This article is a primer on the pathophysiology and clinical evaluation of peripheral neuropathy for the radiologist. Magnetic resonance neurography has utility in the diagnosis of many focal peripheral nerve lesions. When combined with history, examination, electrophysiology, and laboratory data, future advancements in high-field magnetic resonance neurography may play an increasingly important role in the evaluation of patients with peripheral neuropathy.
Key points
- •
Distal symmetric sensorimotor polyneuropathy due to “dying-back” axonal degeneration is the most common form of polyneuropathy and is typically caused by a toxic/metabolic condition, such as diabetes.
- •
Electromyography and nerve conduction study (EMG/NCS) is an extremely useful test in determining the localization (anatomic and nerve fiber type), pathophysiology (axonal or demyelinating), acuity, and severity of neuropathies.
- •
Axonal neuropathies typically demonstrate decreased amplitude action potentials on NCS and neurogenic motor units on EMG.
- •
Demyelinating neuropathies show decreased conduction velocity, temporal dispersion, and prolonged distal and F-wave latencies.
- •
MR neurography plays an important role in the evaluation of proximal, focal nerve lesions that are difficult to evaluate by EMG/NCS.
Introduction
Although neuroimaging has been used routinely to help diagnose focal nerve lesions such as trauma and tumors for years, the utility of high-resolution MR neurography in the evaluation of multifocal and systemic polyneuropathies is just now being investigated. For the neurologist, the anatomic distribution, temporal progression, and electrophysiological properties of neuropathies guide the differential diagnosis, workup, and management of most forms of peripheral neuropathy; at present, MR neurography is not part of the standard workup for patients with peripheral neuropathy. For example, both Charcot-Marie-Tooth disease and amyloid neuropathy may show diffuse nerve enlargements that may not be readily distinguishable from one another on MR neurography, whereas a nerve conduction study (NCS) will reveal dramatic reduction of conduction velocity in Charcot-Marie-Tooth type 1A, but normal velocity in amyloid neuropathy. As neuroimaging technology improves, novel MR imaging techniques such as molecular imaging may make MR neurography more useful in the evaluation of patients with neuropathy.
In the first part of this article, the way in which neurologists approach peripheral nerve lesions is reviewed, based on the anatomic, pathophysiological, and electrophysiological properties of peripheral nerves. Later in this article, the characteristics of various peripheral nerve lesions are summarized.
Introduction
Although neuroimaging has been used routinely to help diagnose focal nerve lesions such as trauma and tumors for years, the utility of high-resolution MR neurography in the evaluation of multifocal and systemic polyneuropathies is just now being investigated. For the neurologist, the anatomic distribution, temporal progression, and electrophysiological properties of neuropathies guide the differential diagnosis, workup, and management of most forms of peripheral neuropathy; at present, MR neurography is not part of the standard workup for patients with peripheral neuropathy. For example, both Charcot-Marie-Tooth disease and amyloid neuropathy may show diffuse nerve enlargements that may not be readily distinguishable from one another on MR neurography, whereas a nerve conduction study (NCS) will reveal dramatic reduction of conduction velocity in Charcot-Marie-Tooth type 1A, but normal velocity in amyloid neuropathy. As neuroimaging technology improves, novel MR imaging techniques such as molecular imaging may make MR neurography more useful in the evaluation of patients with neuropathy.
In the first part of this article, the way in which neurologists approach peripheral nerve lesions is reviewed, based on the anatomic, pathophysiological, and electrophysiological properties of peripheral nerves. Later in this article, the characteristics of various peripheral nerve lesions are summarized.
Basic structure of peripheral nervous system
The peripheral nervous system refers to the part of the nervous system outside of the brain and spinal cord. Functionally, peripheral nerves are categorized into motor, sensory, and autonomic nerves. The cell bodies (soma) of motor neurons reside in the ventral gray matter of the spinal cord and are called anterior horn cells. Motor fibers often have very long axons that extend all the way to the neuromuscular junction. A motor unit consists of an anterior horn cell, its motor axon, and all the muscle fibers it innervates, forming a synapse at the neuromuscular junction. Sensory neurons are bipolar with an afferent axon receiving sensory input from the periphery and an efferent axon entering the central nervous system via the dorsal root. The cell body of the sensory neuron resides in the dorsal root ganglion or one of the sensory ganglia of sensory cranial nerves. The autonomic nervous system is classified into sympathetic and parasympathetic nerves. The neurons of sympathetic nerves are located in the lateral horn of the spinal cord from T1 to L2, whereas the neurons of parasympathetic nerves are located in the brain stem and sacral spinal cord (S2, S3, and S4).
Peripheral nerves have multiple layers of connective tissue surrounding axons; epineurium contains blood vessels and other connective tissues that surround multiple fascicles of nerves ( Fig. 1 A). Each fascicle is encased in perineurial connective tissue. Inside of each fascicle, individual myelinated and/or unmyelinated axons are surrounded by endoneurial connective tissue. Blood vessels (vasa vasorum) and nerves (nervi nervorum) are also contained within the nerve.
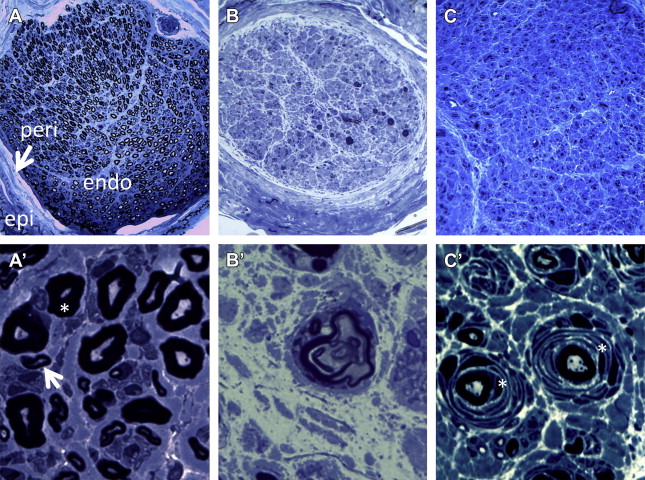
Pathophysiology of peripheral nerve injury
Regardless of its cause and nature of injury, peripheral nerve reaction to injury is limited to certain types of physiologic changes, depending on the extent of injury ( Table 1 ). Minor, local insult to a peripheral nerve will result in a transient, focal conduction block, whereas intermediate insult may cause focal demyelination that requires a longer period of time for recovery. If the extent of nerve injury is severe enough to disrupt its axonal contents, a series of physiologic changes known as Wallerian degeneration follow to ensure removal and reformation of the nerve’s damaged portion. The distal, degenerating portion of the axon undergoes stereotyped morphologic changes and is subsequently digested by Schwann cells to pave the way for regenerating axons from the proximal portion.
Insult | Physiologic Status | Etiology | Electrophysiology |
---|---|---|---|
Minimal | Conduction block, rapidly reversible | Focal ischemia, mild compression | Focal conduction block |
Intermediate | Conduction block, prolonged | Focal demyelination | Focal conduction block and slowing |
Severe | Wallerian degeneration | Loss of axon and myelin sheath | Absent response |
Immediately after nerve transection, there are microstructural changes in the distal portion of the axon without any gross light microscopic abnormalities until 48 hours. During this period, a nerve conduction study of the distal portion will show only a mild decrease in amplitude and nearly normal conduction velocity. At about 48 to 72 hours, the axons begin to fragment and form spiral or hooklike segments; in a cross-section of a nerve biopsy, this fragmentation will appear as “myelin ovoids” (see Fig. 1 B′). At 7 days, there is a complete absence of axon organelles. By this time, there is significant reduction or absence of motor/sensory responses in nerve conduction studies. Considering the length of the entire axon, how these reactions occur in a concerted way within a relatively short period of time remains unknown. In the case of toxic or metabolic neuropathy where the entire nerve fiber, from cell body to neuromuscular junction, is affected, retrograde degeneration appears to take place, possibly due to an insufficient supply of energy or other resource from the soma. This phenomenon is also called “dying-back” neuropathy and typically affects the longest nerves first, thus causing symptoms initially in the feet.
Peripheral nerve injury is often classified into 3 basic categories based on its cause, histologic features, and clinical manifestations: neuropraxia, axonotmesis, and neurotmesis ( Table 2 ). Prognosis following trauma is poor if there is loss of continuity of the endoneurial tube.
Classification | Physiology | Histology | Prognosis |
---|---|---|---|
Neuropraxia | Focal conduction block | Local myelin injury; no axonal injury | Recovery in weeks to months |
Axonotmesis | Loss of nerve conduction at injury site and distally | Loss of continuity of axon, but endoneurial tube, perineurium, and epineurium are intact | Good |
Loss of continuity of axon and endoneurial tube, but perineurium and epineurium are intact | Poor | ||
Neurotmesis | Loss of nerve conduction at injury site and distally | Severance of entire nerve | Regeneration only possible if distal stump reconnected |
Following nerve transection, the regeneration process begins at the distal end of the proximal stump. By 3 to 8 days after injury, small clublike branches will appear at the terminal axon. This distal extension is called a “growth cone.” By 48 hours, only a few of these collaterals reach the zone of injury. In optimal situations, it may take 8 to 15 days for growing axons to reach the distal portion. However, the closer to the cell body the site of injury is, the faster the rate of growth. The ability of nerves to regenerate depends on maintenance of the endoneurial tube, which practically speaking, depends on the severity of nerve injury and length that the axon needs to regenerate. After approximately 9 to 20 days, the regenerating axon remyelinates; however, it should be noted that the myelination of the regenerating axon is often incomplete and has shorter internodal distances compared with the preinjured axon, delaying the propagation of action potentials.
Electrophysiology in peripheral neuropathy
Nerve Conduction Study
Peripheral neuropathy can be divided into those that primarily affect axons and those that primarily affect the myelin sheath. Primary axon loss may be seen after trauma to the nerve or as a result of toxic, ischemic, metabolic, or genetic conditions. Demyelination may be seen in compressive neuropathies, hereditary neuropathies, and acquired immune-mediated neuropathies like Guillain-Barré syndrome (GBS) and chronic inflammatory demyelinating polyneuropathy (CIDP). Nerve conduction studies provide information to differentiate primary axon loss lesion from a primary demyelinating lesion.
Axon Loss
Amplitude of compound muscle action potential (CMAP) correlates with the number of motor nerve axons, and similarly, the amplitude of the sensory nerve action potential (SNAP) reflects the number of sensory nerve axons. Lesions causing axon loss generally result in reduced CMAP and SNAP amplitudes. It is important to keep in mind, however, that secondary axonal loss often occurs in severe or chronic demyelinating lesions. Furthermore, in axonal neuropathies, mild slowing of conduction velocity and prolongation of the distal latency (measure of distal conduction velocity) may occur if the fastest and largest axons are lost.
Demyelination
Loss of myelin is associated with slowing of conduction velocity (slower than 75% of the lower limit of normal), marked prolongation of distal latency (longer than 130% of the upper limit of normal), or both. Amplitude changes can also occur with demyelination because of secondary axonal loss. Reduced motor amplitude may also occur in demyelination if there is conduction block. In conduction block, the amplitude will be low when the nerve is stimulated proximal to the site of demyelination, but will be normal when stimulated below the block ( Fig. 2 ). Any drop in CMAP amplitude or area of more than 20% implies conduction block and any increase in the CMAP duration of more than 15% signifies temporal dispersion; both are hallmarks of demyelination. In patients with demyelinating polyneuropathies, conduction block at nonentrapment sites helps to differentiate acquired (GBS and CIDP) from inherited neuropathies (Charcot-Marie-Tooth disease [CMT]).
Another useful measurement obtained in nerve conduction studies is the “F-wave” response, which is derived by antidromic travel of the action potential up the nerve to the anterior horn cell, which then backfires in a small proportion of anterior horn cells, leading to orthodromic travel back down the nerve, past the stimulation site, to the muscle. The F response measures conduction along the entire length of a nerve and is typically markedly prolonged in demyelinating lesions.
In patients with entrapment neuropathy, the exact entrapment site can be obtained by finding evidence of focal demyelination, either by slowing or by conduction block across the lesion site. Recovery from entrapment may occur quickly over several weeks if the compression is reversible and causes only focal demyelination. In contrast, entrapment causing significant axonal loss, evidenced by marked decrease in motor and sensory amplitudes, will have a longer and less complete recovery.
Electromyography
EMG is the recording of muscle electrical activity at rest (spontaneous) and with exertion (voluntary motor units) with an insertional electrode. The presence of abnormal spontaneous activity (positive sharp waves and fibrillation potentials) suggests active denervation. Analysis of motor unit potentials on needle EMG helps determine the acuity and severity of nerve injury. Long duration, large amplitude, and polyphasic motor unit potentials are seen in chronic axonal neuropathies, because of uninjured motor axons innervating denervated muscle fibers. During muscle contraction, there are 2 ways to increase muscle force: either motor units can increase their firing rate or additional motor units can start firing. Recruitment refers to the ability to add motor units as the firing rate increases. In neuropathic diseases, recruitment is reduced and may be the earliest physiologic sign of nerve injury. Thus, several weeks following a focal traumatic nerve injury, EMG will show abnormal spontaneous activity and reduced recruitment of normal-appearing motor units, whereas several months later, the spontaneous activity will be normal, recruitment will remain reduced, and now the motor units will have prolonged duration and enlarged amplitude. In this way, EMG cannot only help localize nerve lesions, but also can determine the chronicity of the neuropathic process.
Clinical assessment of peripheral nerve injury
When evaluating patients with neuropathy or nerve injury, it is very important to assess the physiologic status of peripheral nerves with nerve conduction studies to correlate their physiology with their clinical symptoms. Once the physiologic status of the nerve injury is assessed, its temporal progression, severity, and anatomic distribution should be carefully determined to reach an accurate diagnosis.
Anatomic Distribution
Localization of nerve lesions is the most important aspect of the neurologic examination. A careful history and examination in conjunction with a thorough understanding of the anatomy of the peripheral nervous system should allow one to localize the lesion. In addition, EMG/NCS can further localize the lesion and aid in understanding the pathophysiology. Table 3 summarizes common types of neuropathies that should be considered according to their characteristic patterns of neurologic findings.
Pattern | Neuropathies that Should be Considered |
---|---|
Symmetric, length-dependent distal weakness with sensory loss (most common type) | Metabolic neuropathies (eg, diabetes), toxic neuropathies, Charcot-Marie-Tooth disease |
Symmetric proximal and distal weakness with sensory loss and areflexia | Inflammatory demyelinating polyneuropathies (CIDP and GBS) |
Multiple mononeuropathies | Vasculitic neuropathy, hereditary neuropathy with liability to pressure palsies |
Asymmetric weakness with intact sensory examination and SNAPs | Motor neuron disease, multifocal motor neuropathy |
Asymmetric weakness with pain in a dermatomal distribution | Radiculopathy |
Sensory ataxia with or without weakness | Sensory neuronopathies from paraneoplastic syndrome, Sjogren syndrome |
Significant autonomic involvement | Amyloidosis, diabetic neuropathy |
Nerve Fiber Type
Motor, sensory, and/or autonomic; large and/or small fibers. Careful attention to patients’ symptomatology and clinical examination often reveals the type of fibers that are involved in disease. Typically, the involvement of motor fibers can cause weakness, fasciculations, or muscle atrophy, whereas sensory involvement causes numbness, tingling, and/or altered perception of pain. Also, the damage of large fibers causes imbalance and reduced vibratory and proprioceptive sensation, whereas small-fiber dysfunction causes decreased pinprick and temperature sensations. Autonomic involvement can result in altered sweating, orthostasis, constipation, urinary retention, or palpitations. Nerve conduction studies can only evaluate large motor and sensory nerve fibers, whereas, if there is selective damage to small fibers, other tests, such as a skin biopsy, are required to evaluate these small unmyelinated nerve fibers.
Pathophysiology: Demyelinating or Axonal
A nerve conduction study is necessary to determine whether a nerve injury is primarily demyelinating, axonal, or both, and is essential in the assessment of peripheral nerve injury. Demyelinating neuropathy characteristically shows a reduction in conduction velocity and prolongation of distal and F-wave latencies, whereas axonal neuropathy shows a reduction in amplitude. In some situations, a nerve biopsy may be considered to evaluate the cause of neuropathy and is most useful in diagnosing vasculitic or amyloid neuropathy and infiltrative neuropathies due to tumor. When possible, the sural or superficial radial sensory nerves are typically resected for pathologic analysis so as to leave minimal neurologic deficit. When other nerves are considered for biopsy, MR neurography is often helpful in determining the optimal biopsy site. Although diagnosis of demyelinating neuropathies (eg, CIDP or CMT) can be aided by biopsy, nerve biopsy is rarely needed for diagnosis.
Severity
In addition to the examination, EMG/NCS is extremely helpful in determining severity of nerve injury. The amplitude of motor and sensory responses is a good measure of the degree of axonal loss and correlates with disability. EMG can assess whether muscles are denervated, and if so, can also determine the acuity and severity of denervation. There are different quantitative methods for measuring severity of nerve injury, often using simple tools such as the Rydel-Seiffer tuning fork, Von Frey monofilaments, and hand-held dynamometer. Various measurement formulas have also been suggested, using a combination of the above quantitative testing results. Among them, total neuropathy score (TNS) is widely used for many systemic neuropathies; its interreliability and intrareliability are well established and are particularly useful in assessing therapeutic responses.
Clinical Course
Careful evaluation of the temporal progression of the patients’ symptoms, when correlated with the examination and physiology, can provide crucial information about the disease process. For example, a toxic or nutritional neuropathy might present with a monophasic course when adequately treated, whereas chronic inflammatory neuropathy can present with a relapsing and remitting course.
Entrapment mononeuropathies
Carpal tunnel syndrome is the most common mononeuropathy and is caused by entrapment of the median nerve as it runs in the carpal tunnel at the wrist. Other common entrapment neuropathies include ulnar neuropathy at the elbow (cubital tunnel syndrome), radial neuropathy at the spiral groove, and peroneal neuropathy at the fibular head. Conditions that predispose to carpal tunnel and cubital tunnel syndrome include occupations that undergo repetitive flexion/contraction of the wrists and elbows, diabetes, obesity, hypothyroidism, arthritis, and underlying peripheral neuropathies leading to nerve hypertrophy. Examination shows sensory with or without motor deficits in the distribution of the peripheral nerve and may show a “positive Tinel’s sign” in which percussion over the site of nerve injury reproduces the patient’s sensory symptoms. Diagnosis is typically made with EMG/NCS, but imaging modalities such as ultrasound and MR neurography are increasingly being used to help surgeons determine cause, severity, prognosis, and treatment of entrapment mononeuropathies.
Neuropathies Associated with Metabolic Disease
Neuropathies associated with metabolic disease typically present with a slowly progressive, distal (length-dependent) symmetric sensorimotor polyneuropathy (DSPN) with physiologic features of axonal loss.
Diabetes Mellitus
Diabetes mellitus is the most common cause of peripheral neuropathy in the United States and Europe. The risk of developing peripheral neuropathy correlates with the duration of diabetes mellitus, glycemic control, and presence of retinopathy and nephropathy. DSPN is by far the most common form of diabetic neuropathy; however, multiple forms of neuropathy are associated with diabetes ( Box 1 ). Nerve conduction studies typically show length-dependent, mixed demyelinating, and axonal polyneuropathy, and this correlates with the nerve biopsy findings of axonal degeneration, regenerative clusters, and segmental demyelination. Autonomic and sensory nerve fibers are prominently involved in diabetic DSPN. Some diabetic patients develop “diabetic amyotrophy,” also known as “diabetic lumbosacral radiculoplexopathy,” which presents with relatively abrupt-onset, severe, asymmetric pain in the proximal thighs, often lasting months. Muscle weakness and atrophy in proximal thigh muscles often develop, although the course of diabetic amyotrophy is monophasic, and patients will usually improve without treatment. Some pathologic studies revealed infiltration of inflammatory cells in various locations in roots and peripheral nerves in patients with diabetic amyotrophy, suggesting an autoimmune cause. However, efficacy of immunotherapy with intravenous immunoglobulin or prednisone is questionable because of its favorable outcome even without any treatment.
Distal symmetric sensory motor polyneuropathy
Autonomic neuropathy
Diabetic polyradiculoneuropathy
- •
Asymmetric, painful lumbosacral radiculoplexopathy (diabetic amyotrophy)
- •
Symmetric, painless, polyradiculopathy
- •
Cervical or thoracic radiculopathy
- •
Focal mononeuropathies
- •
Cranial neuropathy
- •
Entrapment mononeuropathy
- •
Diabetic small-fiber neuropathy
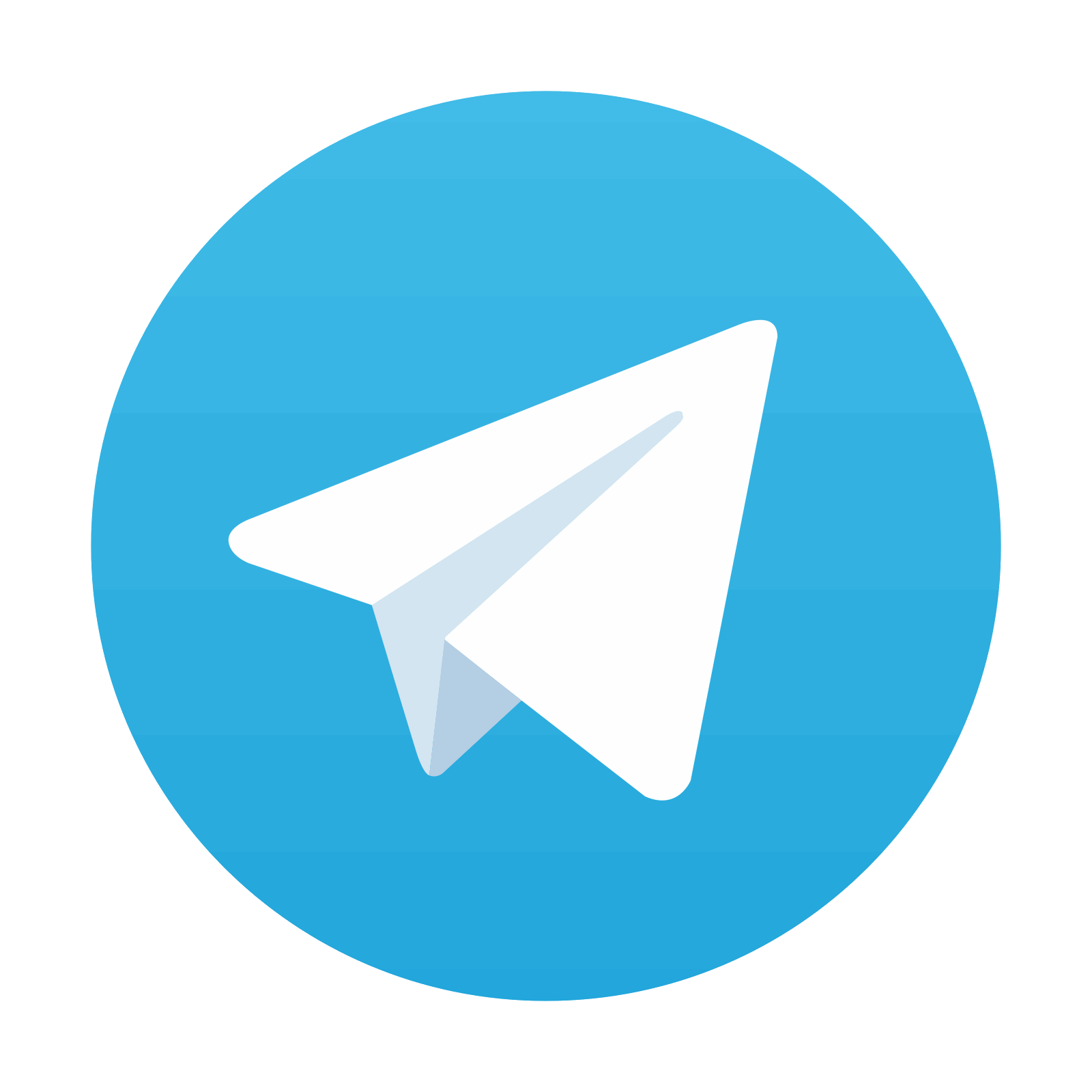
Stay updated, free articles. Join our Telegram channel

Full access? Get Clinical Tree
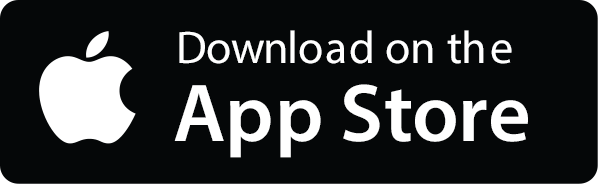
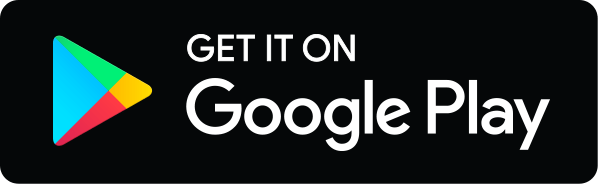
