Orbital Diagnosis
Evaluation of the orbit with ultrasound is part of a continuum of imaging techniques whose other primary components are magnetic resonance (MR), computed tomography (CT), and routine x-rays. In the hands of the skilled practitioner, orbital ultrasound using multiple frequencies (including 20-MHz B-scan of the optic nerve) and color Doppler imaging can provide most, if not all, diagnostic information available with other imaging techniques and may also be complementary to other imaging modalities. For reasons as diverse as reimbursement rates and the relative steepness of the learning curve with ultrasound, the bulk of orbital imaging is now performed with readily accessible CT and MR (Figures 5.1 and 5.2).
Even so, ultrasound remains a highly useful adjunct, and its strength within the diagnostic methodology is a result of its cost-effectiveness and ease of repeatability. The higher spatial resolution of ultrasound is also particularly sensitive to detecting early and low grade inflammatory changes in the sclera, optic nerve, and extraocular muscles. Ultrasound complements MR for monitoring treatment of Graves disease as well as imaging periocular drug delivery. Cone occupying lesions, such as hemangiomas and lymphangiomas, can be imaged and diagnosed with ultrasound as well (Figure 5.3). But most extraocular tumors, such as meningiomas or other solid tumors, are best imaged with MR. MR imaging of the orbit is optimal, if it includes the use of an appropriate surface coil, thin slices, contrast, and fat suppression.
Metallic or questionable orbital foreign bodies are best detected with routine x-rays or helical CT (e.g., presence of metallic fragments, such as shotgun pellets, to determine the number of foreign bodies [Figure 5.4] or unusual metal objects, such as nails [Figure 5.5], paper clips [Figure 5.6], or aerials [Figure 5.7]) because MR should not be used when any possibility of ferrous foreign body exists. CT should be limited because of radiation hazards in children and young adults. Ultrasound is useful in detecting low density foreign bodies, such as wood or some plastics in the proximal orbit, particularly if they are surrounded by exudates.
Color flow Doppler ultrasound remains in limited use by ophthalmologists outside of the hospital setting, where higher frequency small parts probes for general radiology ultrasound units are available, and the test may be reimbursable through the radiology department. Flow studies are, however, helpful in quantifying blood flow in the optic nerve and in differentiating suspicious masses by flow characteristics.
All imaging techniques continue to improve with increasing computer power and other technologic advances, particularly in ultrasound, where higher frequency systems and digital analytic techniques permit improved higher resolution imaging of the posterior sclera and the optic nerve.
This chapter will describe ultrasonic examination methods and present a perspective in the complementary use of ultrasound and other imaging methods for orbital evaluation.
TECHNIQUES
Ultrasound aids in the diagnosis of orbital abnormalities by providing information that may not be obtainable by any other examination technique and is noninvasive and easy to perform in a serial manner. As discussed in Chapter 3, we prefer to use combined A- , B- , and M-scan ultrasonic techniques for evaluation of both the globe and orbit. A water-bath standoff with the lids open permits maximum penetration of sound into the orbit.
![]() Figure 5.1 A surface-coil T1-weighted image of an orbit and surrounding tissue shows excellent demonstration of the globe, nerve, orbital muscles, and orbital fat. |
In orbital diagnosis, the topographic outlining capabilities of the B-scan are used for localizing and delineating abnormalities. In our combined technique, after initial localization with B-scan has been achieved, we use the A-scan for tissue evaluation and use A- or M-scan for determination of the pulsatile vascular properties of tissues. Orientation difficulties (as a result of the lack of distinctive anatomic landmarks in the orbit) preclude total reliance on an A-scan-only method. Byrne and Green (1) and DiBernardo and Schachat (2) have provided excellent and comprehensive reviews of
A-scan and combined A-scan/B-scan methods for evaluating the orbit. Their techniques, following concepts by Ossoinig (3), place greater emphasis on the A-scan than do our techniques.
A-scan and combined A-scan/B-scan methods for evaluating the orbit. Their techniques, following concepts by Ossoinig (3), place greater emphasis on the A-scan than do our techniques.
![]() Figure 5.2 A CT scan of an orbit with a foreign body, demonstrating its use for suspected foreign bodies and its excellent delineation of bone. |
![]() Figure 5.3 A 10-MHz A- and B-scan ultrasonogram of a globe and orbit showing the well-encapsulated lesion. A-scan shows internal acoustic reflections of a typical hemangioma. |
![]() Figure 5.5 Plain film x-ray of a nail that penetrated the orbit and cranial vault but did not puncture the globe. (Courtesy of Alan Maberley, MD, Vancouver, BC.) |
Orbital evaluation with ultrasound is typically performed at 10 MHz, with higher frequencies limited to the posterior sclera, optic nerve, and proximal orbital region. The ultrasonic orbital evaluation consists of serial tomographic sections, using both static and kinetic A-and B-scanning. In general, horizontal scans are made serially across the eye and orbit at roughly 2-mm intervals, with the eye in the six major gaze positions. Ossoinig (3) has emphasized the importance of additional meridional scanning of the orbit to portray structures partially obscured by the orbital rim. Lower frequency transducers (such as 5 MHz) may be used to outline the posterior extent and apex of the orbit. A lower frequency may also be useful, if ocular pathology prevents adequate penetration at the normal examining frequency of 10 MHz. However, these transducers are not generally
available in ophthalmic ultrasound equipment and have low resolution.
available in ophthalmic ultrasound equipment and have low resolution.
Kinetic scanning, that is, having the patient move his or her eye from side to side and up and down, while fast sector scans are performed, is important in orbital diagnosis. Kinetic scanning helps indicate the degree of adherence of a mass to the mobile anatomic features of the orbit, such as the optic nerve, and to the fixed structures, such as the orbital wall.
Comparative evaluation of the patient’s other orbit is not usually performed, because variations from the normal orbital pattern in most disease states are easily discerned. However, in patients with subtle pathologic changes, such as disease of the optic nerve, a comparative evaluation of the companion orbit may aid in differentiation.
When a lesion is palpable (e.g., a cyst or mass in the adnexa) it is useful to use direct contact A- and B-scan techniques. The contact probe can be more easily manipulated to confirm the extent and location of the mass. In addition, the contact A- or B-scan can be used to identify a lesion that may be concealed by the bony overhang of the superior orbital rim. These techniques are particularly useful with lacrimal gland tumors. A contact probe to compress various orbital lesions has been described by Ossoinig (3) and is useful in characterizing the cystic or solid components of orbital masses.
A thorough knowledge of orbital anatomy and the pathologic situations likely to arise in the orbit greatly enhances the ability to interpret information that can be obtained from the ultrasonic evaluation. For these reasons, ultrasonic orbital examination is best performed by an ophthalmologist or a technician who is well trained for ophthalmic ultrasonography.
DIAGNOSTIC PARAMETERS
The ultrasound echo patterns seen in the orbital plane arise from the acoustic impedance mismatch between adjacent tissues. Measuring acoustic characteristics of orbital tissues, Buschmann (4) reported sound velocities of 1,462 meters per second for fat compared with 1,615 meters per second for optic nerve and 1,631 meters per second for muscle. The difference in velocity between these tissue components is the acoustic impedance mismatch that causes partial reflection of an ultrasonic wave as it meets the tissue interfaces.
Within a heterogeneous tissue, such as retrobulbar fat, are many smaller tissue elements, including vessels, nerves, and fat globules with many fibrous septa. These multiple tissue interfaces produce individual echoes and result in a nearly uniform confluence of echoes representing the fat pad. The relatively uniform and organized overall tissue structure of the optic nerve and extraocular muscles has markedly less internal impedance mismatches and produces only low-amplitude echoes within the tissue substance. In addition, the optic nerve and, to a lesser extent, extraocular muscles and major structural components are organized in tissue planes parallel to the ultrasonic beam, minimizing echoes that are detectable along the transmitter-receiver beam path. The same principle of reflections from internal structural elements of a tissue applies to tumors and other abnormalities and is a major criterion for differentiation of tissue types. Orientation to the beam is, thus, the major reason that amplitude of the tissue impedance differences alone cannot be absolutely reliable from the A-scan.
TYPES OF DIAGNOSTIC INFORMATION
Clinicians have long relied on radiographic techniques for orbital evaluation. Plain films and computed tomography depict bony abnormalities well—fractures, erosion, or hyperostosis. Vascular contrast studies demonstrate arteriovenous lesions and intracranial abnormalities causing exophthalmos. Some indications of orbital soft tissue lesions may also be gained from these studies, but the findings are often not definitive (5). Direct injection of contrast material into the orbit (contrast orbitography) has been used but has fallen out of favor because of morbidity and diagnostic unreliability. The most common diagnostic tests performed today for orbital imaging are plain film radiography, computed tomography, magnetic resonance imaging, and ultrasonography. Additional tests include plain film angiography and venography, MR angiography (MRA), color Doppler imaging (CDI), and dacryocystography. Radiography methods
and computed tomography subject patients to the known risks of radiation exposure. Magnetic resonance imaging is contraindicated in the case of ferromagnetic foreign objects in the body. CT and MR provide similar soft tissue details in the orbit. CT provides clearer bone details and is sensitive to calcification. MR is better at defining the globe and intraocular structures as well as the optic nerve along its path.
and computed tomography subject patients to the known risks of radiation exposure. Magnetic resonance imaging is contraindicated in the case of ferromagnetic foreign objects in the body. CT and MR provide similar soft tissue details in the orbit. CT provides clearer bone details and is sensitive to calcification. MR is better at defining the globe and intraocular structures as well as the optic nerve along its path.
MR is the best overall way of imaging abnormalities of the orbit. Definition and resolution are superb, but the test is still far more expensive than ultrasound.
Ultrasonography, in contrast, provides unique high-resolution information regarding tumors and inflammatory orbital changes using an inexpensive, reliable, and easily repeatable technique. Bony changes and vascular abnormalities, however, are not well demonstrated, although soft tissue is generally well seen. Ultrasonography can serve as an invaluable complement to the usual radiographic, CT, and MR studies, and can often demonstrate an abnormality (because of its sensitivity and high resolution) when all other tests are negative.
Medical and surgical therapies are aided considerably by ultrasonographic findings. For instance, the effect of steroid administration in presumed pseudotumors can be monitored by following the inflammatory signs ultrasonically. Surgical approaches to a tumor, for example, plaque therapy, can be planned with full knowledge of the location, size, extent, tissue composition, and circumscribed or invasive character of the tumor. In general, ultrasonography, followed by contrast MR or CT, should be the first test used for orbital evaluation. Ultrasound is a sensitive test and rarely misses any significant orbital abnormality. This inherent sensitivity is occasionally misleading (in that inflammatory tissues can resemble neoplasms) so further imaging with MR or CT is desirable, if the ultrasonographic findings indicate a pathologic situation.
INDICATIONS FOR ORBITAL ULTRASOUND
The indications for orbital ultrasonography are summarized in Table 5.1. Ultrasound is specifically useful in documenting clinically, evaluable pathologic states such as myositis, Graves disease, or optic neuropathy. In general, ultrasound is indicated when orbital pathology is suspected. Ultrasound findings may aid in the management and treatment of these orbital pathologic conditions, particularly inflammatory conditions.
TABLE 5.1 Indications for Ocular Ultrasonography | |||||
---|---|---|---|---|---|
|
B-SCAN
HORIZONTAL SCAN PLANE THROUGH THE OPTIC NERVE
With the ultrasonic scan plane passing through the optic nerve, the normal retrobulbar echo pattern is a W-shaped, acoustically opaque (white) area (Figure 5.8). This opaque W-shaped area is bounded anteriorly by the globe and is indented posteriorly by an acoustically empty (black) notch that widens toward the orbital apex. This notch, or triangle, is formed by the optic nerve and associated structures.
The source of the echoes that give rise to the relatively uniform, isoechoic W-shaped retrobulbar echo pattern is not definitely known. Purnell (6) postulated that orbital fat lobules provide the major source of these echoes, and his view is generally accepted. Acoustic discontinuities occur throughout this loculated tissue between intracellular lipids and cell membranes and between cell membranes and loose connective tissue septa. (Similar echo patterns can be produced experimentally in fine-mesh silicone sponge.) M-scans of the orbit have shown marked pulsatile vascular activity, and acoustic discontinuities in the blood vessel network in the muscle cone probably contribute to this echo pattern.
In all normal orbits, the optic nerve consistently appears as an acoustically empty triangular notch in the retrobulbar fat pattern. The optic nerve appears acoustically hypoechoic because of homogeneous tissue structure and because the nerve fibers, septa, and meninges usually lie parallel to the examining ultrasonic beam, thus causing no reflections. The anterior angle of the optic nerve triangle or notch is usually less than 90 degrees (with a range of normally 40 to 70 degrees). Widening or any rounding of this angle may be an indication of pathologic enlargement of the optic nerve or its sheaths.
HORIZONTAL SCAN PLANE ABOVE OR BELOW THE OPTIC NERVE
When scans of the orbit are made inferior or superior to the optic nerve, the acoustically hypoechoic (black) optic nerve triangle is, of course, absent. The retrobulbar pattern appears as a uniform, acoustically isoechoic (white) crescent lying immediately posterior to the globe (Figure 5.9). This crescent becomes progressively wider as the center of the orbit is approached.
VERTICAL AND MERIDIONAL SCAN PLANES
Although horizontal scan planes are generally used, performing both vertical and meridional scans of the orbit is important, because areas of pathology that might be missed in serial horizontal scans may be demonstrated more readily. The vertical scans closely resemble horizontal scans. On an axial scan, the optic nerve notch is demonstrable, and orbital structures can be accentuated by having the patient look in different fields of gaze.
VARIATIONS WITH POSITIONS OF GAZE
The normal retrobulbar fat pattern has been described, with the eye in a straight-ahead direction of gaze. Gaze to the far right or left results in a foreshortening of the retrobulbar pattern (decreasing its thickness) and bending of the optic nerve pattern toward the direction of gaze (shown dynamically on DVD). The movement of the optic nerve with changing positions is diagnostically important. If a mass lesion is found in the orbit, a fast sector scan performed as the patient moves his or her eye may demonstrate the relationship of the mass to the mobile optic nerve.
VARIATIONS WITH AGE
Age has relatively little effect on the B-scan ultrasonographic orbital pattern. The retrobulbar pattern in infants is, of course, smaller than in adults but is identical in outline. Our impression is that the retrobulbar fat pattern in infants and children usually appears denser and more uniformly white than in adults, possibly indicating fat distribution in smaller micelles.
EXTRAOCULAR MUSCLES AND ORBITAL WALL
The outer limit of the acoustically opaque (white) W-shaped retrobulbar pattern is formed by the rectus muscles and intermuscular septa. In B-scans made exactly in the horizontal meridian, a rectus muscle outline can often be seen and traced forward to the globe at its insertion.
The ultrasonic patterns formed by the normal orbital walls in different horizontal scan planes are shown for reference in Figure 5.10.
A small portion of one orbital wall (usually the more perpendicular accessible lateral orbital wall) is usually seen on B-scan ultrasonograms, but, if a large portion of the orbital wall or both medial and lateral walls are seen, edema or inflammation of the ocular muscles or periorbital tissue is suggested. The orbital apex is rarely seen in clinical ultrasonograms but can be approximated by tracing the optic nerve and the rectus muscle/orbital wall echoes to their juncture.
Demonstration of the orbital wall is not a dependable ultrasonographic finding but is a feature dependent on the transducer frequency (more likely to be seen with the lower-frequency transducers, i.e., 5 MHz); depth of ultrasonic beam penetration, for example, focal zone of the transducer; and receiver gain. The following are imperative: (a) to obtain familiarity with the area of orbital wall seen in normal orbits with a given instrument, (b) to use a given transducer, and (c) to calibrate the receiver gain before conclusions can be made, with regard to abnormal prominence of the orbital wall on one or both sides in a scan of a possibly abnormal orbit.
FREQUENCY-RELATED VARIATION
The orbital ultrasonographic appearance varies according to the transducer frequency selected. Figure 5.11 demonstrates a comparison of the normal orbital patterns found, using 10- and 20-MHz transducers. As a rule, the lower the transducer frequency, the deeper the orbital penetration achieved.
ARTIFACTS ENCOUNTERED IN ORBITAL ULTRASONOGRAPHY
Numerous artifacts may occur during B-scan ultrasonography of the orbit, and knowledge of these possible artifacts will help avoid erroneous interpretation of ultrasonograms of the orbit. As discussed in Chapter 3, artifacts may be classified into two groups: (a) reduplication artifacts and (b) absorption defects.
REDUPLICATION ARTIFACTS
These artifactual echoes (also known as axial multiple echoes) occur commonly and usually appear in the central orbit along the axis of the cornea and lens, often in the region of the optic nerve triangle. They represent a “second bounce” of echoes, usually from anteriorly located ocular surfaces. A metal lid speculum can be another common cause of reduplication echo artifacts, especially with immersion ultrasound. These echoes can be distinguished from “real” echoes by moving the transducer either away from or toward the eye in the immersion system. This causes movement of the reduplication echo relative to the tissue, allowing them to be readily identified (Figure 5.11). In CDI, reduplication effects from foreign bodies or other strong, reflective surfaces can cause the so-called twinkle artifact, where false flow is seen (7).
ABSORPTION DEFECTS
Absorption of ultrasound energy by structures located in or in front of the eye may cause abnormal orbital
ultrasonic patterns. Because these absorption defects, or “shadows,” in the retrobulbar fat pattern may simulate orbital tumors, one must be aware of their existence. Ocular tumors (Figure 5.12) or dense calcified lenses (Chapter 3, Figure 3.23) are commonly encountered ocular causes of such orbital defects.
ultrasonic patterns. Because these absorption defects, or “shadows,” in the retrobulbar fat pattern may simulate orbital tumors, one must be aware of their existence. Ocular tumors (Figure 5.12) or dense calcified lenses (Chapter 3, Figure 3.23) are commonly encountered ocular causes of such orbital defects.
![]() Figure 5.11 A comparison of the orbital fat patterns with transducer center frequency. A: 20-MHz scan of the normal orbit, with high resolution but inferior penetration. B and C: 10-MHz scan of the normal orbit, demonstrating good resolution and good penetration. D: 7.5-MHz linear array scan of normal orbit demonstrating poor resolution, but excellent penetration with angular resolution. Right: 7.5-MHz CDI scan of normal orbit showing retinal and optic nerve flow. (see color image) |
When an abnormal orbital B-scan pattern is encountered, the above artifacts should be ruled out. Recognition is aided by careful monitoring of the A-scan, permitting detection of many electronic artifacts, movement of the transducer toward or away from the eye if a reduplication echo is suspected, and analysis of the ocular B-scan for structures that may cause absorption defects in the orbital pattern.
GENERAL CLASSIFICATION OF ORBITAL ABNORMALITY
Ultrasonically, orbital abnormalities can be classified into structural anomalies, mass lesions, inflammatory or congestive changes, or foreign bodies. Each of these categories has several well-defined subdivisions. A-mode findings in orbital pathology have been described extensively by Ossoinig (8) and Byrne and Green (1). B-scan patterns were classified by Purnell (6) and elaborated on by Coleman et al. (9, 10, 11, 12, 13, 14, 15, 16). The flow diagram proposed by Coleman (17) (Figure 5.13) is useful for the examiner in approaching an unknown orbital problem.
Pseudoproptosis of an eye may be accounted for by either a large globe or a shallow bony orbit (Figure 5.14). A-scan measurement of globe diameters will establish any significant difference between the eyes. A posterior staphyloma may be completely outlined with B-scan. With progressively increasing exophthalmos, even with a unilaterally large globe, complete ultrasonography of orbital structures should always be done to evaluate coincident disease.
MASS LESIONS
A distinct distortion of the retrobulbar fat, optic nerve, or rectus muscles by any sort of abnormal contour ultrasonically indicates a mass lesion in the orbit. Purnell (6) first described ultrasound B-scan patterns of the orbit that we have reclassified into four general diagnostic patterns
of mass lesions that are identifiable with B-scan ultrasonography: cystic, solid, angiomatous, and infiltrative (Figure 5.15). Several features of the abnormal area can be used to categorize the lesion, including its contour, sound transmission, internal echoes, and location (Figure 5.16). Close correlation exists between the ultrasonographic findings and the morphologic and histologic characteristics of mass lesions.
of mass lesions that are identifiable with B-scan ultrasonography: cystic, solid, angiomatous, and infiltrative (Figure 5.15). Several features of the abnormal area can be used to categorize the lesion, including its contour, sound transmission, internal echoes, and location (Figure 5.16). Close correlation exists between the ultrasonographic findings and the morphologic and histologic characteristics of mass lesions.
For example, the contour of an orbital tumor may be smoothly rounded, sharply defined, and compressing adjacent normal structures. If the lesion has good sound transmission, its posterior wall will also be clearly evident. Internally, the lesion may be devoid of echoes, indicating no significant tissue interfaces within the lesion. These findings are characteristics of a fluid-filled cystic lesion, such as mucocele or dermoid tumor.
In other cases, a mass lesion may have a contour similar to a cystic lesion. However, its posterior boundary may be indistinct because of poor sound transmission through it, indicating a solid tumor. Low-amplitude echoes within the substance of a tumor indicate minor tissue interfaces, characteristic of a homogeneous solid tumor. These findings indicate a well-circumscribed solid tumor, for example, neurogenic tumor, lacrimal gland tumor, and some metastatic tumors. Location of the lesion within the orbit gives further clues to identification. A lesion of this type within the muscle cone and involving the optic nerve is
probably one of the neurogenic tumors (glioma or meningioma). A similar lesion located in the upper temporal aspect of the orbit is more likely to be a lacrimal gland tumor.
probably one of the neurogenic tumors (glioma or meningioma). A similar lesion located in the upper temporal aspect of the orbit is more likely to be a lacrimal gland tumor.
![]() Figure 5.14 A myopic globe can produce pseudoproptosis and a slightly reduced orbital volume, as demonstrated in this 10-MHz B-scan. The globe outline can also be seen to be staphylomatous. |
![]() Figure 5.15 Schematic presentation of the division of orbital tumor patterns based on their outline and transmission properties. |
![]() Figure 5.16 The location of a tumor in the orbit is an important aspect of acoustic evaluation. Tumors within the muscle cone are particularly susceptible to ultrasonic detection. |
An orbital tumor with an irregular contour suggests a different group of tumors. Angiomatous tumors show fingerlike protrusions extending into the orbital fat pattern, usually with a larger mass more posteriorly. The tumor contour may or may not be well defined. The internal structure of angiomatous tumors presents many dense acoustic interfaces from vessel walls and blood-filled spaces comprising the tumor. Ultrasonically, this heterogeneous interior appears as multiple, irregular, high-amplitude echoes throughout the mass, with little sound attenuation. This irregular contour and heterogeneous internal structure are distinctly different from the cystic and solid tumor patterns described previously.
Another irregular orbital tumor, having a more solid character, indicates a solid infiltrative tumor. Although the anterior lesion outline is jagged, it is also sharply defined and distinct. As with other solid tumors, low-amplitude internal echoes are present and sound attenuation is marked, making the posterior tumor margins indistinct. This pattern is associated with infiltrative tumors, particularly lymphomas and sarcomas. Metastatic tumors may also show these characteristics.
Idiopathic granulomas, or pseudotumors, often mimic invasive, solid tumors clinically and ultrasonically. Orbital hematoma may also appear ultrasonically as an infiltrative mass lesion, although it is nonneoplastic.
A history of trauma can, occasionally, be misleading, because some tumors may bleed with minimal trauma. A contrast MR should be considered for more definitive imaging of most of these lesions. Follow-up serial ultrasonography can be a useful way to document resolution of the lesion, in the case of hematoma.
In our experience, the infiltrative tumor category is the most difficult to identify with certainty, using ultrasound, because inflammatory changes in the orbit, as described later, may produce a similar appearance, especially when edema involves the optic nerve and the sub-Tenon’s space. Scarring from previous orbital surgery may also be confused with this category.
Classifying orbital tumors into the four categories noted earlier is not always possible, because tumors do not have a consistent presentation. However, the pattern and absorption characteristics outlined are consistent with the tumor types noted. Other ultrasonic techniques can augment the available information in these cases. Ballottement of the tumor with a contact probe placed against the eye will demonstrate compression of cystic or angiomatous lesions and resistance to compression with solid tumors. M-scan ultrasound or Doppler ultrasound provides a means of studying vascular pulsations within tumors. Dynamic or kinetic sector scanning will demonstrate motion of orbital structures with changes in gaze position and often aids in categorization.
Individual tumor types, the variations of ultrasonic patterns associated with them, and the reliability of ultrasonic diagnosis with each tumor type will be discussed more fully as separate topics.
INFLAMMATORY AND CONGESTIVE CHANGES
In contrast to orbital mass lesions, inflammatory and congestive processes tend to involve the normal tissues that are present in the orbit, causing subtle changes in them. Inflammatory changes may be classified, ultrasonically, as diffuse or as localized to a particular area or tissue in the orbit, depending upon the specific inflammatory process (Figure 5.17).
A generalized abnormal mottling of the orbital fat pad is indicative of a diffuse orbital inflammation, such as cellulitis. Echoes within the fat are more widely spaced than normal and are of high amplitude, giving the fat pad a more heterogeneous appearance without circumscribed borders. A similar acoustic appearance is seen in traumatic orbital hemorrhage. A localized area of such mottling within the fat is seen with an orbital abscess or focal granuloma. Other localized inflammatory findings involve specific orbital structures. Expansion of the sonolucent (hypoechoic) space between the fat pad
and the orbital wall generally indicates enlargement of the extraocular muscles. The orbital wall may be accentuated in these cases, where the acoustic transmission characteristics of extraocular muscles are modified by the disease state. These findings are particularly characteristic of thyroid-related disease or endocrine exophthalmos, where edema and cellular infiltration of the muscles are present.
and the orbital wall generally indicates enlargement of the extraocular muscles. The orbital wall may be accentuated in these cases, where the acoustic transmission characteristics of extraocular muscles are modified by the disease state. These findings are particularly characteristic of thyroid-related disease or endocrine exophthalmos, where edema and cellular infiltration of the muscles are present.
Localized inflammation and edema may also involve the optic nerve or Tenon’s space adjacent to the sclera. Accentuation of the optic nerve sheath without enlargement of the nerve itself is indicative of optic neuritis or edema—a distinctly different ultrasound presentation from enlargement produced by a tumor. These abnormalities usually resolve with regression of the inflammatory process. Although no pathologic specimens have been examined in the acute stage of retrobulbar neuritis, the ultrasonic and MR findings are consistent with inflammatory edema of the dural sheaths surrounding the nerve. Similar optic nerve findings may be seen with pseudotumor (idiopathic orbital inflammation), papilledema, or vascular anomalies.
The sub-Tenon’s space surrounding the globe may become expanded posteriorly from edema associated with any type of orbital inflammation. Ultrasonically, this appears as a sonolucent area adjacent to the globe wall and connecting to the optic nerve outline. Other inflammatory signs are usually present in the orbit along with this finding. It may also accompany some orbital tumors, particularly granulomatous tumors or lymphomas. In a normal orbit, this becomes a potential space and, as such, is not acoustically evident.
ORBITAL TUMORS
ACOUSTICALLY “CYSTIC” TUMORS
Acoustically “cystic” orbital tumors are those that have a rounded regular outline and good acoustic transmission. It should be emphasized again that acoustic transmission is a relative phenomenon, and the ability to demonstrate transmission through orbital structures depends on a knowledge of the patterns obtained with one’s own equipment and transducers and with careful calibration of receiver gain.
Mucocele
Mucoceles are cysts lined by paranasal sinus mucous membranes. They enlarge slowly because of continued secretion and desquamation of lining cells and frequently cause erosion of the bony walls of the sinus by pressure. They expand in the direction of least resistance, often into the orbit through the medial wall and floor of the frontal sinus, forcing the globe in the opposite direction. Common clinical presentations are pain and periorbital swelling, diplopia, and proptosis. Invasion of the orbit by mucoceles of the paranasal sinuses is an important cause of unilateral exophthalmos. In series compiled by radiologists, orbital mucoceles are the most common cause of unilateral exophthalmos, with a figure as high as 15% in the series compiled by Zismor et al. (18). In series compiled by ophthalmologists, orbital mucoceles are a less common but still significant cause of unilateral exophthalmos. In Reese’s clinical series of 230 cases, 3% of unilateral exophthalmos was a result of orbital invasion by mucoceles (19). In children, Shields et al. (20) note that mucoceles are the most common secondary cysts that occur in children.
Ultrasonography gives accurate information as to the location and size of an orbital mucocele. Secondary changes, such as compression of retrobulbar fat and indentation of the posterior pole of the globe, are well shown by ultrasonography (21).
Mucoceles ultrasonically appear rounded and smooth, or even spherical in contour (Figure 5.18). They are well demarcated from the surrounding normal orbital tissues. Mucoceles demonstrate a sharply defined, rounded anterior acoustic border that indents the retrobulbar fat. They show definite acoustic hollowness, and A-scans through them show few or no internal echoes (Figure 5.18). On B-scan, the interior of a mucocele appears as a solid, black cavity, that is, a sonolucent space like the globe itself. Little sound energy is absorbed in these cystic structures, and, consequently, ultrasonic radiation can penetrate through the mass, clearly outlining its posterior extent and the orbital walls and orbital apex.
Although MR diagnosis is reliable in orbital mucoceles, CT may better confirm the extent of bony erosion,
particularly in the case of paranasal sinus involvement (20). B-scan ultrasonography may add information as to the soft tissue configuration of the orbit and facilitate the choice of surgical approach.
particularly in the case of paranasal sinus involvement (20). B-scan ultrasonography may add information as to the soft tissue configuration of the orbit and facilitate the choice of surgical approach.
Dermoid Cysts
Dermoid cysts are relatively common tumors of aberrant ectodermal tissue. They usually occur in children (22). When dermoid or other epithelial cysts involve the bony orbital wall, they may be diagnosed on x-ray, CT, or MR (23). However, even in this situation ultrasound is valuable, because it can often demonstrate the exact limits of the orbital mass (24,25). In cases without bony involvement (up to 16% in one series) (26), ultrasound is particularly useful in diagnosing these tumors.
![]() Figure 5.19 10-MHz B-scan images of a cavernous hemangioma (H) of the orbit. These tumors are well encapsulated and usually in the muscle cone. The internal A-scan echoes and MR are shown in Figures 5.20, 5.21 and 5.22. |
Cavernous Hemangiomas
Hemangiomas are one of the most common of all orbital tumors. Reese (19
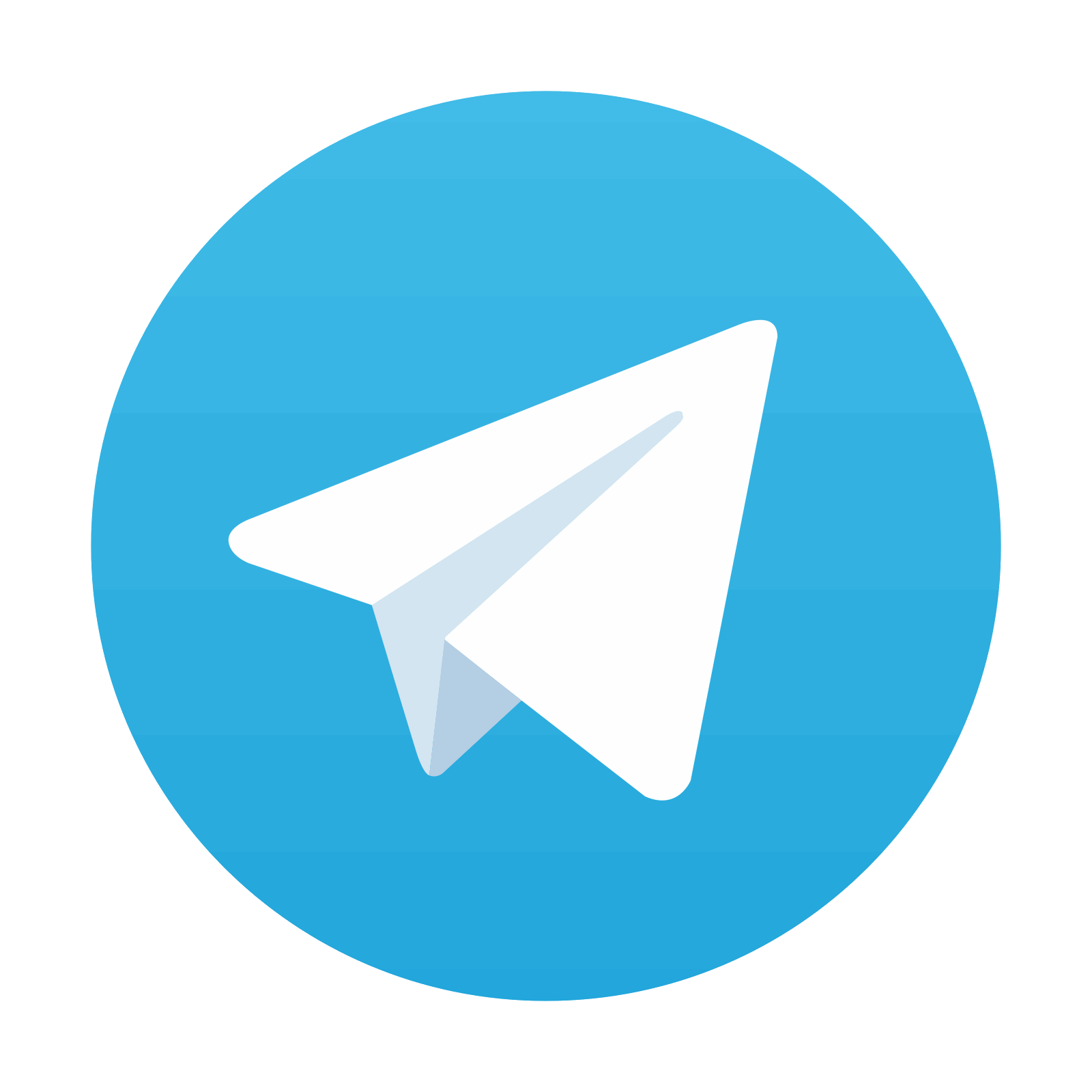
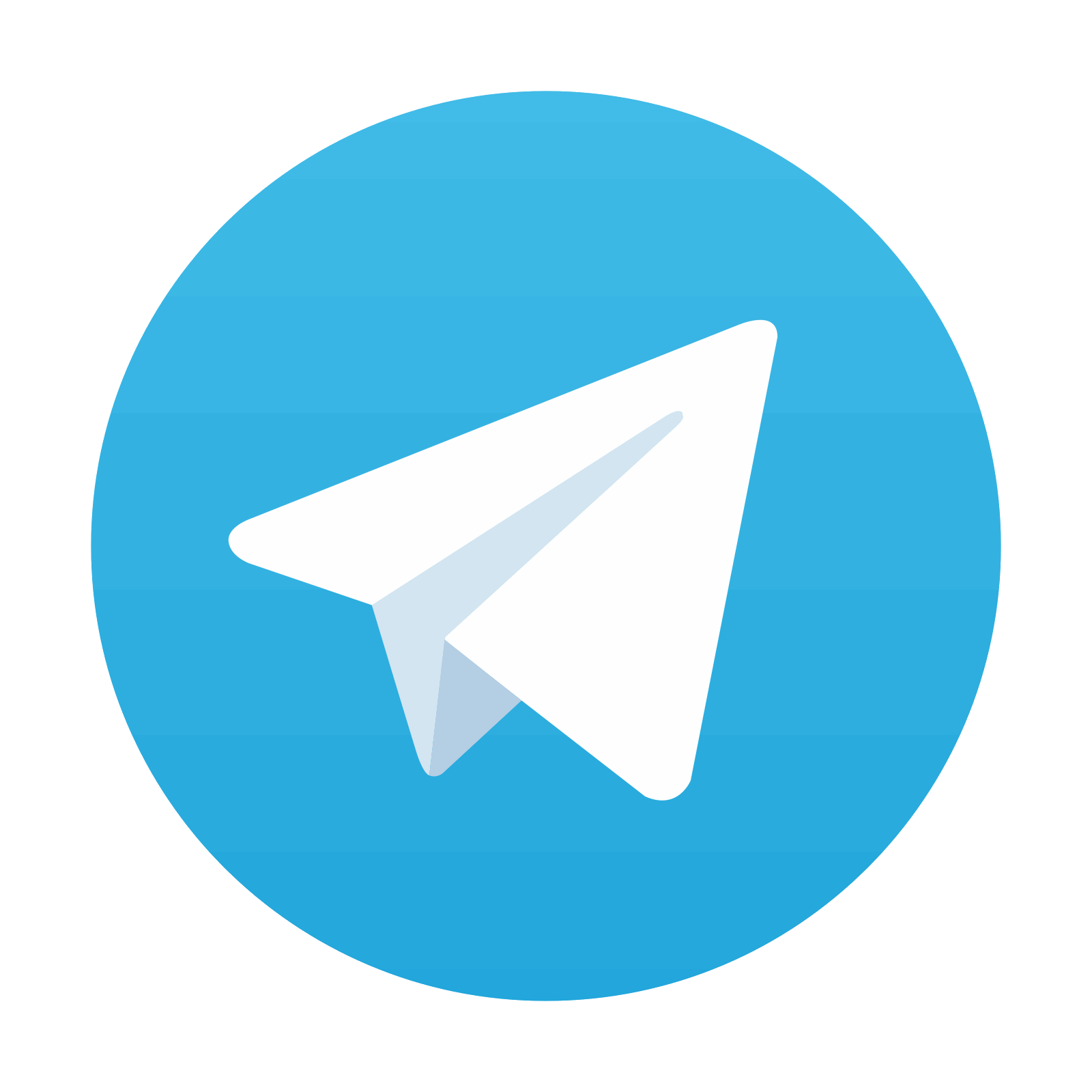
Stay updated, free articles. Join our Telegram channel

Full access? Get Clinical Tree
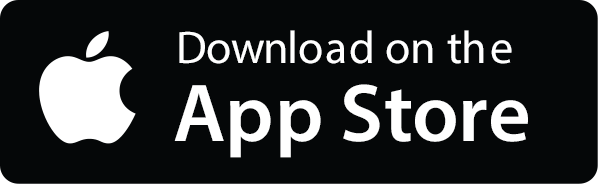
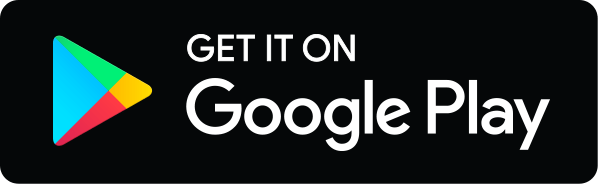
