Acknowledgments
This work was in part supported by a grant from the National Cancer Institute, grant number R01CA269231, and the Sarcoma Foundation of America. We thank Mehdi Khalighi, Dawn Holley, and Kim Halbert from the PET/MRI Metabolic Service Center for their assistance with the acquisition of PET/MRI scans at the Lucas Research Center at Stanford.
Introduction
Children and adolescents with cancer are referred to specific staging tests based on the primary tumor organ of origin and histology. Based on the accumulated evidence and published experience with 18 F-fluorodeoxyglucose ( 18 F-FDG) positron emission tomography (PET)/magnetic resonance imaging (MRI) studies ( ; ; ; ; ), this technology can add value in imaging pediatric lymphomas and sarcomas. These also represent the main cohort of pediatric cancer patients referred to 18 F-FDG PET/computed tomography (CT) ( ; ; ; ). Evidence in patients with other tumors is emerging and will also be discussed. Integrating MRI and 18 F-FDG PET in children with cancer is advantageous allowing local and whole-body (WB) staging in one session. The label “patient convenience” in this context might be underrated. One-stop exams can help decrease patient suffering and psychological distress while maximizing precious time outside the hospital.
Imaging protocol
Patient preparation
All patients must fast for at least 4 h before an 18 F-FDG PET/MRI scan. Directly before the 18 F-FDG radiotracer injection, we confirm that the blood glucose concentration is below 175 mg/dL.
Radiotracer
We typically inject 3 MBq/kg of 18 F-FDG; followed by a radiotracer uptake time of 45 min. Considering the time to place the patient in the PET/MRI scanner and apply the surface coils, the PET scan starts 60 min after injection. The effective dose from the 18 F-FDG injection can be calculated using the age-specific conversion factors published by the International Commission on radiological protection ( ). While the main radiotracer for pediatric PET/MRI is 18 F-FDG, a few alternate radiotracers can be advantageous for specific clinical questions and will be discussed ahead.
PET/MRI protocol
To set up a time-efficient protocol, whole body axial PET acquisition slabs (25 cm axial field-of-view; 3–4 min per slab) are our time-limiting step. Each bed position is subsequently “filled” with suitable MRI sequences. Different investigators suggested a wide variety of T1-and T2-weighted sequences for anatomical coregistration of 18 F-FDG PET data in children ( ; ; ; ; ). Pediatric tumors can be equally well delineated on Gd-enhanced (CE) T1-weighted scans and unenhanced T2-weighted scans ( ). However, intravenous contrast improved vessel and tumor delineation compared to unenhanced T1-weighted scans, especially when faster T1-weighted sequences were applied. We found CE T1-weighted gradient-echo scans particularly useful for accurate tumor measurements and surgical planning. Therefore, our WB staging protocol always includes CE breath-hold Liver Acquisition with Volume Acquisition (LAVA; 3D Fast Spoiled Gradient Echo) sequences for anatomical coregistration of PET data. These CE scans are acquired sequentially or simultaneously, depending on the contrast agent used (see below). In addition, we acquire axial in and out-of-phase T1-weighted LAVA sequences for attenuation correction and diffusion-weighted imaging (DWI) sequences simultaneously with PET. After completing the WB scan, we add an axial periodically rotated overlapping parallel lines with enhanced reconstruction (PROPELLER) sequence through the lungs to screen for lung nodules. Note that both MRI and PET have limited sensitivity for the detection of subcentimeter pulmonary nodules ( ). Finally, we apply a surface coil and add T1-and T2-weighted Fast Spin Echo (FSE) sequences in appropriate orientations for local staging of the primary tumor. PET data are reconstructed using a 3D time of flight iterative ordered subsets expectation-maximization algorithm (24 subsets, three iterations, temporal resolution =400 px, matrix 256 × 256: voxel size 2.8 × 2.8 × 2.8 mm), accounting for attenuation from coils and patient cradle.
MRI contrast agent
The MRI component of a PET/MRI is often enhanced with gadolinium (Gd)-chelates. When using gadolinium chelates, we perform (1) head-to-toe axial PET and DWI images, followed by Gd-injection, and (2) a second series of head-to-toe LAVA sequences. Considering the growing concern about Gd deposition in the brain, we have introduced the iron oxide nanoparticle compound ferumoxytol (Feraheme) as a contrast agent. Ferumoxytol is FDA-approved as an iron supplement for treating anemia in adult patients and can be used “off-label” as a contrast agent for MRI and PET/MRI. Ferumoxytol provides a long-lasting vascular enhancement for the entire duration of a WB scan and, therefore, can be integrated into the protocol (1) above, eliminating the need for Gd (2). Ferumoxytol enhanced MRI added value to 18 F-FDG PET scans by providing anatomical detail and facilitating the differentiation between reconverted hematopoietic marrow and bone marrow metastases ( ). Nanoparticle-enhanced 18 F-FDG PET/MRI might also improve tumor response assessments to immunotherapies ( ; ).
Lymphomas
Clinical background
Lymphomas comprise up to 10% of newly diagnosed cancers in children (0–14 years) and up to 23% of newly diagnosed cancers in adolescents (15–19 years). Lymphomas are classified as Hodgkin (HL) or non-Hodgkin lymphoma (NHL) ( ). HL has an overall incidence of 1.3 per 100,000 cases ( ), is the most commonly diagnosed cancer in adolescents ( ), and is twice as common in boys as in girls aged 0–14 years, (0.8 vs. 0.4 per 100,000 cases, respectively) ( ). Histological classification of HL includes nodular lymphocyte-predominant (5% of HL cases) and classic HL (95% of HL cases). Classic HL is subcategorized as nodular sclerosis, mixed cellularity, lymphocyte depleted, and lymphocyte-rich ( ). In contrast to HL, while the overall incidence rate of NHL is 1.3 per 100,000 cases, NHL has a higher incidence in male children and adolescents (1.8 M vs. 0.8 F per 100,000 cases, respectively) ( ). According to the World Health Organization (WHO) classification, NHL is phenotypically classified as B cell, including Burkitt lymphoma and diffuse large B-cell lymphoma (DLBCL), T cell, and natural killer (NK) cell NHL ( ). The overall survival rate of HL is significantly higher than NHL ( ).
Diagnosis
Early diagnosis and correct staging of lymphoma can improve optimized therapies and thereby maximize survival. HL staging is performed using the modified Ann Arbor staging system ( ), while the staging of NHL is based on the St. Jude classification ( ). The Lugano classification is simplified and applies to both initial staging and subsequent follow-up ( ). Imaging plays a critical role as the disease stage will determine the treatment ( ). 18 F-FDG PET/CT has been established as the standard technique for WB staging of lymphoma ( ). MRI, compared to CT, can potentially detect additional lesions in the case of involvement of bones, bone marrow, and soft tissues. 18 F-FDG-PET helps to identify tumors based on their high 18 F-FDG avidity. This feature resulted in the incorporation of 18 F-FDG-PET/MRI in the staging of lymphoma and can replace bone marrow biopsies in evaluating bone marrow metastases ( ; ).
18F-FDG-PET/MRI
While 18 F-FDG-PET could be combined with both CT and MRI for attenuation correction and anatomical coregistration of radiotracer data, utilizing MRI as an ionizing radiation-free modality can reduce the radiation exposure ( ). In both HL and NHL 18 F-FDG-PET/MRI ( Fig. 6.1 ) has proven to be a reliable and lower radiation dose alternative to 18 F-FDG-PET/CT ( ).
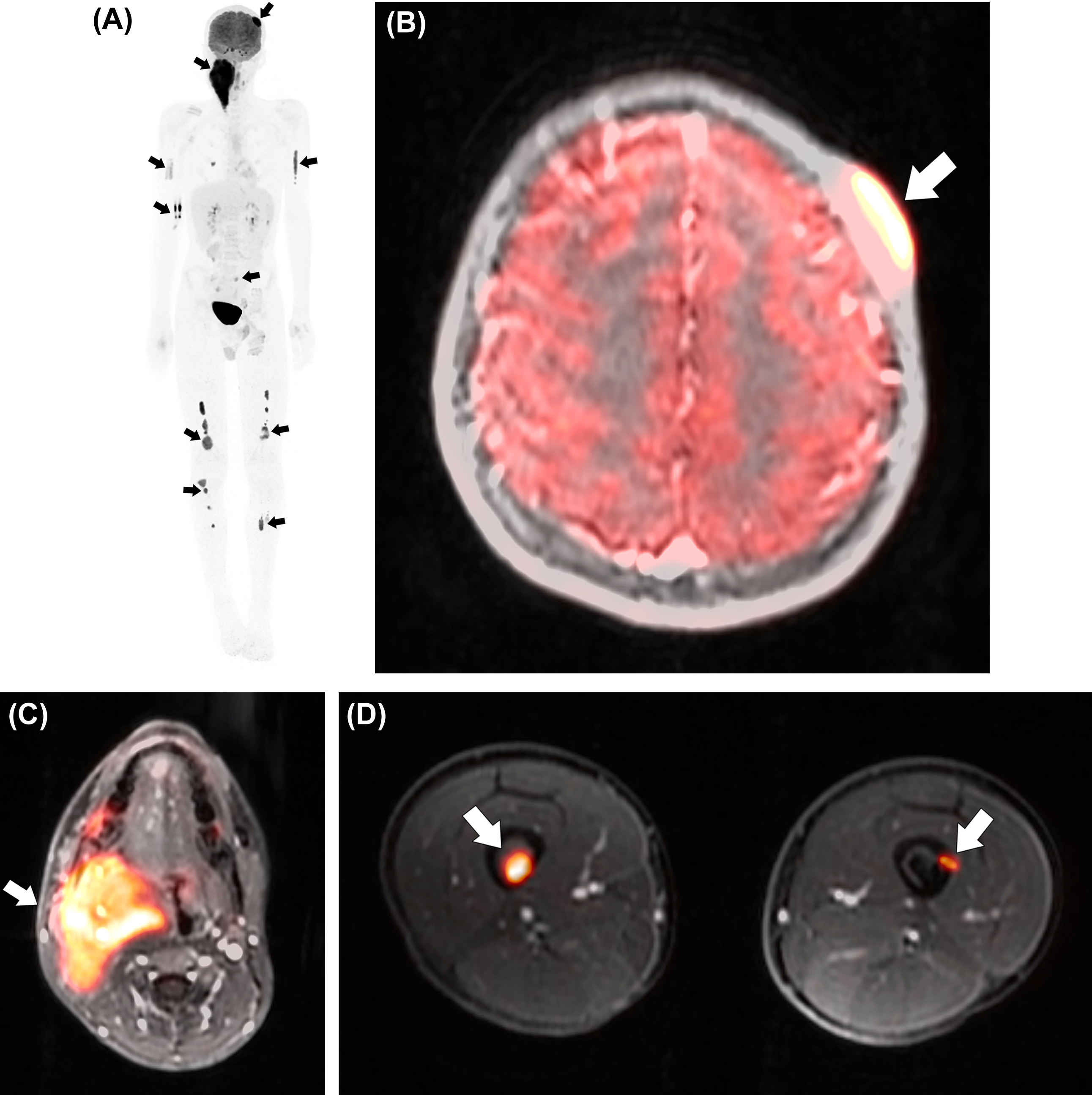
Diffusion-weighted imaging (DWI) has been utilized for the WB staging of lymphoma in adults and children ( ; ). WB DWI could be easily integrated into tumor staging protocols for pediatric lymphoma patients ( ). The addition of DWI improved staging agreement with FDG-PET/CT ( ). Furthermore, in staging pediatric patients with lymphoma, Littooji et al. introduced WB DWI as a radiation-free alternative to FDG-PET/CT ( ). DWI results in higher sensitivity and accuracy in diagnosing specific subtypes of lymphomas, for example, MALT lymphomas, which have variable or no FDG uptake ( ).
DWI could also be utilized to predict clinical response at the end of treatment. Concordant changes of DWI and 18 F-FDG uptake tend to correlate with the response at the end of treatment in pediatric lymphoma patients. Moreover, several patients may demonstrate partial or complete responses also on interim scans ( ). Interim-apparent diffusion coefficient (ADC) can also help recognize nonresponder lesions in HL patients ( ).
Ewing sarcoma
Clinical background
Ewing sarcoma, the second most common bone malignancy in the pediatric population ( ), has an incidence of 1–3 cases per 10 6 children and accounts for approximately 3% of pediatric malignancies. Ewing sarcoma family of tumors comprises classic osseous Ewing sarcoma of the bone, primitive neuroectodermal tumor, and Askin tumor. They all share a common translocation between chromosomes 11 and 22 and contain similar small round blue cells ( ). At diagnosis, 15%–46% of patients present with distant metastases ( ). Despite recent advancements in treatment, the overall prognosis remains poor (5-year survival rate of approximately 25%–30% in metastatic patients).
Diagnosis
Classic osseous Ewing sarcoma can affect any bone but predilects the femur, ilium, tibia, humerus, fibula, ribs, and sacrum. Ewing sarcomas of the long bones are often diaphyseal and demonstrate aggressive permeative destruction with associated soft tissue components. Before cross-sectional imaging, a radiograph of the primary tumor should be obtained. On radiographs, the typical pattern would be that of a nongeographic, permeative, and moth-eaten appearance with a wide zone of transition, usually accompanied by cortical destruction, soft tissue components, and characteristic onion skin or hair on end periosteal reaction ( ). The absence of tumor osteoid helps to differentiate it from osteosarcoma.
18F-FDG-PET/MRI
MRI imaging features closely mirror the radiographic findings: a large permeative soft tissue component extending from the bone marrow through the cortex. The soft tissue component usually demonstrates homogeneous and intermediate T1w signal and homogeneous low to intermediate T2w signal, secondary to the high cellularity of this tumor ( ). Larger lesions can be heterogeneous with central hemorrhage and necrosis. Enhancement may be homogeneous or peripheral and nodular. Spiculated subperiosteal low signal corresponds to the radiographic hair on end periosteal reaction.
On 18 F-FDG-PET, the tumor may show areas of up-regulated glucose metabolism which correlate with its biological aggressiveness. 18 F-FDG-PET can help to decide the areas to biopsy.
Areas of metabolic activity may or may not overlap with areas of diffusion restriction. However, a high degree of overlap is indicative of highly aggressive sarcomas. Besides local staging, 18 F-FDG-PET/MRI also provides excellent WB staging ( Fig. 6.2 ). Metastases are hematogenous and predominantly hit the lungs and bone marrow ( ). FDG-PET combined with MRI is sensitive even for subtle bone marrow metastases. Since osseous metastases in Ewing sarcoma are lytic, PET has better sensitivity in detecting such hypermetabolic lesions than bone scans ( ). One of the limitations of the PET/MRI is identifying subcentimeter pulmonary metastases which are not metabolically active ( ). Mean SUV values of the primary tumor range from 5.3 for patients with no metastases at presentation to 11.3 for patients with metastases at presentation ( ).
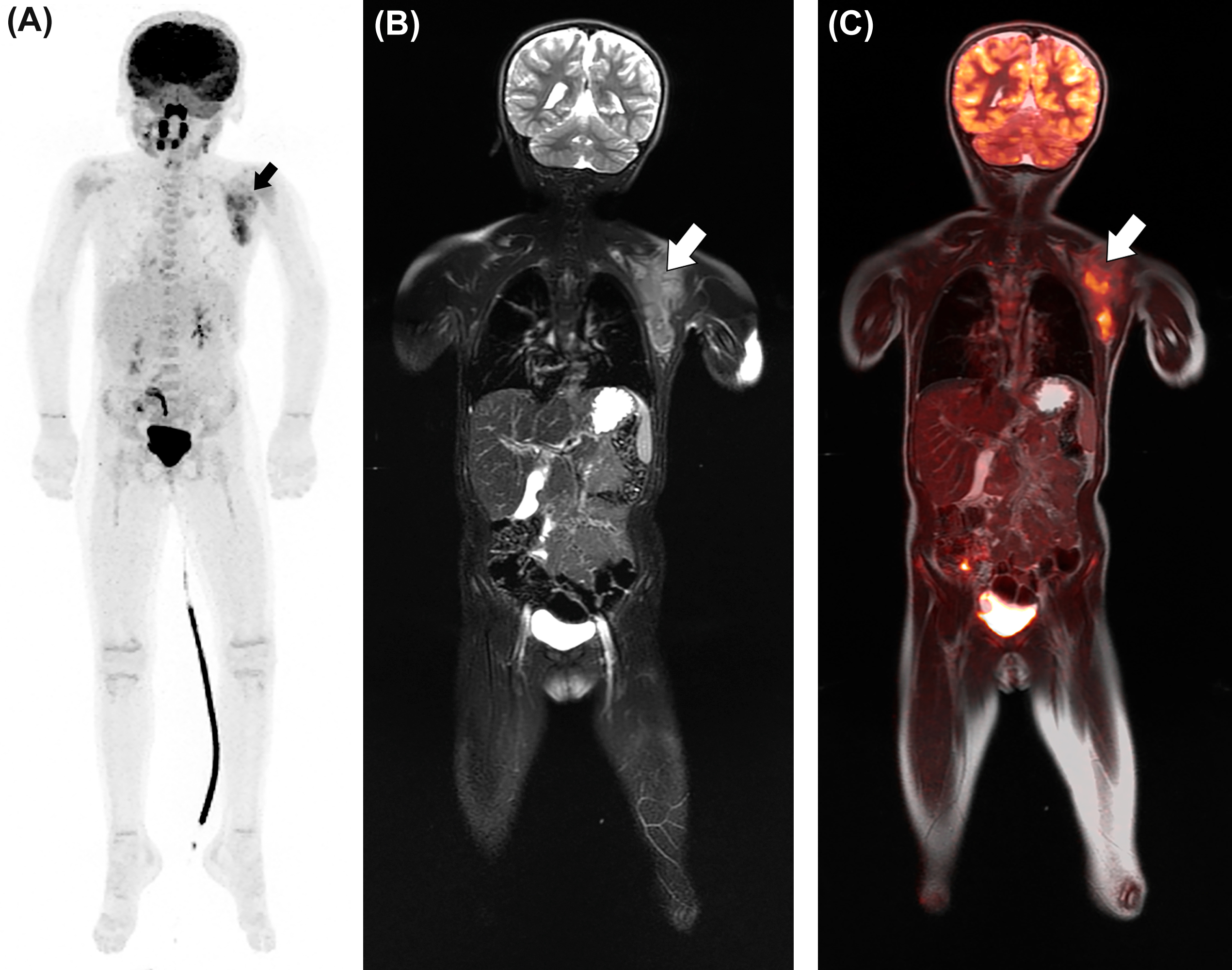
Assessment of treatment response using MRI alone is less accurate since only minimal morphological changes may be evident despite significant tumor viability reduction ( ). FDG-PET allows better detection of progression or response since the biochemical changes in the tumor occur before morphological changes ( ; ).
Osteosarcoma
Clinical background
Osteosarcomas, the most common primary malignant bone tumors in the pediatric population, arise from mesenchymal stem cells or from committed osteoblast precursors ( ). They are characterized by the production of osteoid tumor matrix. According to WHO, they are classified into eight different subtypes: conventional, parosteal, periosteal, telangiectatic, small cell, low-grade central, secondary, and high-grade surface ( ). Other uncommon subtypes include gnathic osteosarcoma and osteosarcomatosis, not included in the WHO classification. This discussion is focused predominantly on conventional osteosarcoma, the most common subtype.
Diagnosis
Common sites of involvement include the metaphysis of the long bones, predominantly of the distal femur, proximal tibia, proximal humerus, and proximal femur. Other bones, such as the pelvis and mandible, can also be involved. Primary osteosarcoma of the wrist and ankle are extremely rare. On the radiographs, it usually presents as a sclerotic, nongeographic aggressive and permeative mass with a sunburnst periosteal reaction ( ).
18F-FDG-PET MRI
Conventional osteosarcoma
Hybrid imaging technique provides excellent sensitivity in assessing local and metastatic disease. Conventional osteosarcomas originate in the center of the medullary cavity. They exhibit a characteristic zoning phenomenon: as the tumor propagates from the medullary cavity to the periphery, the older and mature tumor cells in the medullary cavity ossifies first, followed by the newer peripheral nonossified components of the tumor, which reflect the most actively proliferating part. The latter demonstrates hyperenhancement, diffusion restriction, and FDG uptake; meanwhile, the more mature ossifying central part shows decreased or even absent abnormal metabolic activity and enhancement. This also helps guiding potential sites for biopsy. Occasionally, a characteristic peripheral circumferential “Donut” type of uptake is visualized, which, based on our institutional experience, is associated with a highly aggressive behavior.
Telangiectatic osteosarcoma
This tumor occurs mostly in the metaphysis of the long bones, with the femur being the most common site. As the name suggests, the tumor is composed of telangiectatic elements resulting in characteristic MRI findings, that are an aggressive expansile permeative lesion with multiple blood fluid levels, internal necrosis, and mostly peripheral enhancing soft tissue components. Differentiating this lesion from primary aneurysmal bone cyst (ABC) or secondary ABC is challenging; however, the presence of tumor osteoid in the nonnecrotic peripheral aspect of the tumor on CT or radiographs can help narrow the differential ( ).
Juxtacortical osteosarcoma
Unlike the conventional type, these tumors arise within the periosteum and only secondarily involve the bone marrow ( ). There are three types based on the WHO classification: parosteal, periosteal, and high-grade surface OS.
Parosteal osteosarcoma
This sarcoma originates from the outer fibrous layer of the periosteum and presents as a sessile mushroom-like lesion in the metaphysis of the long bones, characteristically from the posterior aspect of the distal femur ( ; ). On imaging, a lobulated exophytic mass with a central osteoid matrix is seen adjacent to the bone. The lesion has a characteristic “stuck on” appearance and a cleavage plane, separating the mass from the bone marrow (“string sign”) ( ).
Periosteal osteosarcoma
It is predominantly made up of cartilaginous elements, since it arises from the inner germinative layer of the periosteum, thus resulting in high T2w signal ( ). On radiographs, the periosteal reaction perpendicular to the cortex results in a “hair on end” appearance. Medullary involvement is rare.
High-grade surface osteosarcoma
These are usually large at presentation, ranging from 4.5 to 22 cm, and are more aggressive than other surface osteosarcomas, with greater circumferential bone involvement and medullary invasion. Otherwise, imaging features overlap those of periosteal, parosteal, and conventional OS ( ).
Initial staging
Evaluation for potential involvement of adjacent joints, muscles, and neurovascular structures plays a major role for planning local tumor resection. These features are depicted with exquisite details by MRI. It is important to evaluate the adjacent vasculature for tumor thrombosis by assessing flow voids and enhancement patterns. Occasionally, linear FDG uptake along the vessels, corresponding to the tumor thrombus, can be visualized ( Fig. 6.3 ).
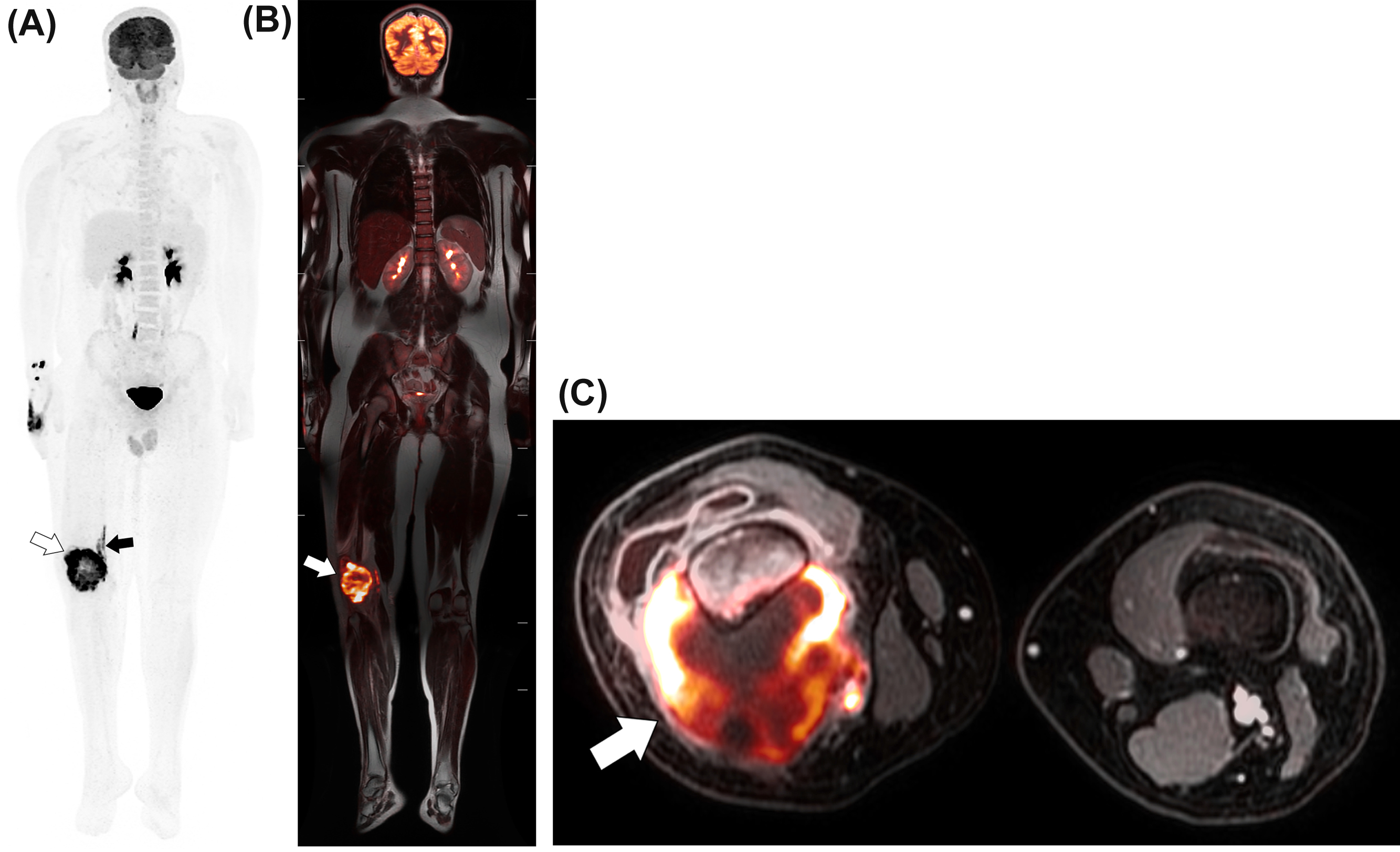
On MRI, skip lesions can be diagnosed as areas of abnormal marrow signal, marrow replacement, or enhancement. Diagnosing skip lesions on MRI becomes challenging in patients with physiologic red marrow changes which show high T2w, low T1w signal, and contrast enhancement. In such instances, FDG-PET can diagnose metabolically avid skip lesions.
Osteosarcomas metastasize early and hematogenously, particularly to bones and lungs. Since PET/MRI is limited in assessing lung metastases, chest CT is included for staging ( ).
Treatment monitoring
Since sarcomas do not demonstrate significant reduction in size posttreatment, PET can provide important prognostic information. Percentage necrosis on histology may used for quantifying treatment response ( ).
Soft tissue sarcomas
Clinical background
Soft tissue sarcomas constitute about 7% of all solid pediatric tumors ( ). Rhabdomyosarcoma is the most common subtype. They arise from the mesenchymal cells and can differentiate and dedifferentiate into multiple cell lineages. A single soft tissue mass can contain more than one histotype; therefore, these lesions are classified according to the predominant component. Moreover, long-standing indolent benign-appearing lesions can differentiate and dedifferentiate into malignant ones; one such example is the low-grade liposarcoma-atypical lipoma spectrum.
Diagnosis
MRI allows the assessment of various morphologic features including size, shape, margins, and internal characteristics, such as necrosis, fibrous tissue, calcium, blood degradation products. Furthermore, DWI can highlight the areas of hypercellularity, and contrast enhanced sequences can highlight the areas of neovascularity and active cellular proliferation. The excellent soft-tissue contrast of MRI is also advantageous to evaluate the local extent of disease, for example, involvement of neurovascular bundles, which could alter the surgical management.
Size, shape, and margins have limited utility in differentiating benign from malignant soft tissue tumors. However some imaging features can help narrow the differential. While some benign entities such as hemangiomas, vascular malformations, lipomas, ganglions, schwannomas, and elastofibromas can be usually diagnosed with confidence based on imaging, in other instances, biopsy is needed.
Although MRI is excellent for the initial diagnosis and staging of soft tissue sarcomas, evaluating previously treated areas becomes challenging. PET is particularly useful in such cases. The combination of the morphological MR details with the metabolic PET data is advantageous ( ). PET/MRI results in higher detection of soft tissue sarcomas compared to MRI or PET alone and is the ideal hybrid imaging tool to assess for recurrence after treatment.
Rhabdomyosarcoma (RMS)
Rhabdomyosarcoma is an aggressive tumor that may arise from almost any part of the body except from bones ( ). It is the most common pediatric soft tissue sarcoma accounting for 4% of the cases. Three major histological subtypes are embryonal, alveolar, and pleomorphic types ( Fig. 6.4 ).
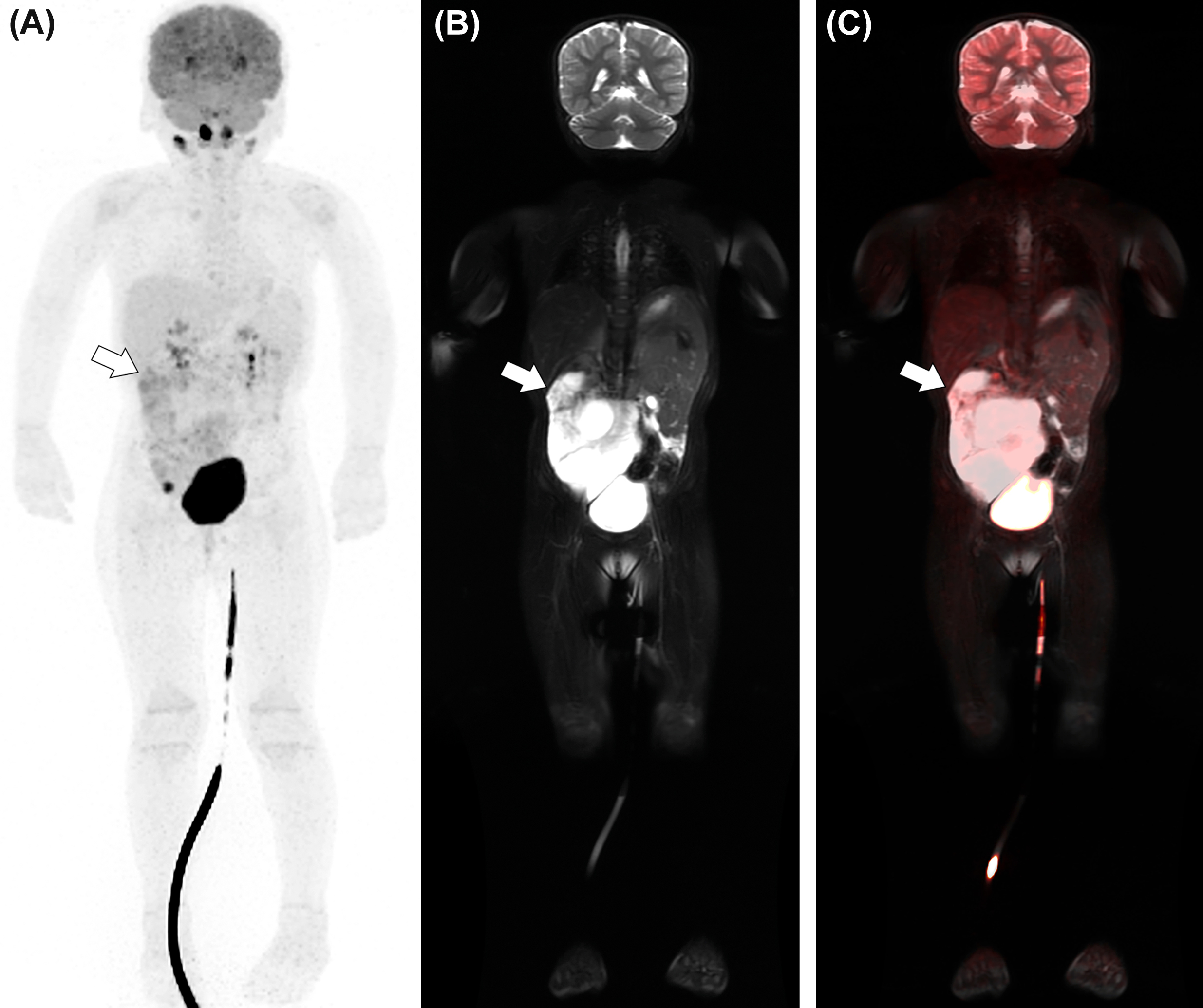
Embryonal RMS
Head and neck are the most common sites of occurrence, followed by the genitourinary organs; other common sites include the retroperitoneum and paratesticular region. Botryoid variant, characterized by a “bunch of grapes” appearance, mostly arises in the pediatric genitourinary tract and biliary tree.
On imaging, they present as heterogeneous masses, due to necrosis and hemorrhage, that are hyperintense on T1w, heterogeneously hyperintense on T2w, and enhance heterogeneously. Owing to the high cellularity, these tumors are diffusion restricted. FDG uptake closely mirrors the contrast enhancement pattern.
Alveolar RMS
They usually painlessly and indolently grow in the deep soft tissues of the extremities and trunk ( ). Imaging findings are similar to those of other soft tissue sarcomas with T1w iso to hyperintensity, heterogeneous T2w hyperintensity, heterogeneous contrast enhancement, and restricted diffusion. They exhibit a very inhomogeneous appearance on postcontrast sequence and PET. Muscles and fasciae are often involved; osseous erosions are common. Alveolar RMS tends to metastasize to regional lymph nodes which can be visualized in both MRI and PET. Some tumors demonstrate overlapping features between DWI and FDG uptake (i.e., tumors with a higher degree of restricted diffusion exhibit higher levels of FDG uptake). The grade of the tumor is directly proportional to the degree of overlap.
Pleomorphic RMS
This is an exceedingly rare high-grade sarcoma composed of undifferentiated pleomorphic cells without embryonal or alveolar components. Imaging features are nonspecific and overlap with other sarcomas. Large mass at presentation and necrosis are common.
Synovial sarcoma
Synovial sarcomas don’t originate from the joints but adjacent to the joints, most commonly around the knee. On MRI, the triple signal (high, intermediate, and low or “bowl of grapes” appearance on T2-weighted sequences) occurs in 35%–57% of synovial sarcomas; it is suggestive but nonspecific of SS since it might also be seen in other neoplasms, such as malignant fibrous histiocytoma. The intermediate signal is due to solid elements, the high signal to hemorrhage or necrosis, and the low signal to calcified or fibrotic components. On T1-weighted sequences, the lesion appears as a heterogeneous soft tissue mass, slightly hyperintense than muscles. Blood-fluid levels can also be visualized. Concurrent evaluation of the radiographs is essential since 30% of them may have eccentric calcifications. Involvement of the adjacent bone is rare ( ).
Desmoplastic small round blue cell tumor (DSRCT)
DSRCT most often occurs in young male patients. The predominant pattern is multifocal bulky nodular omental or peritoneal masses without a definitive organ of origin and occasional calcifications ( ). The primary site is usually in the retro-vesical or retro-uterine region. The tumor tends to mildly enhance and contains areas of necrosis and calcification. It spreads by intraperitoneal seeding. Metastases to lymph nodes, liver, lung, and pleura are also common. The diagnosis is particularly challenging because of imaging overlap with other mesenteric masses such as rhabdomyosarcoma, mesothelioma, intraabdominal desmoid, peritoneal carcinomatosis, and infections, like tuberculosis ( ). Although primarily intraperitoneal, rare cases of widespread disease involving intracranial compartments, salivary glands, breast, ovary, bones, etc., have also been reported ( ).
Fibrosarcoma
Congenital or infantile fibrosarcoma, although histologically identical to the adult form, has different biological behavior and better prognosis. Patients are usually younger than 5 years; this tumor can be discovered even at birth. The most common sites of origin are the proximal extremities, trunk, head, and neck region, and rarely the retroperitoneum. On clinical exam and ultrasound, it usually mimics hemangioma. Internal hemorrhage, central necrosis, and heterogeneous enhancement are present. Although few lesions can show T2w hypointensity due to fibrous components, they are usually T1w isointense, heterogeneously hyperintense on T2w, and enhance heterogeneously ( ). The T2w hyperintensity is due to actively proliferating fibroblasts. This tumor has high local recurrence rates and rare metastatic potential.
Epithelioid sarcoma
Epithelioid sarcomas usually present as a subcutaneous or deep dermal multinodular aggressive masses in the distal extremities, particularly the forearms and hands ( ), extending along the fascial planes with intense perilesional edema. Local recurrence and metastases are common. On MRI, hemorrhage or necrosis may be detected ( ). The factors which predict adverse outcomes are proximal location, large size, depth of infiltration, and presence of hemorrhage, necrosis, vascular invasion, and metastases ( ). There are two types: proximal and conventional, with different histological and clinical features. The proximal type has a predilection for the genitalia.
Alveolar soft part sarcoma
These are rare tumors that comprise less than 1% of all soft tissue sarcomas ( ) and are more common in adolescent girls or young women. They usually present as slowly enlarging or less likely as painful masses in the extremities, mostly in the thigh or buttock. They can also involve the orbit or tongue. Although these lesions are usually small and slow-growing, they have very high metastatic potential given the extreme vascularity and early microinvasion. Approximately 30% of the children present with metastases at the diagnosis, especially in the lungs, brain, and liver ( ; ). Since distant metastases dictate the prognosis, WB PET/MRI can play a crucial role.
Large feeding arteries and draining veins, with early hyperenhancement and delayed washout, are often visualized in ASPS. The delayed washout is likely due to venous thrombi. On the other hand, arteriovenous malformations, given the high flow, account for hyperenhancement, and washout. ASPSs usually have intermediate to high T1w signal, high T2w signal, and vascular flow voids. A peripheral fibrous capsule can also be responsible for low peripheral signal. The most common feature of this tumor is represented by the intratumoral and peritumoral flow voids. Unbalanced p11; q25 translocation resulting in the formation of the ASPL-TFE3 fusion protein is seen exclusively in ASPS and in a few pediatric RCC and peri endothelial epithelioid cell carcinomas.
Liposarcoma
Although traditionally the vast majority of lipomatous neoplasms were thought to be lipomas or liposarcomas, a whole new entity named atypical lipomatous tumors (ALT) or undifferentiated liposarcoma has emerged to clinical attention; ALT are locally aggressive and often do not metastasize ( ). Imaging features that favor ALT over benign lipoma include size greater than 10 cm, septa thicker than 2 mm, nodules, and <75% fat content. On MRI, liposarcomas exhibit increased complexity with enhancing nonlipomatous components. The degree of complexity correlates with higher grades. The enhancing nonlipomatous components are usually metabolically avid on FDG-PET. Liposarcomas can dedifferentiate and contain other sarcomatous elements such as osteosarcoma, chondrosarcoma, etc. Although benign lipomas do not exhibit abnormal metabolic activity, hibernomas, which are benign brown fat tumors, are metabolically active on FDG-PET, causing diagnostic challenges. They exhibit homogenous fatty signal without any complex features on MRI, thus proving the advantages of hybrid imaging.
Soft tissue Ewing sarcoma
Soft tissue Ewing sarcoma is rare and includes extraskeletal Ewing sarcoma/soft tissue primitive neuroectodermal tumor. They fall under the same spectrum of osseous Ewing sarcoma and most commonly present as a large soft tissue mass in the paraspinal region or lower extremity. Askin tumor is a type of extraskeletal Ewing sarcoma of the chest wall, often presenting as a pleural based mass with associated pleural effusion ( ). Local and WB imaging features are similar to classic osseous Ewing sarcoma.
Malignant peripheral nerve sheath tumors
Clinical background
Malignant peripheral nerve sheath tumors (MPNST) are soft tissue sarcomas that arise from perineural cells outside of the central nervous systems. Approximately 50% of MPNST are sporadic, and 50% occur in neurofibromatosis 1 (NF1) patients. MPNST is often associated with chromosomal rearrangements (17q11.2, loss or rearrangement of 10p, 11q, 17q, 22q). In patients with NF1 (mutations of chromosome 17, which encodes the tumor suppressor protein neurofibromin), MPNST often arises from a precursor plexiform neurofibroma. The molecular pathway from neurofibroma to MPNST in patients with NF1 is multifactorial and not fully characterized. Only about 10% of all NF1 patients eventually develop MPNST ( ). Therefore, screening patients with NF1 is typically done with WB MRI, which allows for yearly (or more frequent) scans without radiation exposure. Once an MPNST is suspected, a PET scan is often requested.
Diagnosis
The most common anatomic sites where MPNST can be found include proximal upper extremities, lower extremities, and trunk. MPNST are known to have high metastatic potential and a poor prognosis, with a 50% survival rate in the case of curative surgical resection. In patients with metastasized MPNST, the prognosis is very poor. Therefore, it is of utmost importance to diagnose MPNST at an early stage, before occurrence of metastases. At the same time, surgical excision of benign neurofibromas would cause unnecessary pain and potential iatrogenic morbidities. Therefore, an imaging test with high sensitivity for detecting early MPNST and high specificity for differentiation of benign and malignant tumors is urgently needed for these patients.
Criteria for MPNST
Tumor size, location (trunk, depth of infiltration), degree of necrosis, degree of restricted diffusion, as measured by the ADC, as well as the metabolic activity of the tumor on a standard and delayed 18 F-FDG PET scans can all be used for the differentiation of benign neurofibromas and MPNST. If serial scans are available, then rapid interval growth of a peripheral nerve tumor is also an important feature for malignant transformation.
It is important to note that all quantitative threshold values reported in the literature represent an estimate. Since the evolution from neurofibromas to MPNST is a continuous spectrum rather than a binary process, all threshold values are associated with false positive and false negative rates. In a study, tumor diameter of more than 4.2 cm, loss of the typical target sign (as seen in neurofibromas on T2-weighted images) and ADC min ≤ 1.0 × 10 −3 mm 2 /s provided 100% sensitivity for MPNST (95% CI, 66.4%–100%) ( ). Some MPNST can be surrounded by peritumoral edema, making it difficult to determine the exact borders of the lesion.
Reported standardized uptake values (SUVs), which are suspicious for MPNST, vary from ≥3.5–4.5 on standard 18 F-FDG PET scans ( ). An increasing tumor FDG uptake on delayed 18 F-FDG PET scans can further increase specificity ( ). However, there is no current consensus about the best timing of a delayed scan or the threshold that indicates a significant increase in radiotracer uptake. Suggested intervals between early and delayed scans vary between 1 and 2 h and for positive diagnosis of MPNST suggested increase in radiotracer uptake varies from 10% to 20% ( ). Conversely, a decrease in radiotracer uptake on delayed scans is deemed indicative of a benign lesion ( ). MRI often shows a split-fat sign in benign neurogenic tumors. Only about 50% of benign neurofibromas show the typical target sign. Therefore, the absence of the target sign has limited specificity per se.
Different criteria have been proposed to diagnose MPNST. Some rely on the occurrence of at least two of the following signs: large size, peripheral enhancement, perilesional edema, and intratumoral cystic components, to diagnose MPNST ( ). Others use a decision tree model: superficial tumors and tumors without any necrosis and a SUV max of ≤4.5 are likely benign; deep tumors with any necrosis and a SUV max of ≥4.5 are likely malignant, mandating biopsy and, if positive, resection ( ); deep tumors with ≥25% necrosis with or without a SUV max of ≥4.5 are very likely malignant and should be resected.
Local staging
In patients with localized disease, complete surgical resection with clear margins is critical to achieving survival. Therefore, it is important for the radiologist to tridimensionally evaluate the relationships to adjacent vascular structures, muscles, fasciae, bones, and/or visceral organs. Tumors deep to muscular fasciae tend to have a worse prognosis, similarly to tumors with large size and inability to resect them with clear margins. Metastasized tumors may be addressed by chemotherapy or radiation, although the efficacy of these therapies is limited. While tumor responses are often based on RECIST criteria, it is advised to always provide three-dimensional tumor measurements to ensure that the longest diameter is being recorded. Even in the case of curative resection MPNST, there is a high risk (13%–86%) of local recurrence. Therefore, postsurgical follow-up imaging is necessary.
Whole-body staging
MPNST most commonly metastasizes to the lungs, followed by the bones and pleura. Since the primary tumor is usually evaluated with MRI, WB 18 F-FDG PET/MRI provides a time-efficient and highly accurate approach to also assess for metastases in that same session ( ) ( Fig. 6.5 ). Given the limitation of MRI in detecting small pulmonary nodules, a staging chest CT is needed.
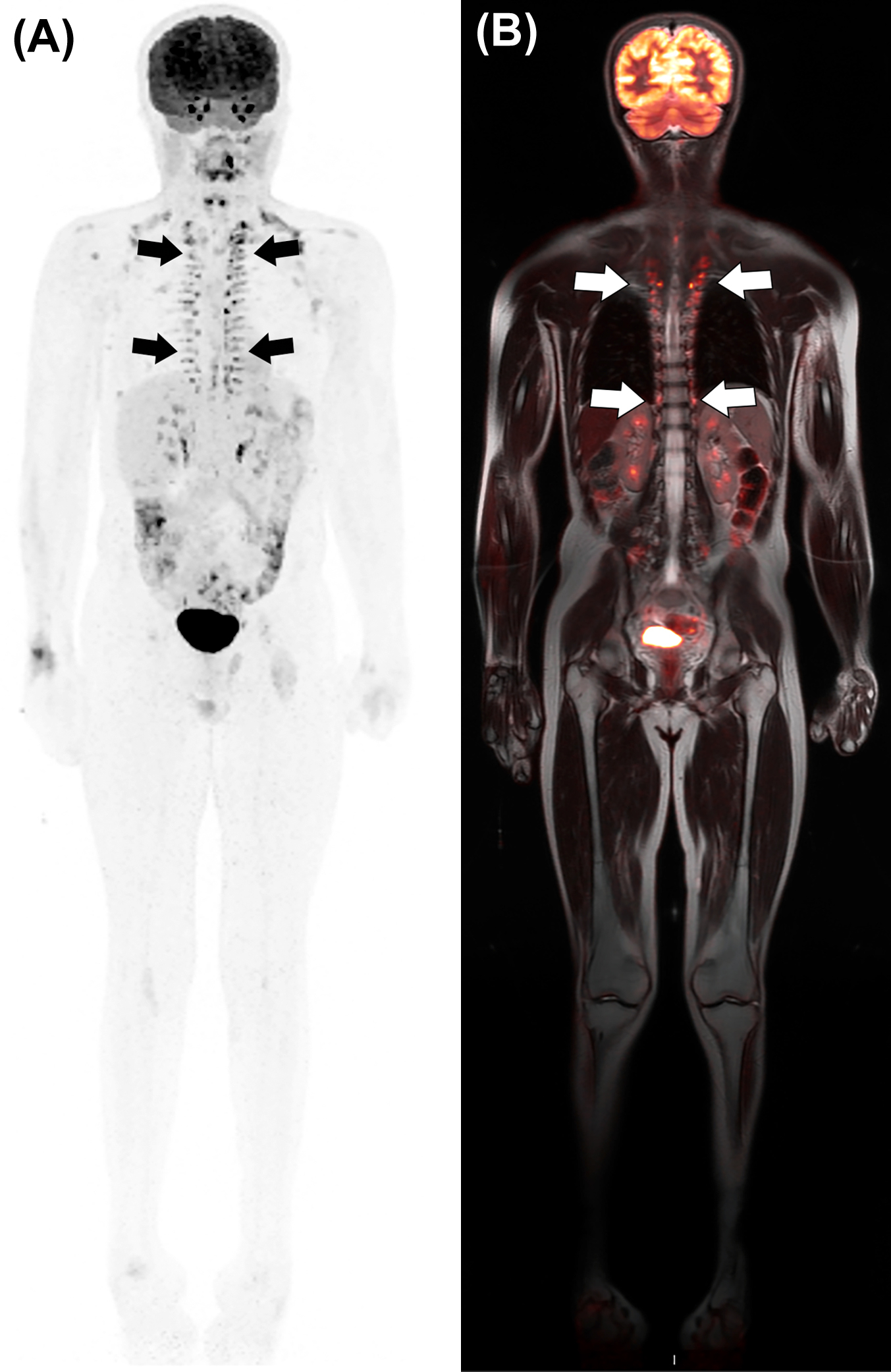
Future developments
Future studies might introduce more specific biomarkers to improve the differentiation between benign and MPNSTs. For example, topoisomerase II α is overexpressed in MPNSTs but not in benign neurofibromas ( ). While imaging topoisomerase II is challenging, recent investigations reported the successful development of fluorescent biomarkers ( ). In addition, the development of both biomarkers and therapeutics that target the mTOR and hsp90 pathways might have significant potentials ( ).
Neuroblastoma
Clinical background
Neuroblastoma is a malignancy originating from aberrant differentiation of the primordial neural crest cells that form the sympathetic nervous system ( ). It is the most common extracranial solid tumor in children worldwide, accounting for about 8%–10% of all childhood cancers ( ; ). Nearly 70% of neuroblastomas occur in the retroperitoneum, especially in the adrenal glands, while the remaining 30% develop in the mediastinum (15%–20%) and other regions (7%–10%) ( ). Prognosis varies from an overall long-term survival rate >90% for low and intermediate-risk patients, to <40% for high-risk patients ( ). Imaging plays a crucial role in risk assessment by defining the extent of the disease, vital organ involvement, and the presence of viable tumor at surgical resection. Complete surgical resection is the cornerstone for localized disease, while combined therapies are necessary for metastatic diseases.
Diagnosis and staging
The diagnosis of neuroblastoma is based on the International Neuroblastoma Staging System (INSS) criteria ( ): positive conventional histology (with or without immunohistology and increased urine or serum catecholamine or metabolites) or, presence of unequivocal tumor cells in the bone marrow and increased urine or serum catecholamines or metabolies ( ). Cross-sectional imaging is used to assess the extent of the disease, especially hepatic, bone marrow, and spinal canal involvement. Iodine 123 ( 123 I) metaiodobenzylguanidine (MIBG) is the first-line functional imaging agent for identifying both the primary tumor and the metastases ( ). One unequivocal MIBG-positive lesion at a distant site is sufficient to define the disease as metastatic. A single equivocal lesion on MIBG requires confirmation by another imaging modality (plain radiographs, and if negative, MRI) and/or biopsy ( ). Bone marrow involvement should be assessed by two aspirates and two biopsies from bilateral sites ( ). A biopsy is not required for infants <6 months ( ; ; ).
Criteria
Over the last 3 decades, the most common staging system for neuroblastoma was INSS ( ; ) which relies on the extent of surgical resection. In 2004, the International Neuroblastoma Risk Group Staging System (INRGSS) developed a pretreatment staging classification based upon image-defined risk factors (IDRFs) at diagnosis. This has been adopted by most cooperative clinical trials ( ; ; ). INRGSS uses 20 IDRFs across multiple organ systems to distinguish local versus invasive tumors and to assess involvement of critical structures ( ). Imaging modalities used to determine IDFR include US, MRI, CT, and scintigraphy. IDRF includes five main groups: vascular encasement, involvement of multiple body compartments, infiltration of adjacent organs and/or structures, airway compression, and intraspinal tumor extension. The IDRF status of the primary tumor should be recorded in all patients (including patients with metastatic disease) so that the impact of IDRFs on surgical resection, surgical complications, and the outcome can be prospectively evaluated ( ).
123I-MIBG versus 18F-FDG
123 I-MIBG is an analog of norepinephrine. Most malignant neuroblastomas and differentiated tumors highly express the norepinephrine transporter (NET) receptors ( ). 123 I-MIBG scan has overall sensitivity of 88%–93% and specificity of 83%–92%; the extent of 123 I-MIBG–positive disease before, during, and after treatment correlates with event-free and overall survival ( ; ; ). MIBG is also a theranostic agent. 131 I-MIBG can be used to treat relapsed 123 I-MIBG-avid tumors (reported response rate 20%–37%) ( ; ; ; ). The role of 18 F-FDG PET in neuroblastoma is still under evaluation. Most neuroblastomas show elevated 18 F-FDG uptake as well. Patients with 18 F-FDG-avid but 123 I-MIBG-negative tumors have significantly lower survival than patients with 18 F-FDG-negative but 123 I-MIBG-avid tumors ( ; ). However, it is not clear yet if there is a correlation between increased FDG activity and lesser 123 I-MIBG uptake and unfavorable histology. 18 F-FDG PET offers many advantages over 123 I-MIBG scan: in addition to superior imaging quality, it requires no patient preparation, shorter scan time (potentially reducing the amount of required anesthesia/sedation), and shorter procedural time (2 h vs. 2-day) ( ). One of the main drawbacks of 18 F-FDG is its limitation in identifying bone marrow infiltration, especially for patients treated with chemotherapy whose bone marrow activation is 18 F-FDG avid. Simultaneous 18 F-FDG PET/MRI might overcome this issue. Currently, the main application of 18 F-FDG PET is PET is for 10% of neuroblastomas that are not MIBG avid ( ).
Future directions
Various PET tracers have been studied over the years. 18 F Fluorodeoxyphenylalanine ( 18 F DOPA) PET/CT has higher accuracy than 123 I-MIBG scan, and holds prognostic value in the evaluation of patients with neuroblastoma ( ; ; ; ; ). 18 F-DOPA-PET outperforms 18 F-FDG-PET for the assessment of possible intracranial lesions given the lack of uptake in normal brain tissue ( ). 18 F-labeled meta-fluorobenzylguanidine ( 18 F-MFBG) has a biodistribution similar to 123 I-MIBG but much faster blood clearance ( ; ; ). 18 F-MFBG PET/CT has higher sensitivity than 123 I-MIBG 93 (122 lesions detected by 18 F-MFBG PET/CT vs. 68 lesions detected by 123 I-MIBG) ( ). 18 F-MFBG-PET/CT also detected all lesions shown by 123 I-MIBG.
Other adrenal gland tumors
Adrenocortical neoplasms are rare endocrine malignancies. While adrenocortical carcinoams and adenomas are distinct entities in adults, in children theres is considerable histopathologic overlap, leading to their classification as adrenocortical neoplasms ( ). Their high hormone secretion capabilities are responsible for clinical manifestations even when small, e.g., precocious puberty in boys or virilization in girls. Bone age is typically markedly accelerated. Pediatric adrenocortical carcinomas tend to be homogeneous if <6 cm and heterogeneous if >6 cm ( ). They are bilateral in 2%–10% of cases and can metastasize to the liver, lungs, or lymph nodes; invasion of the adjacent organs or extension into the renal vein and/or inferior vena cava may be present as well ( ; ). CE CT or MRI is the diagnostic imaging modality of choice for initial staging and follow-up. Hybrid imaging, such as 18 F-FDG PET/MRI, may be used to confirm the diagnosis of malignancy or to evaluate for local recurrence. Adrenocortical neoplasms show higher metabolic activity, but FDG cannot reliably distinguish adrenocortical neoplasms from adrenal metastases ( ).
Neuroendocrine tumors
Clinical background
Neuroendocrine tumors (NETs) are a heterogeneous group of malignancies that arise from neuroendocrine cells. They account for about 0.5% of all newly diagnosed malignancies, with a female predominance of around 2.5:1 Presentation can vary from slow-growing and indolent tumors, incidentally found on imaging, to highly aggressive malignancies with poor prognosis. Twelve percent to 22% of patients are metastatic at presentation ( ; ). The most frequent primary sites are the gastrointestinal tract/pancreas (62%–67%) and the lung (22%–27%). Most NETs are sporadic, but they can also be associated with hereditary syndromes, like multiple endocrine neoplasia type 1 (MEN-1), MEN-2, von Hippel-Lindau (VHL) syndrome, neurofibromatosis, and tuberous sclerosis ( ; ). They can develop in the context of a carcinoid syndrome induced by serotonin hypersecretion from enterochromaffin cells ( ). Tumor markers include urinary 5-hydroxyindole acetic acid (5-HIAA), a serotonin metabolite, and plasma chromogranin A (CgA), a glycoprotein secreted with serotonin ( ; ). CgA levels correlate with tumor bulk, differentiation, and secretory activity, which may predict treatment response and overall survival (fast-rising levels portrend a poor prognosis) ( ; ). Staging is essential since surgery remains the optimal treatment in nonmetastatic disease ( ; ).
Diagnosis and staging
The 2017 WHO NETs classification subdivides NET tumors into three pathologic grades (G1–G3) based on the number of mitoses on high-powered microscopy, the Ki-67 index, and the presence or absence of tumor necrosis and apoptosis ( ). Histopathology characterization is fundamental for diagnosis confirmation and staging. A combination of clinical features and biochemical tests (gastrin, insulin, glucagon, serotonin catabolites) is essential to determine tumor activity. Cross-sectional imaging is based on CT and MRI. Metabolic imaging is based on the somatostatin analogs described in Chapter 2 ( ; ). 68 Ga-DOTATATE imaging is also essential for eligibility assessment of patients who will benefit from radionuclide therapy with 177 Lu-DOTATATE.
18 F-FDG PET/MRI has limited value in well differentiated tumors, but it is useful for assessing tumor aggressiveness and metastases in high grade NETs ( ). A characteristic “flip-flop” phenomenon can be visualized in NETs, that is, low-grade highly differentiated hormone-secreting tumors are usually positive on SSTR-PET and negative on 18 F-FDG PET, and vice versa ( Fig. 6.6 ); this is explained by decreased expression of SSTRs in aggressive and poorly differentiated tumors ( ).
