Fig. 23.1
Spatial information or distance (z) is determined from the time delay of reflected light (T) and the speed of light (v) according to the formula z = ∆T × v (With permission from Chest, Michel et al.)
To generate a two-dimensional high-resolution image using OCT, a near-infrared, high irradiance beam of light is emitted from a low-coherence light source. This beam of light initially passes into a beam splitter. One arm of the split beam is directed to a reference mirror and the other through the tip of a fiber-optic probe to shine upon a sample area (see Fig. 23.2).
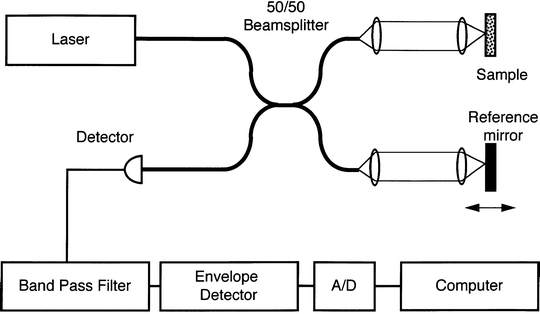
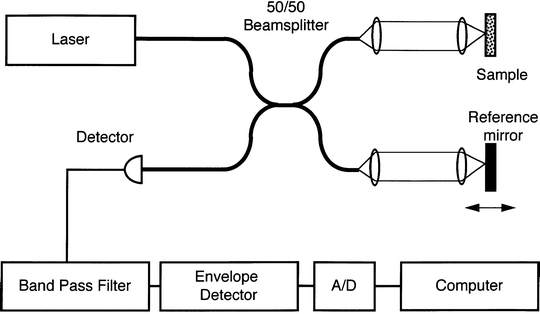
Fig. 23.2
Schematic of the fiber-optic implementation of an OCT System. OCT images are generated by performing successive measurements of optical backscattering versus depth at different transverse positions on the specimen (From Costas et al. with permission from the American Thoracic Society. Copyright © American Thoracic Society)
The sample arm of the split beam is moved laterally along the surface of the tissue of interest. Currently available forward-facing probe devices use a miniaturized electromagnetic mechanism, coupled with an optical lens system to move the fiber-optic tip and provide lateral scanning of tissue over a 2 mm range. Signal intensity decreases as the light signal passes deeper into the tissues (see Fig. 23.3).
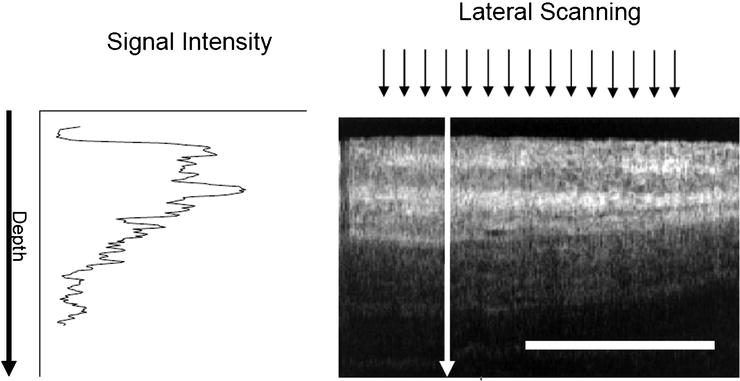
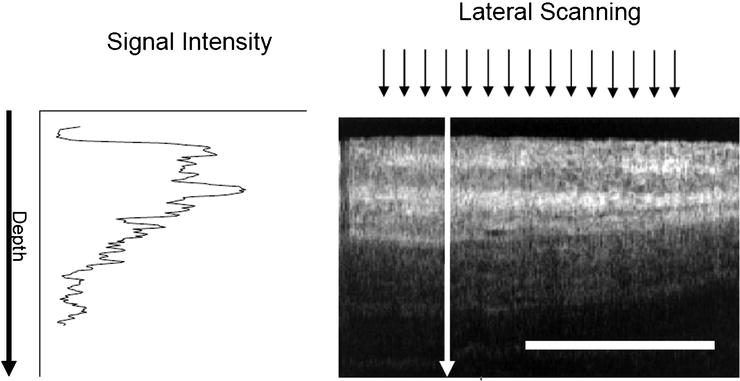
Fig. 23.3
OCT light signal intensity diminishes at increased tissue depth. Lateral scanning range is 2 mm. For reference, the white horizontal scale bar is 1 mm (With permission from Chest, Michel et al.)
Light reflected from the tissues reenters the tip of the OCT probe. The backreflected light from the sample arm of the split beam recombines with the reference arm. The complete signal is delivered to a photodetector. Signal time-delay data in the photodetector is used to generate a real-time view of tissues on a microscopic level. These images can be displayed on an imaging console and saved electronically as digital images. As OCT technology is evolving, available equipment is improving. Current time-domain systems will likely be replaced by more advanced Fourier-domain systems. These newer frequency-based systems use spectrally separated photodetectors, allowing immediate depth scan calculations with enhanced imaging speed.
Early Use of OCT
The use of optical coherence tomography in clinical medicine was initially described in the early 1990s. The earliest and most extensive clinical use of OCT has been in the field of ophthalmology for retinal imaging in patients with macular degeneration. This technology has also been used by otolaryngologists and has been shown to be a feasible adjunct to awake transnasal laryngoscopy. OCT can clearly identify basement membrane violation and transition zones at cancer margins in patients with laryngeal cancer. Gastroenterologists have found that in vivo OCT correctly detected disease features of ulcerative colitis in endoscopically affected segments of colon with high sensitivity. This imaging technology has been studied for use by dermatologists for monitoring cutaneous inflammation and hyperkeratotic conditions. In cardiology, optical coherence tomography is being compared to intravascular ultrasound for characterization of coronary artery disease. OCT imaging has also been used by urologists and gynecologists to further characterize bladder and reproductive tract malignancies.
Optical coherence tomography has started to be used by pulmonologists to assist in the diagnosis and management of lung disease. Unlike ultrasound, light waves do not require a liquid-based coupling medium which may make OCT more compatible with airway imaging. OCT uses nonionizing, near-infrared light which has not been associated with any risks in the airways of humans. At this time, the use of this technology during bronchoscopy has been primarily for research purposes. OCT has proven to be capable of generating images of epithelium, mucosal layers, cartilage, and subepithelial structures in the animal and human trachea in multiple in vitro studies (see Fig. 23.4). OCT has also been shown to identify morphologic changes associated with inflammatory infiltrates, squamous metaplasia, and tumor presence in resected lung specimens (see Fig. 23.5).
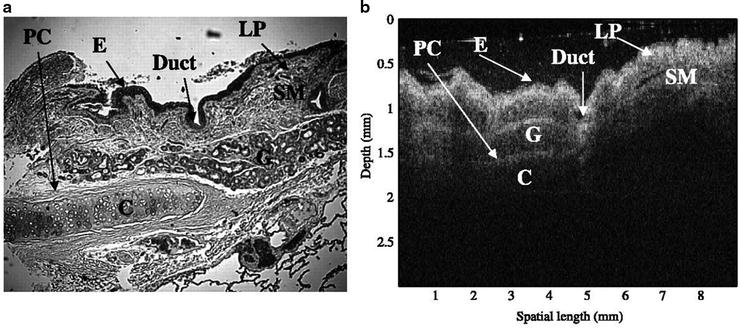
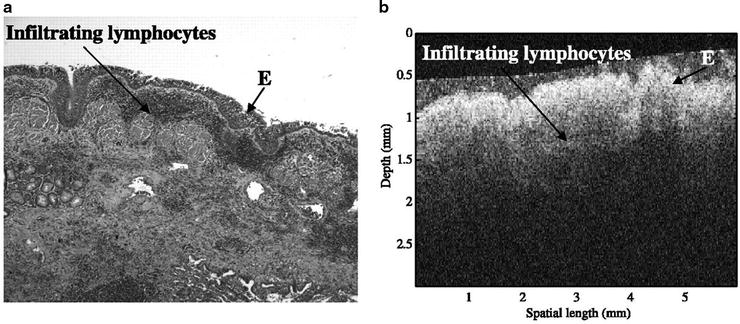
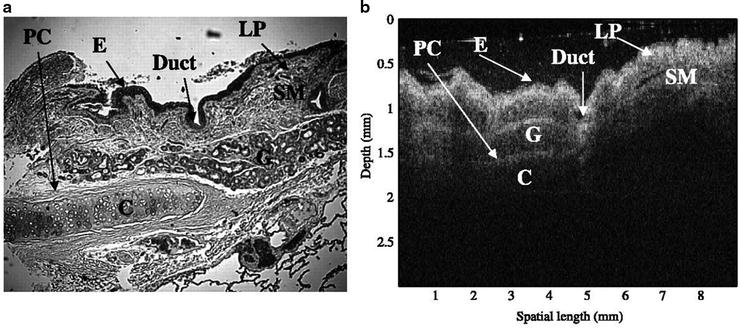
Fig. 23.4
Images of healthy human airways from resected lung specimens by standard histological section (a) (H&E stain; original magnification × 5) and OCT (b). E epithelia, LP lamina propria, SM smooth muscle, PC perichondrium, C cartilage (Awaiting permission from Clinical Cancer Research, Whiteman et al.)
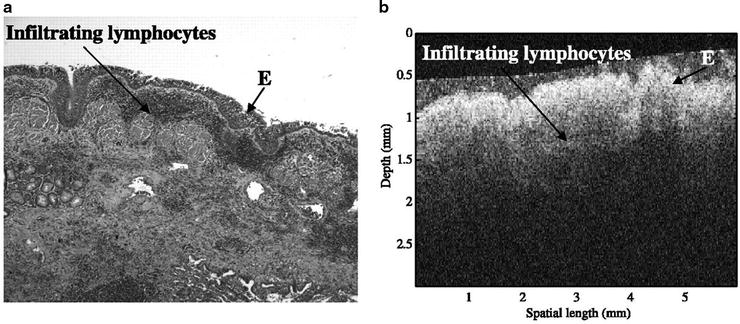
Fig. 23.5
Images of inflamed human airways from resected lung specimens by standard histological section (a) (H&E stain; original magnification × 5) and OCT (b). E epithelia (Awaiting permission from Clinical Cancer Research, Whiteman et al.)
Available OCT Equipment
Optical coherence tomography is a relatively new technology, and at this time, few OCT imaging systems are commercially available. Until recently, the only commercially available OCT system which was cleared by the Food and Drug Administration for use in the United States for lung imaging was the Niris Imaging System (Imalux Corp, Cleveland, Ohio). The Niris system includes an image console with a built-in light source that attaches directly to a long, reusable, flexible, fiber-optic, OCT probe. The distal tip of the OCT probe has an internal electromechanical lateral scanning mechanism encased in a rigid metal tip. In this system, the fiber-optic tip is moved back and forth along a linear axis to generate linear scans. In other OCT devices, the fiber-optic tip can be rotated to create radial scans. Radial scanning has been useful in coronary artery imaging and anatomic OCT which will be described in a later section.
Although the outer diameter of the Niris flexible OCT probe is only 2.7 mm, the currently available model will not pass down the 3.2-mm diameter working channel of a standard therapeutic bronchoscope due to the length of rigid metal probe tip. This problem is currently being addressed by the manufacturer. The Niris OCT probe tip requires mucosal contact for lung tissue imaging. This probe can be sterilized after each use with conventional surgical sterilization devices. The lateral scanning range of this device is 2.0 mm with an image depth range of approximately 2.2 mm. The lateral resolution is approximately 25 μm with a spatial depth resolution of 10–20 μm. These imaging capabilities allow penetration through the upper layers of exposed tissues on airway surfaces where most airway neoplasms originate, and are equivalent to the tissue-sampling depth of conventional endobronchial forceps. The imaging console has a screen, keyboard, and multiple USB port outlets which can be used to save image data (see Fig. 23.6).
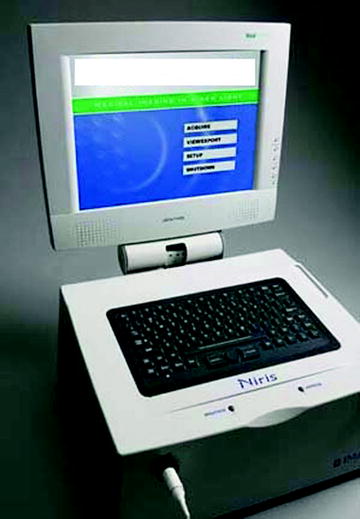
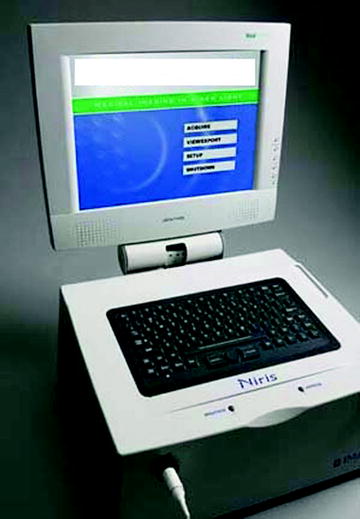
Fig. 23.6
A currently available OCT imaging console (With permission from Imalux)
OCT During Rigid Bronchoscopy
The initial use of OCT for lower respiratory tract imaging in patients in the United States has been during rigid bronchoscopy. Once the rigid bronchoscope is inserted and an area of interest is identified, a flexible OCT probe can be readily advanced through the scope and placed upon the site of interest to generate a topographical image. OCT features of tracheopathica osteochondroplastica as seen in a patient undergoing rigid bronchoscopy have been described. A multimodality bronchoscopic imaging platform including OCT in conjunction with endobronchial ultrasound has been used during rigid bronchoscopy to characterize recurrent respiratory papillomatosis of the trachea. The OCT features of this lesion described by Colt et al. included a layer of heterogeneous light backscattering and a layer of high-degree scattering, corresponding to central, fibrovascular, papilloma core noted on histology (Fig. 23.7). Although OCT use during rigid bronchoscopy has been demonstrated as technically feasible, in order to facilitate widespread routine use by pulmonologists, recent investigatory efforts have focused on the use of OCT during flexible bronchoscopy.
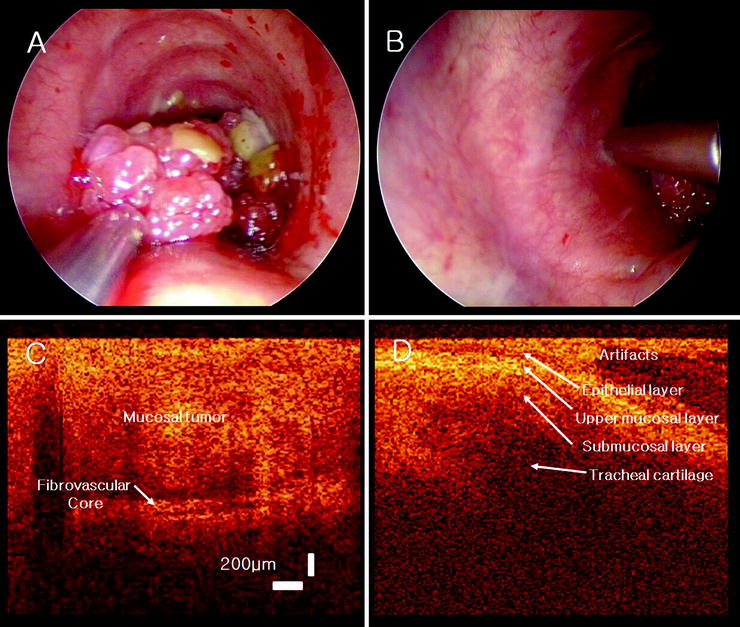
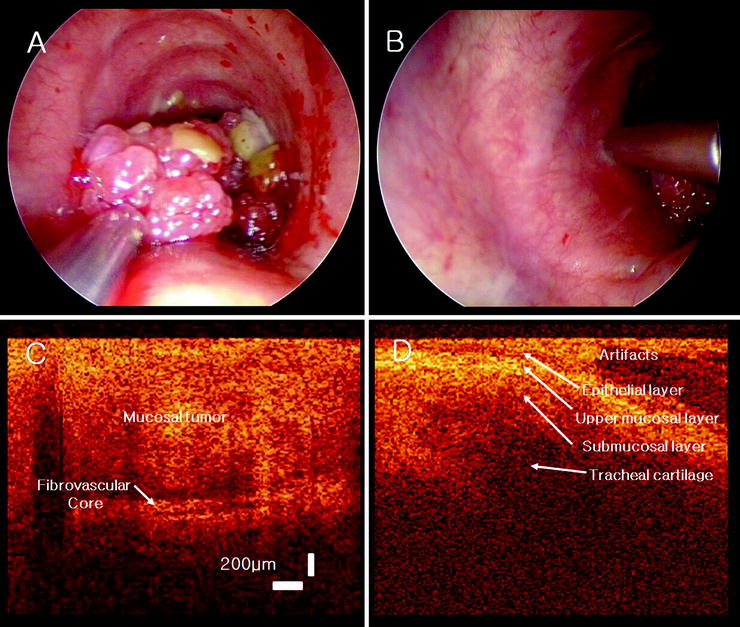
Fig. 23.7
OCT probe overlying an upper tracheal papilloma (a) and the normal tracheal wall (b). OCT images of the papilloma tumor and fibrovascular core (c) and the normal tracheal with mucosal structural layers (d) (With permission from Laryngoscope, Colt et al.)
OCT During Flexible Bronchoscopy
The use of OCT with flexible bronchoscopy has been limited in the United States due to lack of commercially available FDA-cleared OCT devices that are compatible with standard flexible bronchoscopes. Multiple studies outside of the United States have investigated the use of optical coherence tomography with flexible bronchoscopy. In a study by Lam et al., a total of 281 radial scanning OCT images taken during flexible bronchoscopy were compared to corresponding biopsies. Autofluorescence bronchoscopy was used to identify airway sampling sites. A Lightlab/Pentax OCT probe was passed into the airways via the working channel of a flexible bronchoscope and used to generate radial OCT images. Quantitative measurements of the OCT images demonstrated that epithelial thickness of invasive carcinoma was significantly different from carcinoma in situ.
The first use of optical coherence tomography during flexible bronchoscopy in the United States was reported by Michel et al. in 2010. The results of this pilot study suggested that OCT is a technically feasible adjunct to flexible bronchoscopy in the diagnosis of lung cancer. Conventional OCT was performed using a commercially available Niris Imaging System, which is cleared by the Food and Drug Administration for use as an imaging tool in the evaluation of human tissue microstructure. An investigational device exemption for the use of this OCT system during flexible bronchoscopy for lung imaging was approved by the FDA for this study.
In the study by Michel et al., an OCT probe was introduced into the airways of patients with an endobronchial mass during flexible bronchoscopy. The OCT probe tip was attached to the exterior of the flexible bronchoscope (see Fig. 23.8
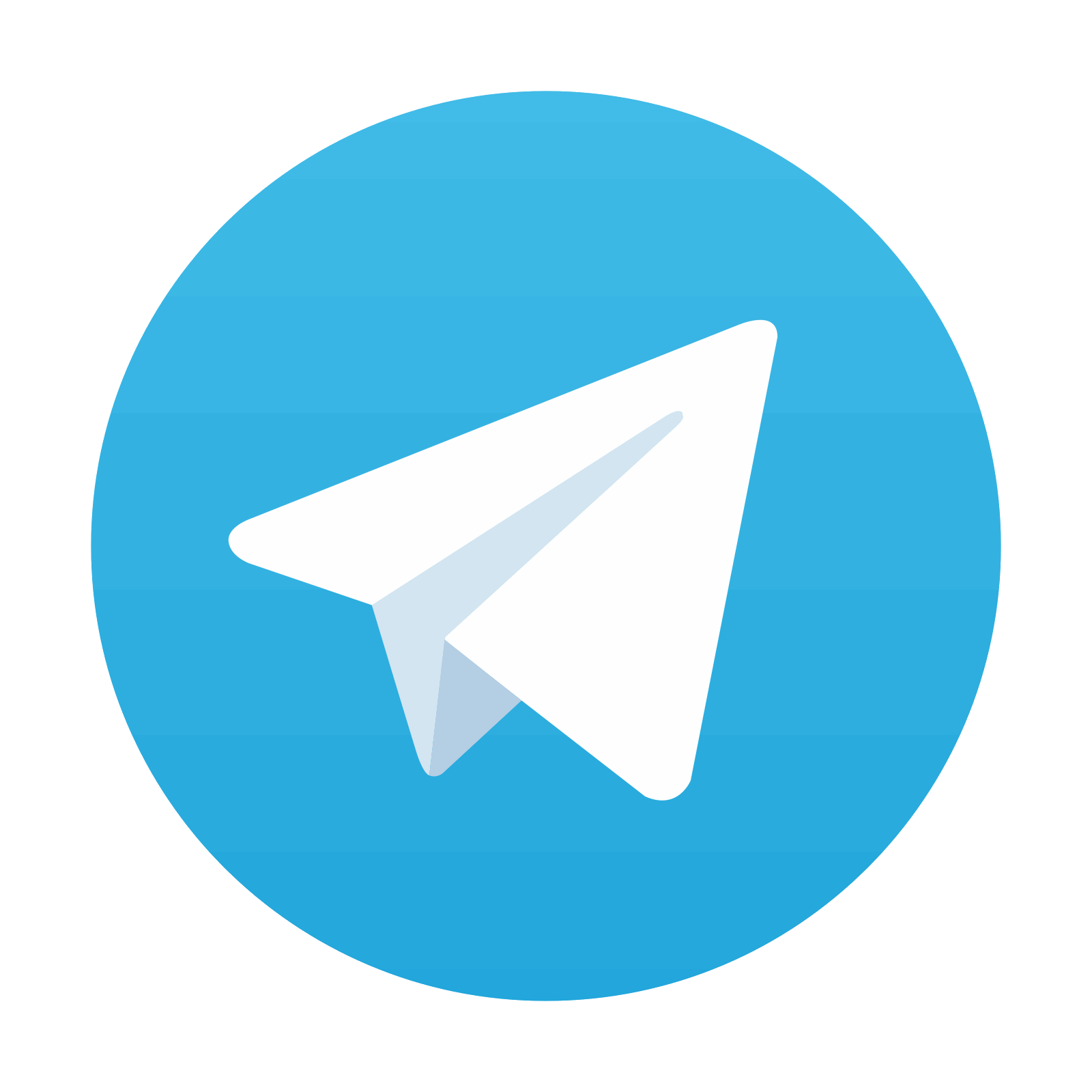
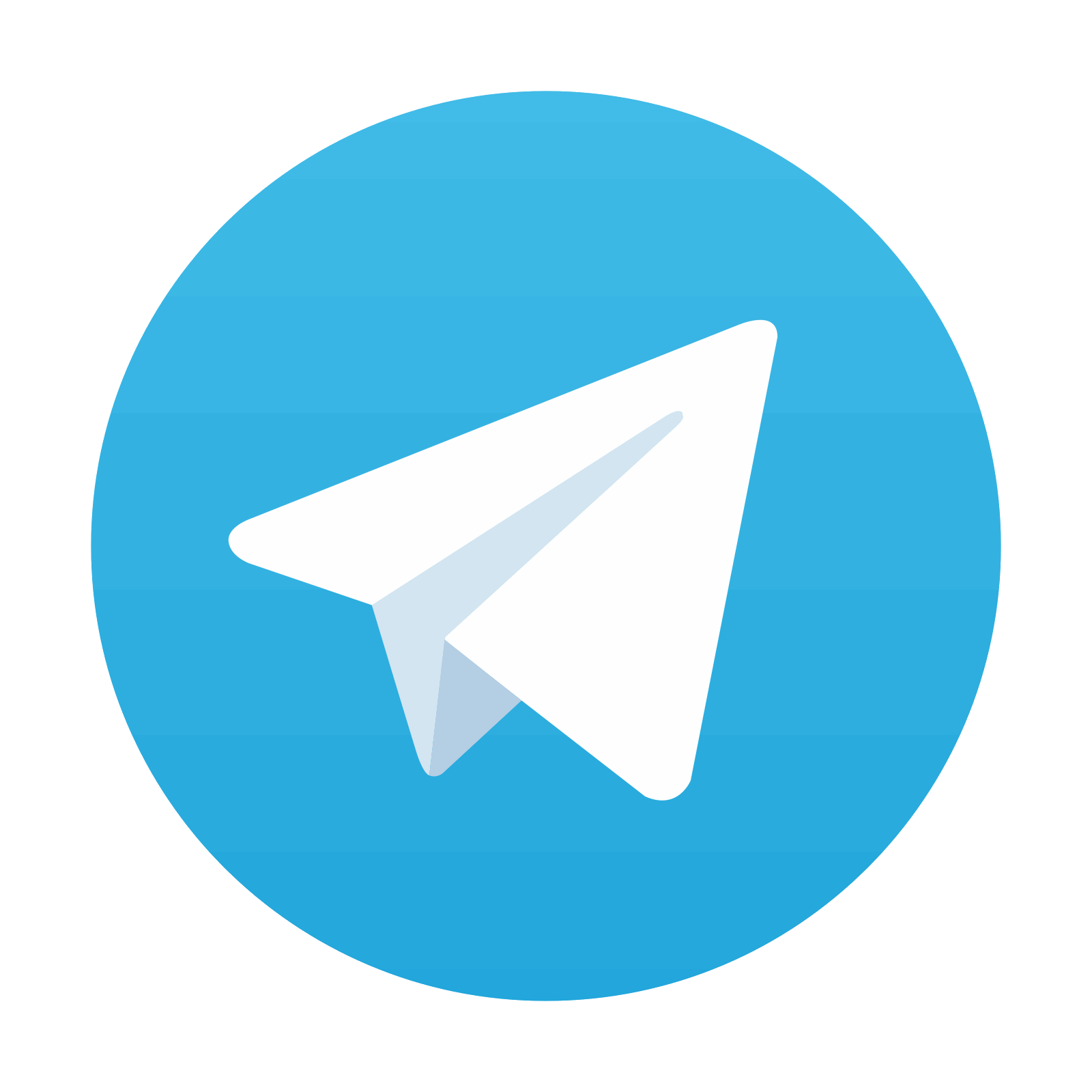
Stay updated, free articles. Join our Telegram channel

Full access? Get Clinical Tree
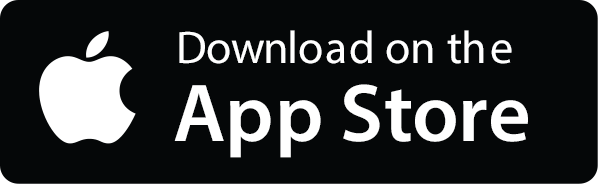
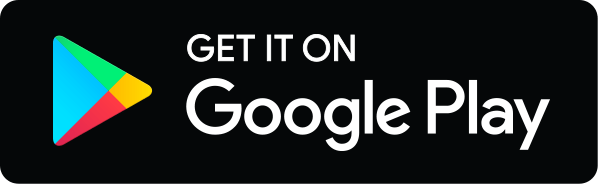