynamic causal modeling (Friston et al. 2003) approach to fixed dipole locations within primary auditory, secondary auditory and inferior frontal cortices. Dima et al. (2012) reported an abnormal reversal of connectivity (i.e., reversal of information directional flow) between frontal and superior temporal sources during the MMNm. Whereas healthy individuals exhibited the predicted negative modulation of temporal lobe from frontal lobe (i.e., bottom up rather than top down), schizophrenia patients exhibited greater bottom up modulation from the temporal lobe to frontal lobe.
MMNm studies have also been published concerning risk for schizophrenia and its genetics. Shin et al. (2009) studied 16 individuals at high risk for schizophrenia based on the presence of attenuated symptoms (i.e., the schizophrenia prodrome) and found that MMNm dipole amplitude was reduced in the right hemisphere and latency was prolonged, relative to 18 healthy controls. Ahveninen et al. (2006) examined MMN and MMNm in a twin design, including monozygotic twins discordant for schizophrenia (N = 10 pairs) and dizygotic twins discordant for schizophrenia (N = 13 pairs) as well as control MZ and DZ twin pairs. Although the EEG MMN component was significantly reduced in the schizophrenia patients and their unaffected twins, the MEG MMNm did not exhibit significant differences between groups, in contrast to the more recent study of Thonnessen et al. (2008), described above. Both EEG and MEG responses exhibited genetic influence relative to the degree of relatedness to schizophrenia (Ahveninen et al. 2006).
Aside from the MMNm component, other evoked magnetic components have been studied with respect to sensory representations and processing in schizophrenia. Two studies have examined tonotopy, or the spatial mapping of frequency to the auditory cortex, using the auditory M100 response (Rosburg et al. 2000b; Rojas et al. 2002). Both found evidence of frequency effects on M100 dipole location location for healthy controls, similar to other MEG studies published using only healthy samples (Romani et al. 1982; Pantev et al. 1995). Although both studies also reported differences in the patient group, the specifics differed between studies. Rosburg et al. (2000b) reported frequency-dependent differences in location in the anterior-posterior coordinate, which were slightly greater in patients than controls in the right hemisphere but much greater in controls than in patients in the left hemisphere. In contrast, Rojas et al. (2002) found frequency differences in location on the medial-lateral coordinate for the M100, as well as a reduction in this difference, in both hemispheres, in patients with schizophrenia. Relative to the head coordinate systems used, Heschl’s gyrus, the nominal structural correlate of the primary auditory cortex, has an oblique angle. Frequency gradients along it may be anterior-posterior, medial-lateral, or both, depending on the specific anatomy. Future comparisons may benefit from an accounting of this variability by expressing location coordinates within an anatomically derived framework (Jordanov et al. 2010).
In addition to dipole location, several studies have examined auditory evoked field latency and amplitude in schizophrenia under various experimental manipulations. M100 amplitude is known to exhibit stimulus-specific refractoriness and habituation to repeated stimulation (Hari et al. 1982). Rosburg et al. (2000a) did not find differences in this behavior in 20 patients with schizophrenia when comparing latency and amplitude of the M100 to repeated stimulation over several trial blocks. Overall both controls and schizophrenia patients showed increased latency and decreased amplitude of the M100 as trial blocks increased. There was, however, a significantly higher degree of habituation in a small sub-group of patients taking clozapine, the dose of which correlated with amplitude habituation. As pointed out by the authors, clozapine may be more effective than other antipsychotics at relieving a deficit in rapid habituation termed sensory gating in schizophrenia (Adler et al. 2004). Yet another study used M100 refractory behavior to examine frequency specific tuning of the M100 and found reduced frequency specificity of the habituation in M100 amplitude in schizophrenia patients (Rojas et al. 2007). Additional reported findings indicating an impairment of early auditory processes include earlier M50 responses (Pekkonen et al. 1999) and reduced amplitude of the M100 (Kreitschmann-Andermahr et al. 1999; Rojas et al. 2007; Edgar et al. 2012). Reduced M100 amplitude appears to be associated with thinner underlying auditory cortex, both in persons with schizophrenia (Edgar et al. 2012) and in subjects at high risk for the disorder based on having a first degree relative and/or prodromal symptoms (Shin et al. 2012).
3.3 Sensory Gating
One of the most widely replicated and highly studied EEG evoked potential findings in schizophrenia is the so-called sensory gating deficit (Adler et al. 1982). Normally studied using the EEG auditory P50 response to closely spaced paired clicks, healthy individuals tend to exhibit reduced amplitude to the second click (i.e., gating), while individuals with schizophrenia do not exhibit suppression of the second click response amplitude (Patterson et al. 2008). It is sometimes observed that reduced amplitudes of responses to the first, rather than the second click, explain the usual gating ratio for P50 and M50-based sensory gating impairment in schizophrenia (Blumenfeld and Clementz 2001). Impaired sensory gating has been linked to mutations of the cholinergic alpha-7 receptor (CHRNA7) on chromosome 15 (Freedman et al. 2003).
MEG studies have added useful information to this extensive literature. EEG researchers commonly measure P50 sensory gating at vertex (Cz), referenced to linked mastoids or ears and therefore have no information about lateralization of the response. Thoma et al. (2003) first reported that the sensory gating deficit appeared to be lateralized to auditory M50 sources in the left hemisphere. The left, but not right hemisphere gating in MEG was correlated to the vertex P50 gating response. The lateralized left hemisphere M50 gating deficit correlates with negative symptoms (Thoma et al. 2005), attention and working memory deficits (Thoma et al. 2003; Smith et al. 2010) and long-term memory (Smith et al. 2010). A recent study also extended the MEG sensory gating deficit for the M50 to human voices rather than clicks, finding that the left-lateralized deficit was associated with auditory hallucinations (Hirano et al. 2010). Simulations of changes in dipole location, orientation and interhemispheric latency differences have shown that source configuration is important to consider in sensory gating studies in schizophrenia (Edgar et al. 2003). Indeed, source modeling of the M50 response has also been shown to improve the reliability of sensory gating measures, compared to Cz-only EEG approaches (Lu et al. 2007). An MEG study that did not examine sensory gating per se found that M50 responses had higher signal-to-noise ratios than P50 responses, suggesting another potential advantage to MEG (Thonnessen et al. 2008).
One MEG study examined the proportion of variance in the vertex EEG explained by bilateral auditory dipoles modeled using MEG data. Huang et al. (2003) found that dipoles in the left and right auditory cortices account for approximately 97 % of the variance in healthy individuals observed at a vertex EEG electrode for the time period including the P50 responses, but a smaller amount, 86 %, in persons with schizophrenia. In that study, the residual variance waveform for the dipole had a peak frequency of 40 Hz, suggesting unaccounted for variance in the gamma-band in schizophrenia subjects. Indeed, an early combined EEG and MEG sensory gating paper suggested that the gating effect was stronger for gamma-band signals overlapping the P50/M50 response temporally (Clementz et al. 1997). Other spectrally-focused MEG studies of sensory gating have implicated theta, alpha and beta abnormalities as well (Edgar et al. 2008; Ho et al. 2008; Popov et al. 2011).
Gating is not specific to the auditory M50 response. The M100 response also exhibits reduced amplitude to the second of two paired sounds, although historically this has been considered in the context of refractoriness or habituation (see Sect. 3.2). Hanlon et al. (2005a) reported M100 gating deficits in schizophrenia, in addition to the M50-based deficit. The M100, unlike the M50, showed bilateral deficits in the patients. One MEG study examining M100 suppression effects in schizophrenia found that when using monaural stimulation, instead of the usual binaural stimuli, ipsilateral but not contralateral response gating was worse in patients compared to controls (Blumenfeld and Clementz 1999). Similarly, Dale et al. (2010) found that M100 response suppression to the second of two closely spaced syllables was impaired in schizophrenia. Another study examined the generality of sensory gating deficits across sensory modalities in 27 patients with schizophrenia and 21 control subjects (Edgar et al. 2005). Deficits were replicated for the auditory M50 gating response, but were not present in the somatosensory system using the M20 response to median nerve stimulation. The lack of somatosensory gating deficit in schizophrenia does not imply an intact somatosensory system, however, as another MEG study found abnormalities in evoked responses to median nerve stimulation in schizophrenia in the context of a somatosensory oddball task (Huang et al. 2010). Additionally, a follow-up study of secondary somatosensory cortical responses (the M20 is generated in primary somatosensory cortex) found evidence for gating deficits in these later responses in patients with schizophrenia (Thoma et al. 2007).
As with the M100 response, M50 and gating measures derived from it are correlated with structural changes in the brain in schizophrenia. An early study reported that M50 gating was negatively correlated with anterior hippocampal volumes in a hemisphere-specific manner, such that gating in the left correlated with left hippocampus and gating in the right correlated with right hippocampus (Thoma et al. 2008). This is an important observation given the putative role for the hippocampus in some models of sensory gating and the general lack of imaging evidence for a hippocampal generator. Other experimental paradigms more specific to hippocampal function have revealed hippocampal deficits in schizophrenia using MEG (Hanlon et al. 2005b, 2011). In addition, Thoma et al. (2004) also found that thinner auditory cortex in schizophrenia subjects was associated with reduced sensory gating of the M50.
Finally, there are two additional points worth considering for MEG measures of sensory gating in schizophrenia. The first is that while most studies do in fact replicate the alteration in response amplitude ratios between first and second stimuli, regardless of whether the specific change is to the first or the second stimulus, at least one study using first-episode, medicated schizophrenia subjects did not find evidence for sensory gating impairment in schizophrenia (Bachmann et al. 2010). A significant number of patients, however, were taking clozapine, which in separate studies has been shown to improve sensory gating in schizophrenia, unlike other antipsychotic medications (Adler et al. 2004). Last, MEG-based sensory gating may be a schizophrenia biomarker amenable to inclusion in clinical trials. Popov et al. (2012) reported preliminary evidence of normalization of M50 and gamma-band measures of sensory gating in a sample of schizophrenia patients assigned to a 4 week cognitive remediation intervention.
3.4 Affect Processing in Schizophrenia
More recently, schizophrenia researchers have been focused on impairments in social cognition in the disorder, and it has long been known that schizophrenia patients have reduced affective expression. Several MEG studies have examined aspects of affect processing in the disorder. Streit et al. (2001) examined visual evoked magnetic fields to standardized pictures of facial affect in patients with schizophrenia (N = 15) and control subjects (N = 12). They reported reduced activations in brain regions including the inferior prefrontal, temporal, parietal and occipital cortices in the schizophrenia group. Inferior prefrontal and fusiform gyrus activity was correlated with behavioral categorization of emotional faces, which was worse in the schizophrenia subjects. A follow-on study of the same dataset examined interregional connectivity using mutual information metrics (Ioannides et al. 2004), observing that schizophrenia subjects had generally weaker linkages between regions involved in the task, including a missing link between right amygdala and primary/secondary visual cortices. Another MEG study employed stimuli from the International Affective Picture System (IAPS) and found that the schizophrenia patients (N = 12) exhibited lower response differences between emotional versus neutral stimuli in frontal and posterior regions of the brain (Rockstroh et al. 2006). They also observed a shift in schizophrenia patients towards emotional valence responsiveness towards the right, rather than left, hemisphere, compared to healthy control subjects. In a separate study involving only neutral-face perception, patients with schizophrenia exhibited significantly greater right hemisphere activation than left, compared to control subjects (Lopez-Ibor et al. 2008). A final MEG study involved a heterogeneous sample of patients including schizophrenia (N = 15), depression (N = 19), drug addiction (N = 10), and borderline personality disorder (N = 6), as well as healthy controls (Weber et al. 2009). This study, which also employed emotional and neutral pictures from the IAPS, examined the impact of early life stress on affective processing by focusing on the visual early posterior negativity (EPN) between 160 and 210 ms. They reported that the EPN response was smaller overall in patients with borderline personality and depression than in schizophrenia patients. They also reported, however, that schizophrenia patients had reduced EPN sensitivity to arousing stimuli. Overall, early life stress was negatively correlated with EPN responses.
4 Spontaneous MEG in Schizophrenia
4.1 Abnormal Slow Wave Activity
Several studies have examined spontaneous low frequency oscillatory signals in resting MEG recordings from schizophrenia patients. Canive et al. (1996) were the first to report the presence of abnormal slow waves in a small sample of schizophrenia subjects using MEG, reporting the presence of the activity in four unmedicated patients out of 11 studied. Fehr et al. (2001) reported increased slow wave activity (delta and theta band), measured via dipole densities, that tended to cluster in frontal and temporal regions of the cortex in schizophrenia patients. In a follow-on study by the same group that compared different mental states (mental arithmetic and imagery) to rest, schizophrenia patients (N = 30) exhibited higher densities of slow wave related dipoles compared to controls (N = 17) in the temporal and parietal regions. Dipole density was correlated with a measure of negative symptoms in the patient group (Fehr et al. 2003). Another MEG research group replicated the increased slow wave result in schizophrenia patients, but reported a relationship between dipole density and both positive and negative symptoms in their sample (Sperling et al. 2002, 2003).
Three studies have examined the effects of medication on abnormal slow wave activity in schizophrenia patients. In a cross-sectional comparison, Fehr et al. (2003) reported that dipole density measure did not differ between medicated and unmedicated patients, in contrast to the earlier report from Canive et al. (1996), who reported that abnormal slow wave activity found in unmedicated patients was not present in the same subjects after antipsychotic medication for 8 weeks. Studies of medication effects within the context of repeated measurements on patients in a controlled trial are more convincing than cross-sectional comparisons. One such study by Sperling et al. (2002) demonstrated that treatment with clozapine or haloperidol had no effect on slow wave density, which also contrasted with the Canive et al. (1996) report. Both medication trials had low numbers of patients, however, and a larger trial would be needed to provide a definitive answer to whether antipsychotic medications reduce slow wave dipole density in schizophrenia.
The specificity of increased focal slow wave activity in schizophrenia has also been examined in MEG studies. Wienbruch et al. (2003) published data from 25 patients with schizophrenia compared to 27 with major depressive disorder and 18 healthy controls. While the schizophrenia patients exhibited the same pattern of increased delta/theta density in frontal regions compared to controls, there was a significant reduction in slow wave activity in the depression sample, relative to both the schizophrenia and control groups. A more recent examination by investigators from the same group confirmed these findings. In a very large sample of 76 schizophrenia/schizoaffective disorder patients, compared to 116 healthy subjects and 42 with mood or somatoform disorders, elevation in slow wave activity was seen in the schizophrenia sample but not in a group comprised of mood and somatoform disorders (Rockstroh et al. 2007). In the Rockstroh et al. (2007
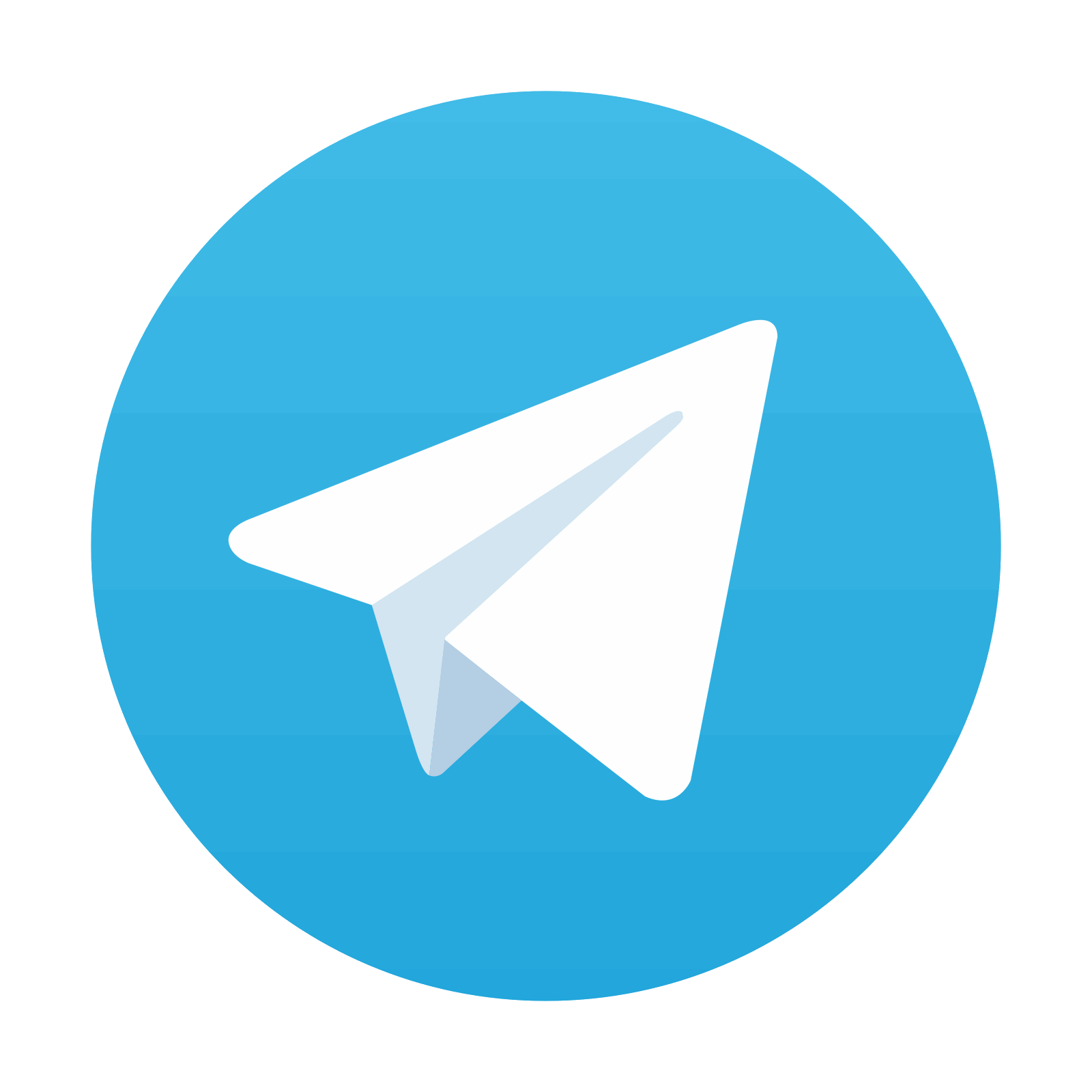
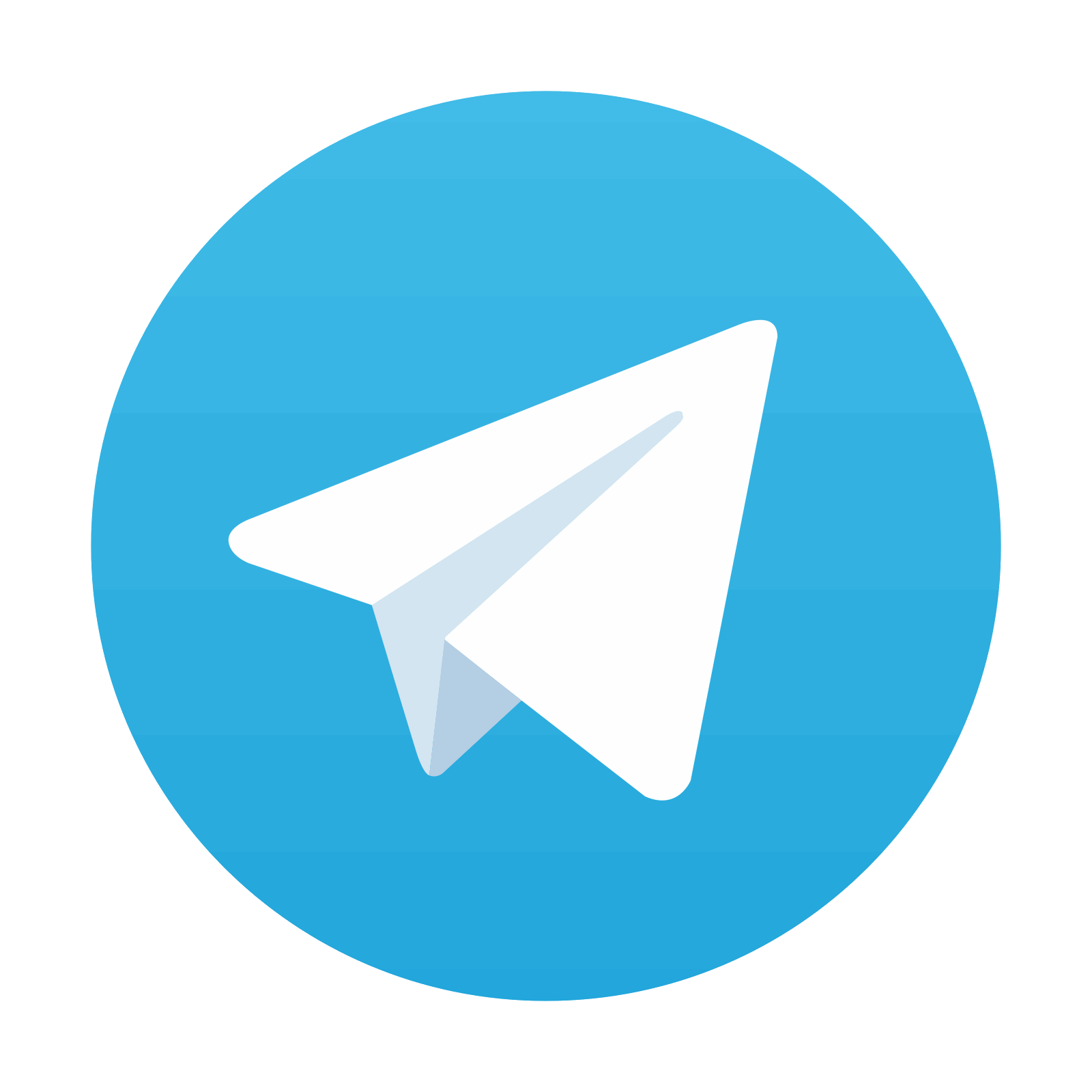
Stay updated, free articles. Join our Telegram channel

Full access? Get Clinical Tree
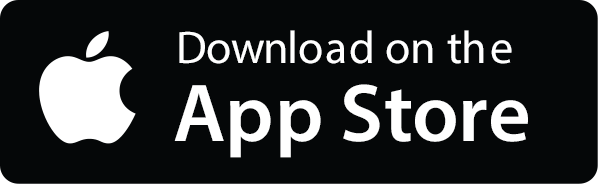
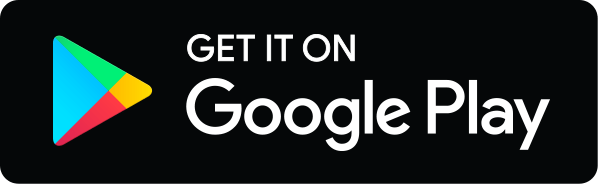