In contrast with the algorithms and screening criteria available for adults with suspected pulmonary embolism, there is a paucity of guidance on the diagnostic approach for children. The incidence of pulmonary embolism in the pediatric population and young adults is higher than thought, and there is an urgent need for updated guidelines for the imaging approach to diagnosis in the pediatric population. This article presents an up-to-date review of imaging techniques, characteristic radiologic findings, and an evidence-based algorithm for the detection of pediatric pulmonary embolism to improve the care of pediatric patients with suspected pulmonary embolism.
Key points
- •
Pulmonary embolism prevalence is much higher in children than thought; timely diagnosis is critical.
- •
There is a relative paucity of guidance on the imaging approach to diagnosing pulmonary embolism in children. Adult-based diagnostic algorithms are not applicable to the pediatric population.
- •
Pediatric-specific risk factor assessment is an important primary tool for guiding decision-making regarding imaging for pulmonary embolism, preferably with computed tomography pulmonary angiography.
- •
Most children diagnosed with pulmonary embolism have predisposing risk factors, including immobilization, a hypercoagulable state, an excess estrogen state, an indwelling central venous line, and prior pulmonary embolism and/or deep vein thrombosis.
- •
Radiologists need to work closely with referring clinicians to choose the most appropriate imaging modality and be familiar with recent imaging advancements to maximize the diagnostic yield, while minimizing radiation dose.
Introduction
The incidence of pulmonary embolism (PE) in the pediatric population and young adults is higher than previously thought. If not diagnosed and treated promptly, PE poses a substantial risk of morbidity and mortality (≤10%). , The clinical presentation of PE in children is highly variable and may even be clinically asymptomatic. Given their substantial cardiopulmonary reserve, children are unlikely to be symptomatic unless there is a greater than 50% obstruction of the pulmonary circulation or coexisting cardiopulmonary disease. , Therefore, high clinical suspicion, guided by pediatric-specific risk factor assessment, is necessary for prompt diagnosis of pediatric PE. In addition, accurate imaging evaluation, which can directly or indirectly visualize PE, is essential for the initial detection and follow-up evaluation.
Multiple diagnostic algorithms and screening criteria for adults suspected of PE (i.e. Wells criteria, Pulmonary Embolism Rule Out Criteria, the Geneva score, etc.) are currently available and widely used. However, there is a relative paucity of guidance for the diagnostic approach to PE in children. Therefore, the goal of this article is to present an up-to-date review of imaging techniques for pediatric PE, including the sensitivity and specificity of various modalities and characteristic radiologic findings, and to present an evidence-based algorithm to guide the imaging approach to detection of PE in children.
Evidence-based imaging algorithm
Five currently available imaging modalities for diagnosing PE in children are radiography, computed tomography pulmonary angiography (CTPA), nuclear medicine ventilation and perfusion (V/Q)scan, MR imaging, and conventional angiography. Given the paucity of scientifically proven data specifically for the pediatric population, the sensitivities and specificities of various imaging modalities for the detection of PE are still mainly based on information obtained in adult patients with PE. The sensitivity, specificity, advantages, and disadvantages of the currently available imaging modalities for diagnosing PE in children are summarized in Table 1 .
Imaging Modality | Sensitivity | Specificity | Advantages | Disadvantages | References |
---|---|---|---|---|---|
Radiography | 3%–20% (individual indirect signs of PE) | 80%–95% (individual indirect signs of PE) | Low radiation dose, widely available, alternative diagnosis | Lack of sensitivity | PIOPED I (Worsley) |
CTPA | 83%–100% | 89%–97% | Noninvasive, relatively fast/available, sensitive and specific, alternative diagnosis | Relatively high radiation dose, iodinated contrast | PIOPED II ( Hayashino ), Stein, Patel, Coche, Qanadli, Winer-Muram |
V/Q scan | 78% a | 98% a | Alternative to iodinated contrast tests, noninvasive | Difficult technique in children <5 y of age, may require more specific testing, relatively high radiation dose | PIOPED II (Sostman), Patocka, Zaidi |
MR imaging | 84% b | ∼100% b | No radiation, noninvasive, alternative diagnosis | Sedation often required, high cost, lack of availability and scanner time | Kalb B, Benson |
Angiography | 32% c –99% | ∼100% | Role for thrombectomy/thrombolysis | Invasive, radiation dose varies, can miss subsegmental PE | Wittram (PIOPED II), Stein |
a Excludes intermediate and low risk, often leading to further testing. Gold standard in these cases either digital subtraction angiography or CTPA in combination with Wells criteria.
b In 22 adult patients when using combination of conventional bolus-triggered 3-D MR pulmonary angiography, respiratory-triggered cardiac-gated true fast imaging with steady-state precession, and contrast-enhanced low–flip-angle 3-D gradient-echo sequences.
c MDCT was shown to be more sensitive in diagnosis of subsegmental pulmonary emboli compared with conventional angiography.
Practical imaging approach to pediatric pulmonary embolism
Survey Study Information on Current Imaging Modality for Evaluating Pulmonary Embolism
Lee and colleagues published survey results collected in 2009 from 160 members of the Society of Pediatric Radiology spanning 118 institutions regarding the practices and policies for diagnosis of PE with emphasis on CTPA. Of the 118 respondents, 104 (88%) performed CTPA in children with clinical suspicion of PE and 93 (89%) of those 104 use CTPA as the first imaging choice. Ten respondents (10%) reported using a V/Q scan, and 1 (1%) reported conventional angiography as their usual study of choice in patients with suspected PE. Twenty-six respondents (25%) reported having a written policy or clinical pathway established for the diagnosis of PE. The majority of the respondents who reported using CTPA in children with suspected PE (64%) stated that obtaining a chest radiograph before the CTPA, whereas 7% did not obtain a chest radiograph before CTPA. The remaining 29% reported variably obtaining a chest radiograph before CTPA “depending on the clinical circumstances and/or ordering physician’s preference.”
The demographics of the respondents and the frequency of CTPA performed in children, and theuse of dose reduction techniques varied widely across institutions. Of the 118 respondents, 80 (68%) primarily worked in academic settings, 27 (23%) in a private practice setting, and 11 (9%) in combined academic and private settings. However, the frequency of CTPA examinations ordered between academic, private, or combined settings did not differ. The majority of respondents (58%) reported modifying CTPA protocols to decrease the radiation dose in children with the 3 most common measures, including mAs reduction, automatic exposure control, and a decreased kVp. However, a significantly higher percentage of radiation dose-reduction techniques were used within academic institutions compared with private institutions ( P = .03).
Current Imaging Techniques
Radiography
Chest radiographs are neither sensitive nor specific for the diagnosis of PE. , Because radiographs are widely available, relatively low cost, and have a relatively low radiation dose, chest radiography may be helpful for providing an alternative diagnosis for acute symptoms, such as pneumonia, pneumothorax, rib fracture, or pulmonary edema. For these reasons, chest radiographs are often the first step in the workup of suspected pediatric PE; however, additional imaging studies are needed to definitively diagnose or exclude PE in the appropriate clinical scenario, especially when 2 or more established risk factors are present.
Normal chest radiographs do not exclude the diagnosis of PE. For example, up to 12% of chest radiographs are normal in adult patients found to have a PE. Several secondary radiographic signs of pediatric PE have been described, including the Westermark sign (oligemia), the prominent central pulmonary artery sign (Fleischner sign), pleural-based increased opacity (Hampton hump), and other noneponymous signs, such as vascular redistribution, pleural effusion, and an enlarged hilum. The sensitivity and specificity of these radiographic secondary signs of PE vary widely from 3% to 20% and 80% to 95%, respectively, and most secondary signs, especially the latter, are considered nonspecific.
Computed tomography pulmonary angiography
After the PIOPED II study, multidetector CTPA emerged as the imaging modality of choice for the diagnosis of PE in adults and children owing to its fast scanning time, widespread availability, high spatial resolution, and high sensitivity and specificity. , PIOPED II is the largest study to date that compared CTPA with a composite reference standard, spanning 8 clinical centers; however, it is important to note that PIOPED II excluded patients younger than 18 years of age. Compared with conventional angiography, CTPA has also been demonstrated to be more sensitive in detection of subsegmental PE. In addition, owing to the high anatomic detail provided by this cross-sectional imaging modality, CTPA may also demonstrate alternative diagnoses that may clinically mimic the nonspecific symptoms of PE in children, such as pneumonia, atelectasis, malignancy, congenital heart disease, and, less commonly, pericardial effusion, rib fractures, right atrial thrombus, and fat embolism. Furthermore, faster scanning times of newer MDCT scanners has decreased the number of children requiring sedation for CTPA scans, substantially decreasing the need for sedation and the cost of using anesthesia resources.
Computed tomography pulmonary angiography parameters
CTPA parameters depend on institutional imaging guidelines. However, in general, collimation size decreases depending on the number of detectors of the scanner, such that an 0.75 mm collimation is typically used for 16 MDCT, an 0.625 mm collimation for 32 MDCT, and 0.60 mm for 64 MDCT. A high pitch range between 1.0 and 1.5 is recommended. Weight-based low-kilovoltage scanning simultaneously decreases the radiation dose and increases the detection of both central and peripheral pulmonary emboli. In both children and adults, the use of sagittal and coronal multiplanar reformats as well as 3D reconstruction of the pulmonary vasculature have been found to result in significantly increased detection of PE and interobserver agreement. ,
Contrast optimization
Optimal contrast enhancement of the pulmonary arteries is critical for the detection of PE ( Fig. 1 ). One large study found that 80% of CTPA studies in children are limited in the evaluation of subsegmental pulmonary arteries owing to suboptimal contrast opacification. Before performing a CTPA, it is essential to carefully review the underlying cardiac anatomy and tailor the CT technique to optimize contrast enhancement of the pulmonary arteries ( Fig. 2 ). Nonionic iodinated intravenous contrast at a dose of 2 to 4 mL/kg (volume to not exceed 125 mL), preferably administered via power injector at rates specific to intravenous catheter size (3.0 mL/s for 20G, 1.5–2.0 mL/s for 22G, 1.0 mL/s for catheters smaller than 22G or in the hand or foot). Faster injection rates are preferred for optimal pulmonary arterial enhancement.
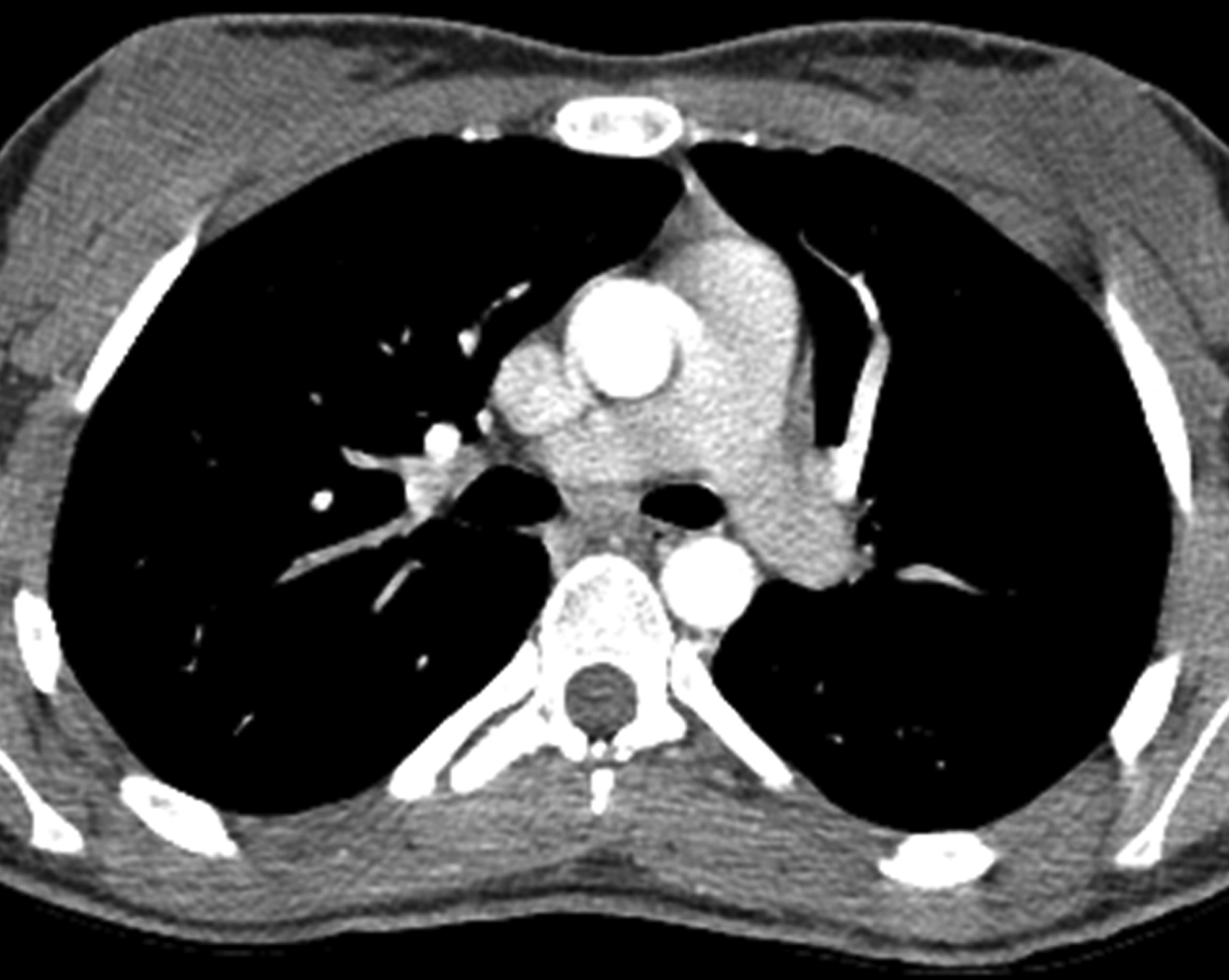
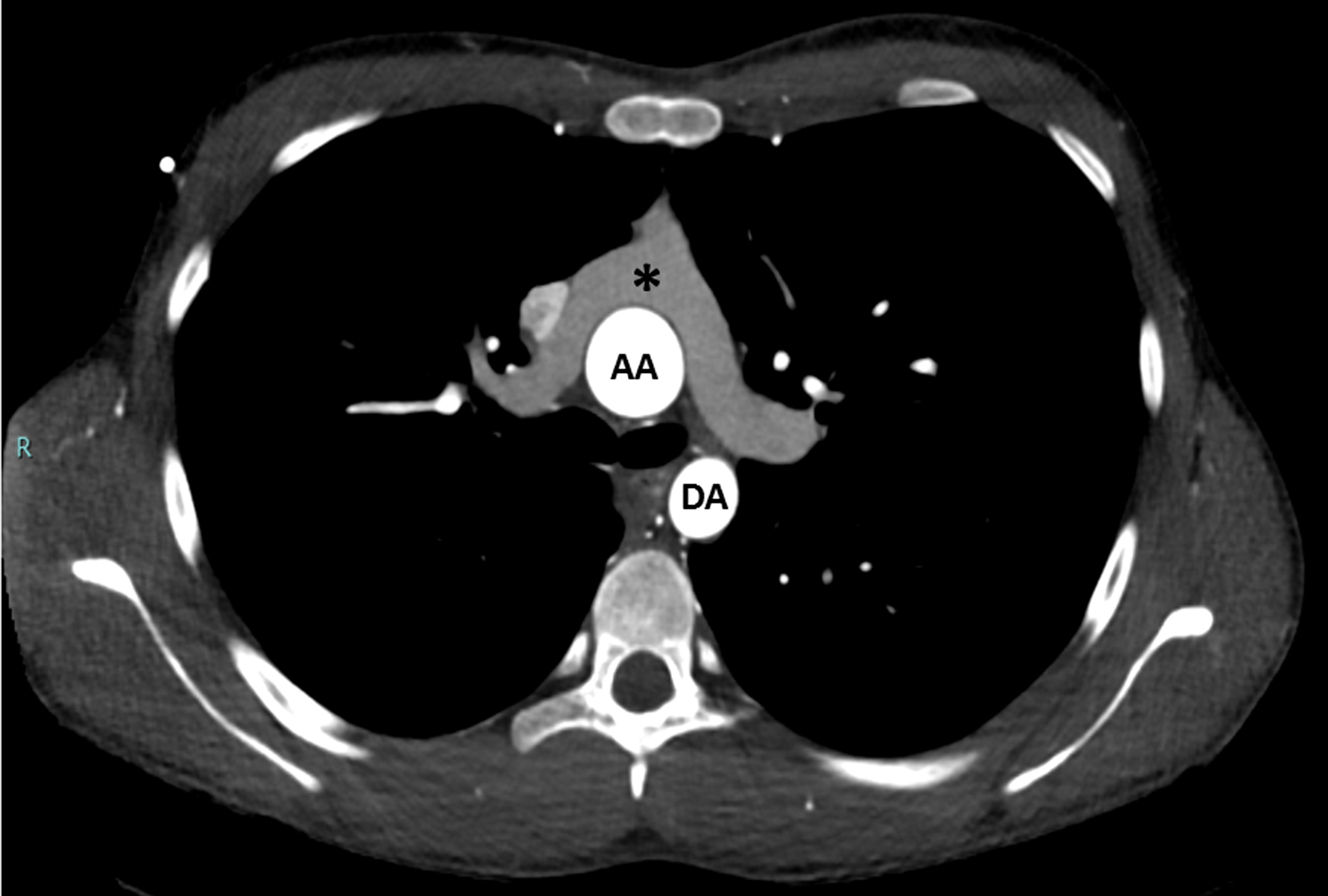
Bolus tracking methods, in which a region of interest is placed over the pulmonary outflow tract to trigger the start of scanning when attenuation within the region of interest reaches more than 150 Hounsfield units, are preferred. , The use of a weight-based empiric scan delay method (starting scan approximately 12–25 seconds after the end of the intravenous contrast injection) has been found to be associated with higher rates of suboptimal pulmonary arterial enhancement, likely owing to wide variation in pediatric heart rates. Shallow free breathing is recommended during CTPA scanning, because pulmonary arterial enhancement is affected by the respiratory cycle. Inspiratory scanning often results in increased dilution from increased venous return (owing to negative intrathoracic pressure during inspiration) and decreased pulmonary blood flow in full inspiration. , ,
CTPA technique should be adapted for patients with congenital heart disease who have undergone the Fontan procedure, especially with bidirectional Glenn shunt, given the very sluggish flow within the Fontan pathway ( Fig. 3 ). Simultaneous intravenous contrast injection from both the upper and lower extremities can help to decrease suboptimal enhancement and false positives related to a mixing artifact. Furthermore, the use of a second delayed scan and bolus tracking may also help to optimize the enhancement of the pulmonary arteries in this setting.
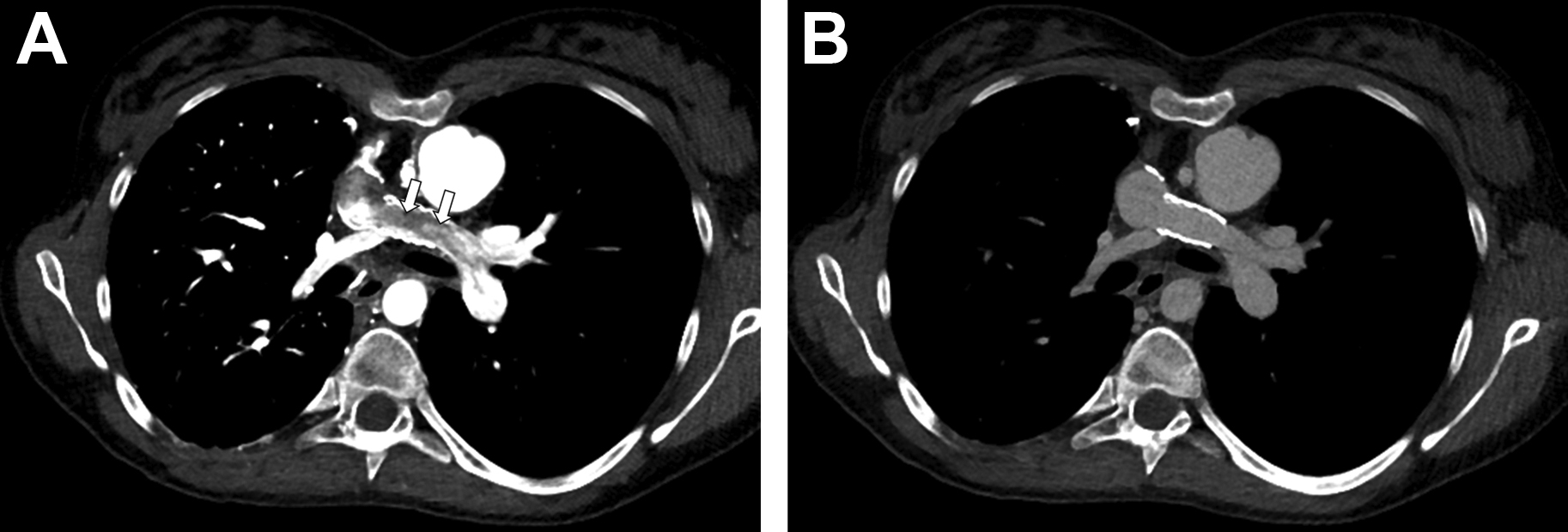
Ideally, imaging review of CTPA images for PE evaluation is performed with an organized search pattern, with a window width of 700 and level of 200. Additional postprocessing techniques, especially the use of sagittal and coronal 2-dimensional reformats and 3-dimensional (3D) vascular reconstructions, improve diagnostic accuracy.
Postprocessing Techniques
Multiplanar Reformation Imaging
Using the initial thin-slice axial data, multiplanar reformation imaging and maximal intensity projection imaging can be used to help increase the diagnostic yield of CTPA. In both children and adults, the use of sagittal and coronal multiplanar reformats and 3D reconstruction of the pulmonary vasculature has demonstrated a significant increase in detection and interobserver agreement for the detection of PE. , One inherent drawback to this method is the increased interpretation time of the study.
Maximum Intensity Projection Imaging
Maximum intensity projection reconstruction improves angiographic imaging detection of vascular caliber, focal narrowing, and filling defects. This volume-rendering technique is broadly used and involves displaying the highest attenuation voxel within an algorithmically selected ray. Slab editing increases the utility of MIP images by removing other high-attenuation voxels not of interest, such as calcifications, bones, surgical material, and so on.
Dual Energy Computed Tomography Applcation
Dual-energy CT is a novel imaging technique that combines functional and high-resolution anatomic information in a single scan by exploiting the unique attenuation properties (photoelectric absorption and Compton scattering) of tissues at different energies (80 kVp and 140 kVp). , , Specifically, iodine perfusion maps are created in a postprocessing technique, which differentiates lung parenchyma perfusion from iodinated contrast (ideally, within pulmonary arteries). However, the timing of the scan should be optimized for the enhancement of the pulmonary arteries, which may not match the timing of maximal lung perfusion. Dual energy CT lung perfusion abnormalities have been shown to correlate well with nuclear medicine scans (planar scintigraphy, single-photon emission CT [SPECT], and SPECT/CT), which remain the gold standard for lung perfusion imaging. , A dual-energy CT scan has been shown to increase the detection of peripheral PE, compared with conventional CTPA, in a canine model.
There has yet to be a large role for combination of CT perfusion and ventilation imaging (using xenon) in the diagnosis of PE in children, but this modality has been used in other applications, such as congenital lung malformations, bronchiolitis obliterans, asthma, bronchopleural fistula, and chronic obstructive pulmonary disease.
Artificial intelligence application
There are multiple potential artificial intelligence (AI) applications being developed for the diagnosis of PE in children however, none are available broadly or used in standard clinical practice. Although there has been much discussion of the use of AI in radiology in the popular media, at present, there are very few AI applications used in pediatric radiology in general. One application of AI in pediatric radiology includes the BoneXpert software, which can provide rapid automatic assessment of bone age in hand radiographs according to Greulich and Pyle, also with estimated standard deviation, which is used at some institutions.
There are reported AI-powered algorithms that have been shown in adults to detect PE on CTPA. Weikert and colleagues describe an AI-powered algorithm that correctly identified 215 of 232 examinations positive for PE (sensitivity, 92.7%; 95% confidence interval, 88.3%–95.5%) while correctly identifying 1178 of 1233 examinations negative for PE (specificity, 95.5%; 95% confidence interval, 94.2%–96.6%). The PE detection algorithm was based on 1-mm images in soft tissue reconstruction, a deep convolutional neural network of Resnet architecture, and trained and validated on 28,000 CTPAs from different institutions. The majority of false positives reported were related to contrast flow-related artifacts, pulmonary veins, and lymph nodes. Unfortunately, the ages of these patients were not reported and likely were mostly adults. Currently, there is no significant role for AI applications in the diagnosis of PE in children.
Nuclear medicine study (ventilation and perfusion scan)
Before the adoption of CTPA, nuclear medicine V/Q scans were the primary noninvasive tests for diagnosis of PE in children and adults. However, the relatively low sensitivity and specificity of V/Q scans (planar scintigraphy, SPECT, and SPECT/CT) compared with CTPA, as well as the technically demanding proper inhalation of radiolabeled gas, limits its usefulness in pediatrics. However, there are clinical scenarios in which other imaging modalities, such as CTPA or conventional angiography, may be contraindicated, such as in severe iodinated contrast allergy or acute kidney injury. Nuclear medicine scans remain the gold standard for the noninvasive imaging detection of lung perfusion abnormalities, despite the recent advent of dual-energy CT perfusion scans.
V/Q scans are usually performed using radioisotope-labeled gas for the ventilation imaging, such as an aerosolized Tc-99m DTPA or xenon-133 gas via a nonrebreathing mask. This step is particularly challenging in young children and in other clinical scenarios in which patients cannot follow specific breathing instructions. The perfusion step involves intravenous injection of Tc-99m macroaggregated albumin particles (approximately 0.03 mCi/kg). Approximately 1 in 1000 particles lodges in the pulmonary capillaries (0.1%) during the examination. Concurrent chest radiographs are crucial for correlation with possible areas of mismatch in perfusion and ventilation. V/Q scans are then rated in probability of PE from very low, low, intermediate, or high risk.
In the PIOPED II study, the sensitivity and specificity of V/Q scans in diagnosis of PE (in adults) was 78% and 95%, respectively; however, this report excludes intermediate and low probability scans. The gold standard in these cases was either digital subtraction conventional angiography or CTPA in combination with Wells criteria. In general, V/Q scans, especially when indeterminate or low risk, usually lead to more testing and the majority of scans yield a low or intermediate probability of PE. , Consequently, V/Q scans are only clinically useful if they are normal or demonstrate high probability (85% chance) of PE.
MR Imaging
Noncontrast MR angiography (MRA) and contrast-enhanced MRA are additional noninvasive imaging techniques for diagnosing PE that avoid the use of iodinated contrast and ionizing radiation, and maintain a high sensitivity and specificity. MRA may be a suitable alternative to CTPA in certain clinical settings. MRA is contraindicated in critically ill patients, despite advances in scan times and the potential to move patients out of the magnet for resuscitation. There are also currently limited data to specifically support the use of MRA in children for the diagnosis of PE.
Similar to CTPA, the goal of contrast-enhanced MRA is to adequately opacify the pulmonary arteries to detect filling defects, such as pulmonary emboli. There are various protocols for the MR imaging detection of PE ( Table 2 ), most widely used is an approach by Benson and colleagues using a gadolinium-based contrast agent, gabobenate dimeglumine (Multihance, Bracco Inc, NJ), at 0.1 mmol/kg, diluted with normal saline for total volume of 30 mL, injected at 1.5 mL/s to ensure uniform bolus throughout the pulmonary arteries during the k-space acquisition. After this, proper breath holding and 2-dimensional autocalibrated parallel imaging may be acquired with greater than 3.5× acceleration, shortening acquisition times and breath holds. Similar to the CT bolus tracking technique, autotriggering can be used with rapid 2-dimensional gradient echo technique with a region of interest at the pulmonary outflow tract (using a signal threshold of approximately 20%).
