9
Organ Access Techniques
General Principles for Localization, Drainage, and Stenting
Percutaneous techniques for localization, drainage, and stent placement are now routine, daily procedures in the interventional radiology suite. Utilization of multiple imaging modalities allows access to almost all organs and body compartments. The goal of this chapter is to describe the general principles of localizing and accessing a target organ for draining collections and for stenting obstructive lesions.
Virtually any organ or body cavity can be accessed from a percutaneous route. It is essential to avoid traversing pleura, bowel, and major vascular structures en route to the target. On occasion, however, the stomach may be used as a pathway or ultimate drainage site for pancreatic collections. In addition to direct, percutaneous access, the liver can be biopsied via the hepatic veins. If necessary, some solid organs, for example, the liver and kidney, may be traversed during percutaneous biopsies, aspiration for diagnosis, or drainage.
Imaging Modalities Used for Localization
All radiographic and imaging modalities may be used for accurate localization of the target. A careful review of relevant preprocedure images should be made. This is necessary to select the optimal imaging modality for the procedure, plan the route of access, and determine material requirements for the procedure.
On plain films, some landmarks are especially helpful and will aid the subsequent fluoroscopic access. A collection may be identified by amorphous-appearing gas. Renal shadows sometimes are seen, especially in the hydronephrotic, enlarged kidney. Renal calculi aid greatly in localization of the kidney. Contrast material may be administered intravenously to opacify the renal collecting system if renal function allows. Dilatation of the gallbladder frequently occurs with biliary tract obstruction. The gallbladder shadow occasionally is seen, assisting in localization during percutaneous cholecystostomy. Patients who are postcholecystectomy frequently have surgical clips, which localize the gallbladder bed.
Under real-time fluoroscopic guidance, the needle, catheter, or guidewire can be visualized directly, which permits immediate redirection of the catheter or guidewire when its course deviates from the desired approach. A damaged catheter or wire can be exchanged immediately.
The benefits of fluoroscopic guidance tend to result in an expedient procedure. The disadvantages include relatively poor contrast resolution. Often the target cannot be seen readily, which may necessitate additional localization punctures. Also, vital structures (e.g., blood vessels) in the anticipated course may not be readily apparent. Thus, it is often helpful to do a cross-sectional image [usually computed tomography (CT)] before a fluoroscopically guided procedure.1 Real-time imaging requires patient cooperation (i.e., breathhold for limited motion). There is also radiation exposure to the patient and physician, although this can be minimized by proper technique.
Ultrasound (US) has increasingly become an extremely useful modality for target localization. In some practices, it has become a primary guidance modality for organ localization. US offers real-time imaging, with potentially excellent tissue contrast for both localization and needle/catheter tract identification. US is often used in combination with fluoroscopy. For example, during percutaneous nephrostomy, after localizing puncture with US, aspiration of about 5 mL of urine is performed, and the needle is injected with an equal amount of contrast to opacify the system for subsequent fluoroscopic guidance.1 US optimally requires specialized needles, guidewires, and catheters that are sufficiently echogenic for proper visualization. It is usually cost-effective but is highly operator and patient dependent.
Computed tomography (CT) is an extremely useful modality because it provides excellent tissue contrast. CT provides clear visualization of the course of the anticipated needle tract and will demonstrate any intervening structures that might preclude placement of the desired drainage catheter.2,3 The target lesion or anatomic structure is shown clearly on CT. The disadvantages include lack of real-time monitoring and delays to check the progress of needle placement by repeated scanning. Patient cooperation is a necessity because variations in breathing or breath-holding can result in large variability in needle placement. CT is a more expensive guidance technology than fluoroscopy or US. With the advent of fast CT scans, procedures can be done more expeditiously, although not in real time.
Magnetic resonance imaging (MRI) also provides excellent tissue contrast, imaging in any plane required for diagnosis, and visualization of the needle/catheter tract; however, specialized instruments that are nonferromagnetic are required. Conventional MRI does not offer realtime imaging and is presently the most expensive guidance modality. Its primary interventional use is in neuroradiologic procedures. Real-time MRI “fluoroscopy” is in early development.
Goal of Access
Percutaneous access to an organ, abscess cavity, or infected or obstructed anatomic pathway can be performed for biopsy, as described elsewhere in this book. Aspiration of a fluid collection may be performed for diagnosis (i.e., culture and sensitivity) or for therapeutic drainage. In less frequent circumstances, a cavity or pseudocyst can be sclerosed by alcohol or other agents to facilitate its closure. Urinary and biliary tract obstructions can be relieved by diverting the path of urine or bile into an external drainage bag (e.g., biliary drainage) or by crossing the site of obstruction and reestablishing the normal antegrade anatomic pathway (e.g., biliary stenting).
Obstructions are typically caused by calculi, tumor, or inflammation. Calculi are frequently the cause of urinary tract obstruction and can result in compromised function of the affected kidney and infection of the stagnant urine. Therefore, rapid relief of urinary tract obstruction is important. The therapeutic drainage procedure is termed percutaneous nephrostomy tube placement. Tumor is also a frequent cause of obstruction in the urinary tract and biliary tree. Primary urinary bladder or ureteral tumors (usually transitional cell) as well as primary pelvic malignancies or metastases are additional causes of urinary tract obstruction that require nephrostomy. Ureteral stent placement can be performed percutaneously, but cystoscopic retrograde stent placement usually is attempted first because it is less invasive than the percutaneous procedure. Percutaneous nephrostomy or ureteral stenting frequently is performed when the tumor causing the obstruction is inoperable or is radiosensitive. The ureter frequently responds to radiation therapy with stricture formation. Therefore, a ureteral stent can be placed prophylactically or when a stricture becomes symptomatic. Other causes of stricture include postoperative injury, ischemia, or passage of ureteral calculi. Percutaneous nephrostomy also is indicated for bladder dysfunction, colovesicular or coloureteral fistulae, or bladder leaks of any cause. Urinary diversion by nephrostomy drainage or stenting across a ureteral tear may be used to aid healing.4
The most common cause of biliary obstruction in the United States is carcinoma of the pancreatic head. Other causes include primary bile duct tumors, bile duct stones, and postoperative injury. Biliary obstruction that results in pruritis or sepsis requires decompression of the biliary tree. A temporary stent is used before a planned operative procedure is done or if resolution of the bile duct obstruction is expected. Typically, permanent stent placement is reserved for inoperable carcinomas. Often a diagnostic cholangiogram and stenting can be performed in one sitting. It is sometimes helpful to perform a Gram stain to determine immediately whether a cavity is infected if this is not readily clinically apparent. If positive, the original puncture then may be used immediately for placement of a drainage catheter.
Materials
Needles
Although a large number and type of needles are available for aspiration and biopsy, only a limited number are used for organ access and catheter placement. The prototype is the Chiba needle, a type of aspiration biopsy needle. The Chiba needle is available in multiple gauge sizes, although the 21- or 22-gauge “skinny” needles are used most frequently. If inadvertent nontarget or vascular puncture occurs or if multiple passes are needed, this small gauge is relatively atraumatic. Chiba needles range in length from 10 to 20/cm. The needle tip is beveled at 25 degrees. Originally designed to obtain cytologic or bacteriologic samples, skinny needles are used increasingly to access visceral organs for drainage and stenting. (Needles with a greater ability to cut typically are used for biopsy; these are available from a wide variety of manufacturers and are typically “gun”-type spring-loaded devices.) For organ access, skinny needles are used for atraumatic access to the target, for example, the biliary tree or intrarenal collecting system. The initial skinny needle approach is completed with contrast opacification of the target for localization and diagnosis. Vendor-specific skinny needles are also incorporated as the initial components of “one-stick” systems used for upsizing the initial puncture site to allow for drainage or stenting.
Access to the kidney and biliary trees which are deep, vascular organs, as well as to many more superficial targets, is typically done with the Seldinger technique; that is, a needle is first placed into the target and exchanged over a guidewire for the drainage catheter. Intermediate dilatation of the track is usually needed. Traditionally, 18- or 19-gauge, 15-cm long, hollow-core needles were used with exchange over a 0.035-inch to 0.038-inch stiff working wire (Rosen or Amplatz type). Many interventional radiologists now prefer “one-stick” systems that allow relatively atraumatic localization with a 21-gauge needle and 0.18-inch wire, with subsequent exchange for a triple coaxial dilator that will accommodate a 0.035-inch or 0.038-inch working wire. (After this wire is placed, the track can be dilated to accommodate a standard 8Fr to 12Fr catheter) (Fig. 9-1
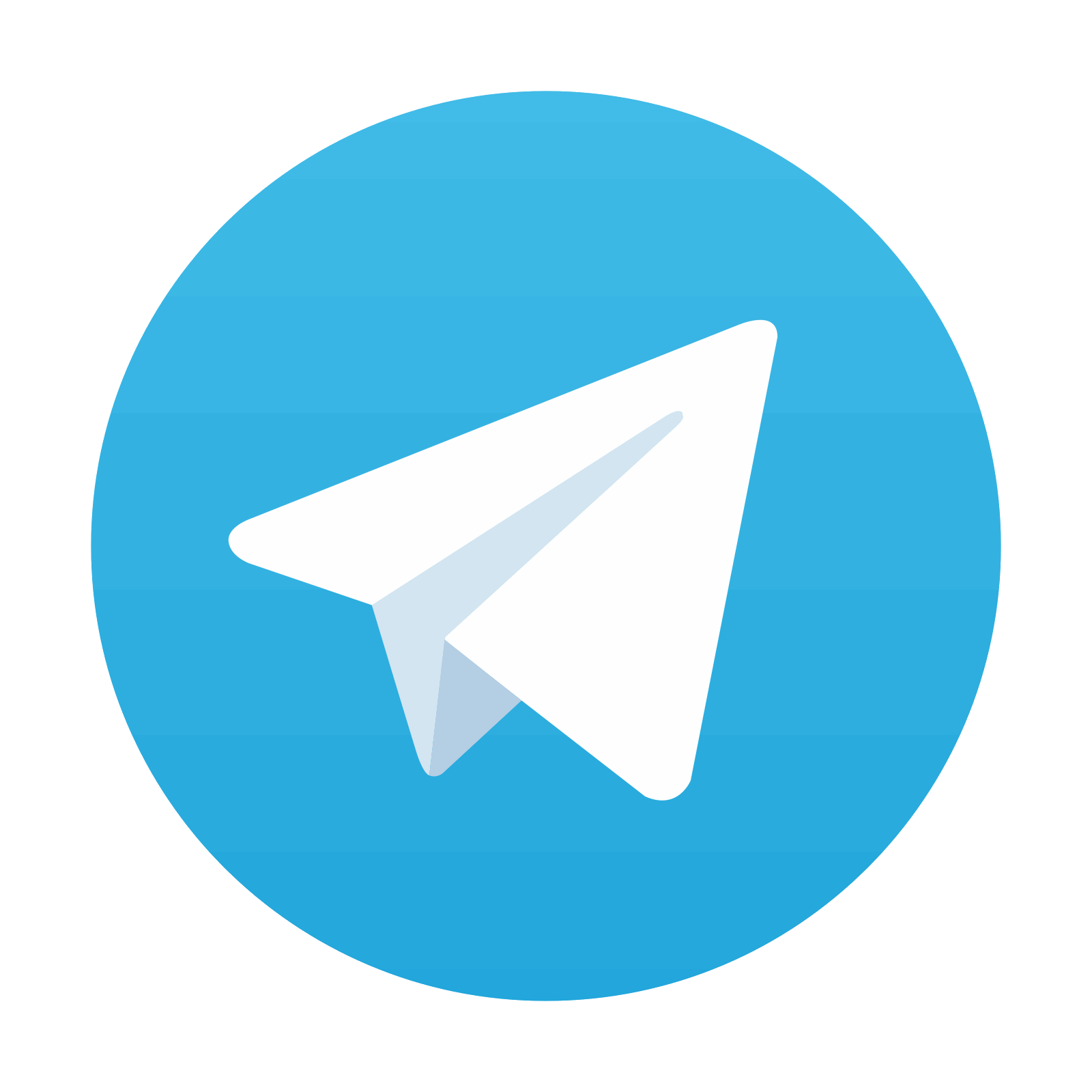
Stay updated, free articles. Join our Telegram channel

Full access? Get Clinical Tree
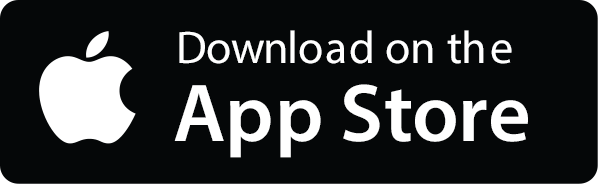
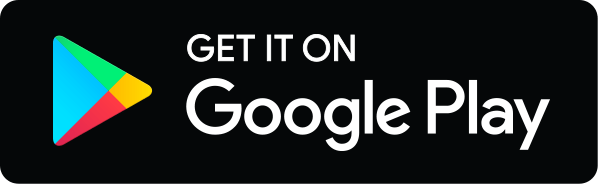