Fig. 1
T2*-weighted magnetic resonance (MR) imaging of normal rat kidney at 4.7 T, before (a) and 24 h after (b) intravenous infusion of ultrasmall superparamagnetic particles of iron oxide (USPIO). Iron oxide particles are captured by the liver (L) but not by the kidney. C cortex, OM outer medulla, IM inner medulla
1.3 Macrophage Targeting in Experimental Models
Macrophage targeting within the kidney using USPIO was first proposed for a model of nephrotic syndrome, induced by intravenous injection of puromycin aminonucleoside in rats (Hauger et al. 1999). This model induces both lesions of glomerular epithelial cells and a glomerular and tubulointerstitial infiltration by macrophages. MR images showed a diffuse decrease of signal intensity predominantly within the outer medulla 24 h after the IV injection of Sinerem® (Guerbet Group, Aulnay-sous-Bois, France). The degree of signal decrease was correlated with the number of macrophages within each renal compartment and to the amount of iron within the tissue.
A diffuse interstitial macrophagic infiltration of renal parenchyma is a well-documented feature of chronic ureteral obstruction (Schreiner et al. 1988). This cellular influx peaks the second day after the obstruction. Delayed USPIO-enhanced MR imaging demonstrated a diffuse but significant decrease of signal intensity in the three renal compartments, slightly more pronounced in the cortex (Hauger et al. 2000) (Fig. 2).
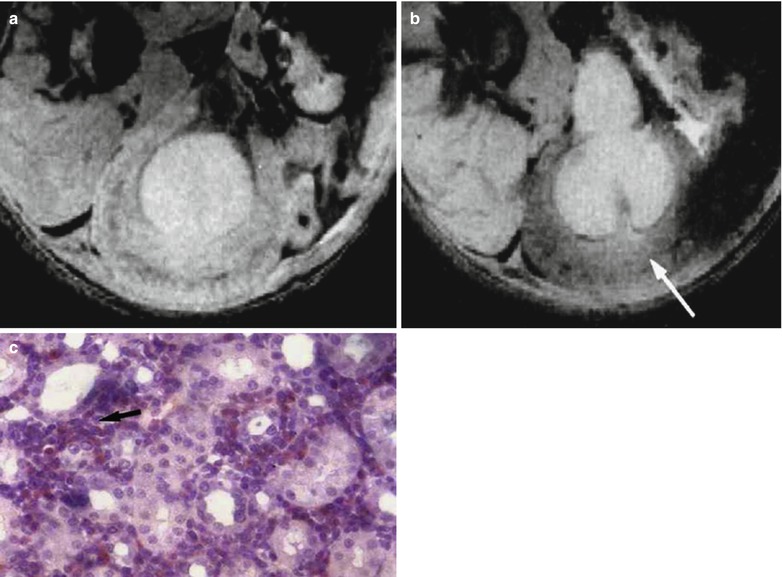
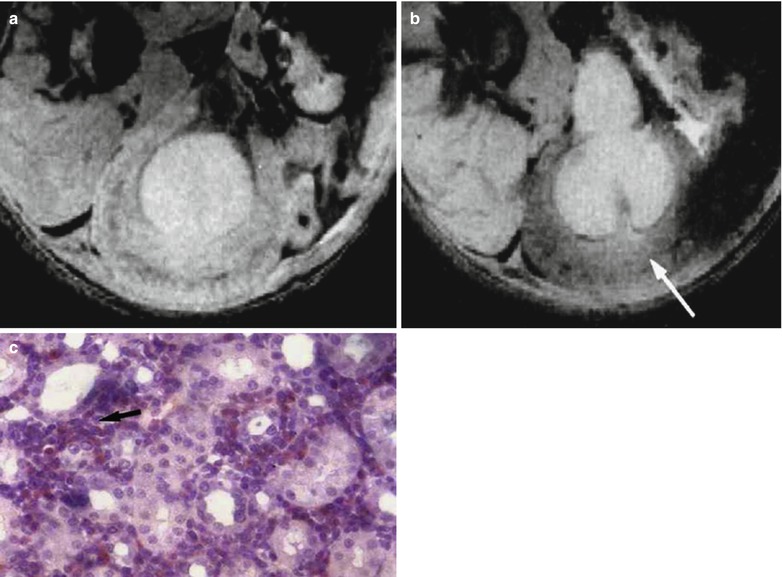
Fig. 2
Obstructive hydronephrosis. T2*-weighted MR imaging of a left obstructed rat kidney at 4.7 T, before (a) and 24 h after (b) intravenous infusion of USPIO. There is a notable decrease in signal intensity (arrow) in all kidney compartments after the injection. Note the dilated renal collecting system. (c) Histological section shows a diffuse infiltration of the interstitium by macrophages (From Hauger et al. (2000), with permission)
In acute proliferative types of GN, such as Goodpasture syndrome or vascular nephritis, macrophages also play a role in the development of glomerular inflammation (Cattell 1994). They accumulate in the Bowman space as a primary feature in the development of advanced cellular crescents which is a known as a feature of rapidly progressive GN which is associated with a poor prognosis. A model of anti-glomerular basal membrane (GBM) GN, comparable to Goodpasture syndrome in humans, was evaluated with MR imaging (Hauger et al. 2000). This model was induced in rats by IV injection of sheep anti-rat-GBM serum. The kinetics of signal intensity followed the biphasic evolution of the disease with a decrease of signal intensity within the cortex only at days 2 and 14 (Fig. 3a) and no change at day 21. This effect was due to endocytosis of USPIO by macrophages at day 14 and by activated mesangial cells at both phases (Fig. 3b). The degree of signal intensity decrease was strongly correlated with the degree of proteinuria.
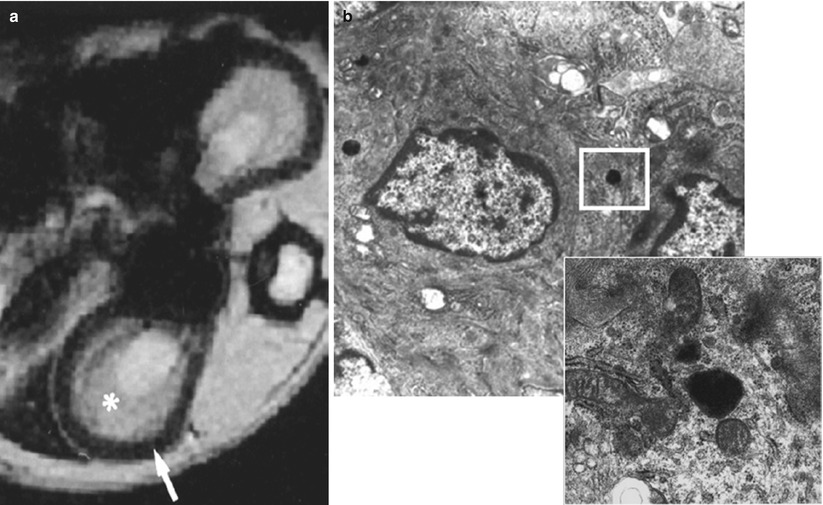
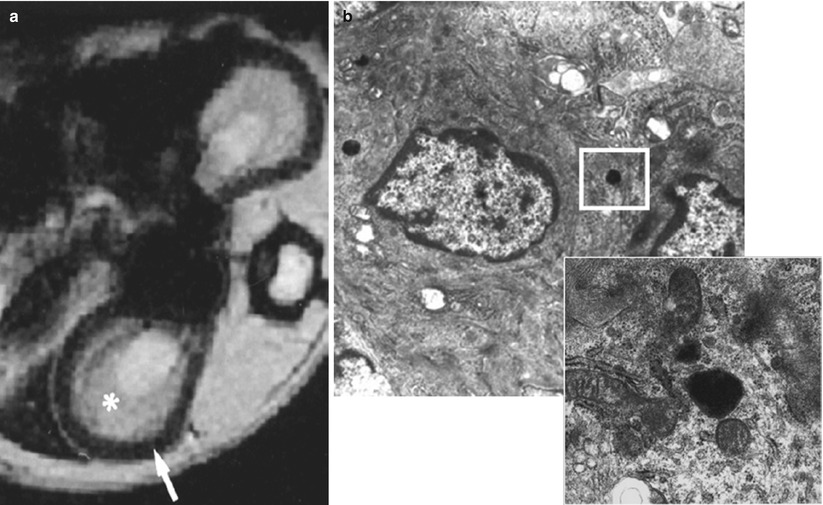
Fig. 3
Nephrotoxic anti-rat-glomerular basal membrane (GBM) glomerulonephritis. (a) T2*-weighted MR imaging at 4.7 T, of rat kidneys in the day 2 pathological group 24 h after the injection of USPIO. There is a notable decrease in signal intensity in the renal cortex (arrow) after the injection, whereas no signal intensity change is observed in any portion of the medulla (asterisk). (b) Electron microscopic image shows the presence of iron particles (see magnification) in a lysosome of a mesangial cell (From Hauger et al. (2000), with permission)
Intrinsic acute renal failure (ARF) is a multifactorial disease with concomitant ischemic, nephrotoxic, and septic components (Devarajan 2006), and ATN is its pathological counterpart. Besides alterations in hemodynamics, tubule dynamics, tubule cell metabolism and structure, the intrarenal inflammatory response plays a major role in the ischemic ARF. Neutrophils are the first leukocytes to accumulate in the postischemic kidney and macrophages are the next. Macrophages migrate into the outer medulla of rat kidneys and accumulate within peritubular capillaries, interstitial space, and even within tubules (Friedewald and Rabb 2004; Ysebaert et al. 2000). In an ischemia-reperfusion model in rats, USPIO-enhanced MR imaging demonstrated the same endocytosis pattern with a decrease of signal intensity within the outer medulla only from 24 to 120 h after the injection of particles (Jo et al. 2003) (Fig. 4). Signal intensity decrease was also correlated with the level of renal function.
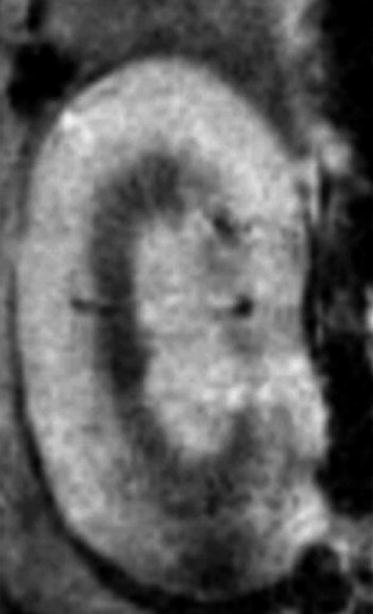
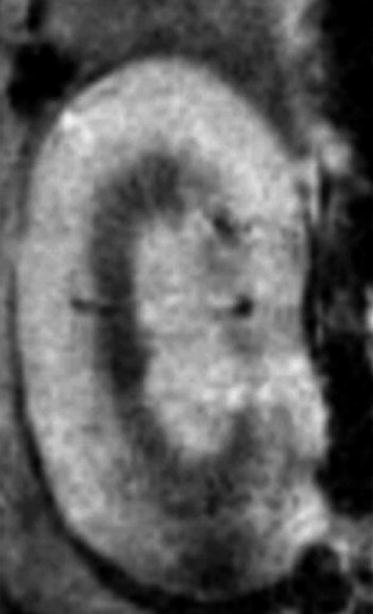
Fig. 4
Ischemia-reperfusion. T2*-weighted gradient-echo MR image 24 h after the injection of ferumodextran-10 USPIO particles in rats subjected to 60 min of bilateral ischemia. A black band in the outer medulla is detected 48 h after the beginning of reperfusion (From Dagher et al. (2003), with permission)
After renal transplantation, acute and chronic rejection episodes play a major role on long-term graft survival and involve T cells and macrophages. Two studies evaluated a rat model of acute rejection and showed a decrease of signal intensity within the cortex and medulla, increasing with iron dosage (Ye et al. 2002) and inhibited by immunosuppressive treatments (Zhang et al. 2000). Beckmann et al. (2006) used SPIO to label macrophagic infiltration in a model of chronic rejection. They showed a dose-dependent decrease in cortical signal intensity in allografts between 8 and 16 weeks after transplantation (Fig. 5). The relative cortical signal intensity in the grafts was negatively correlated to the Banff score 6 weeks after transplantation, and changes in creatinine clearance occurred only after 28 weeks. Groups treated with immunosuppressive and antiproliferative drugs showed lesser degree of signal intensity change (Beckmann et al. 2003).
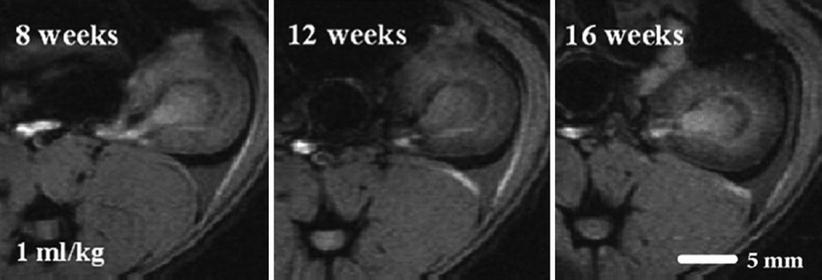
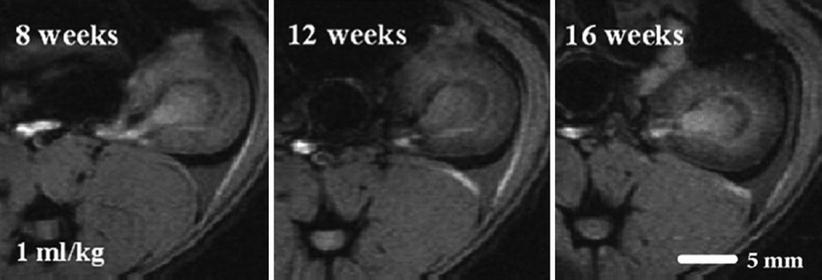
Fig. 5
Gradient-echo MR images of the kidney grafts of recipient rat acquired 8, 12, and 16 weeks after transplantation, 24 h after superparamagnetic particles of iron oxide (SPIO) injection. Darkening of the kidney cortex was apparent at different times after transplantation (From Beckmann et al. (2006), with permission)
All these experimental results showed that USPIO-enhanced MR imaging could demonstrate intrarenal internalization of particles by macrophages or by glomerular cells gaining endocytic activity, i.e., mesangial cells, and could precisely localize this endocytic activity in the different kidney compartments. Different types of experimental renal diseases showed different patterns of signal intensity changes, but the degree of renal dysfunction always appeared correlated to the degree of endocytic activity, which may have significant implications in clinical practice.
1.4 Macrophage Targeting in Humans
These results led to the first pilot clinical study (Hauger et al. 2007), based on 12 patients exhibiting a dysfunction of either their native kidneys or their transplant. As the blood half-life of USPIO is 36 h in humans, MR imaging was performed 3 days after USPIO injection (Sinerem®, Guerbet Group) to ensure avoiding signal changes from vascular blood volume. Patients with chronic and fibrotic disease, without inflammatory component on biopsy, did not show any significant change (Fig. 6a, b). Three patients with ATN (two transplanted kidneys and one native kidney) showed a significant decrease of signal intensity within the medulla only (Fig. 6c, d). All patients but one with an inflammatory component on cortical biopsy showed a significant decrease of signal intensity after USPIO injection (Fig. 6e, f). These preliminary clinical findings seem to corroborate the experimental results, with the same topographic patterns, and call for larger multicenter clinical trials, and the evaluation of imaging at 2 days after injection to reduce delay in the diagnosis.
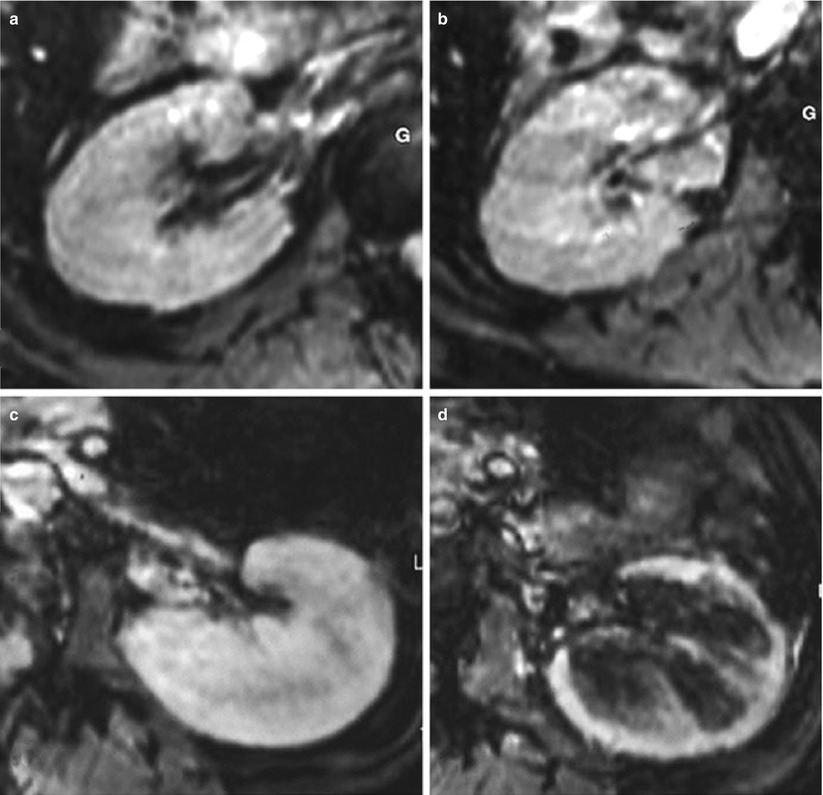
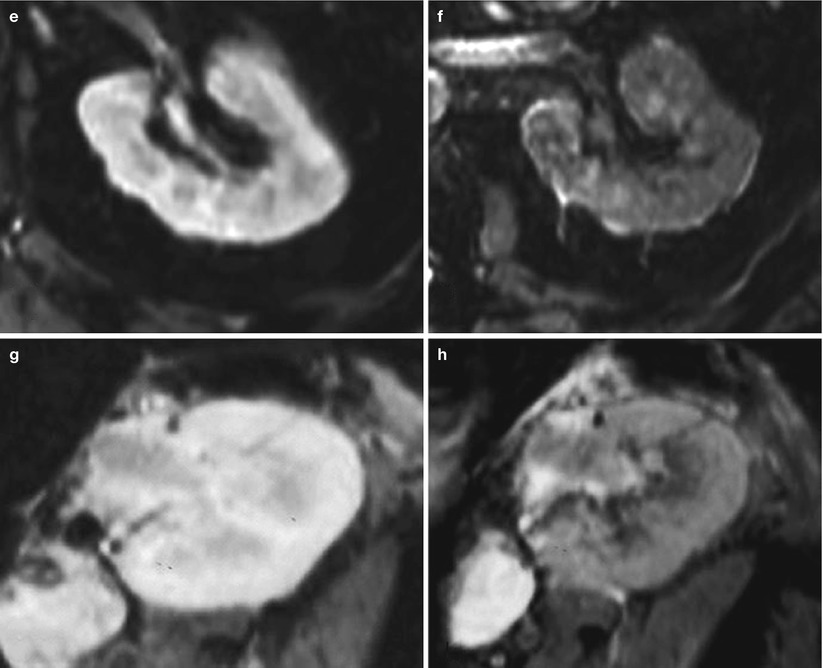
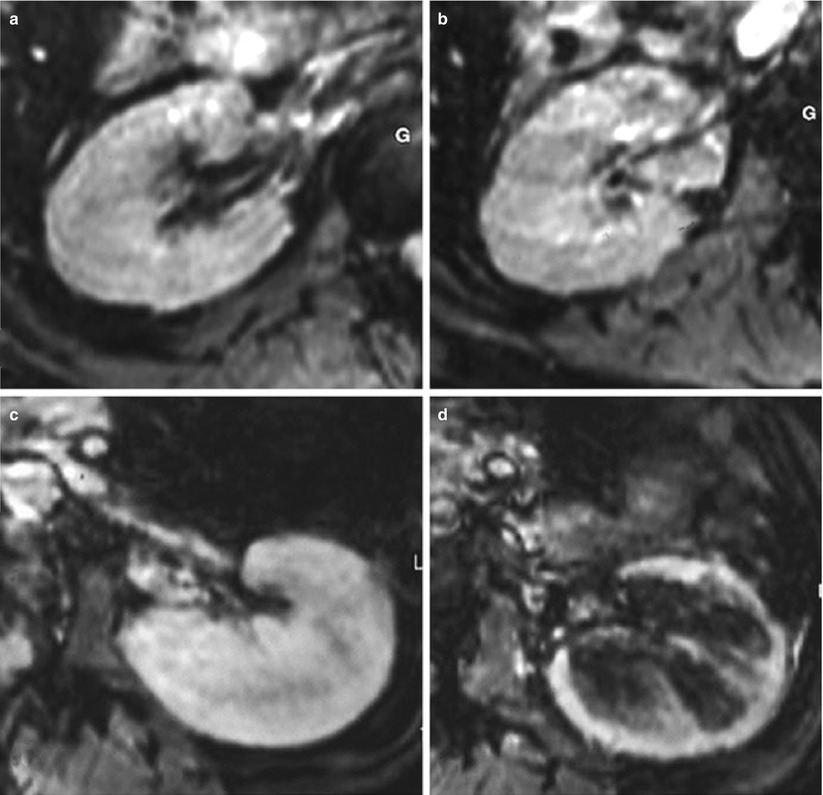
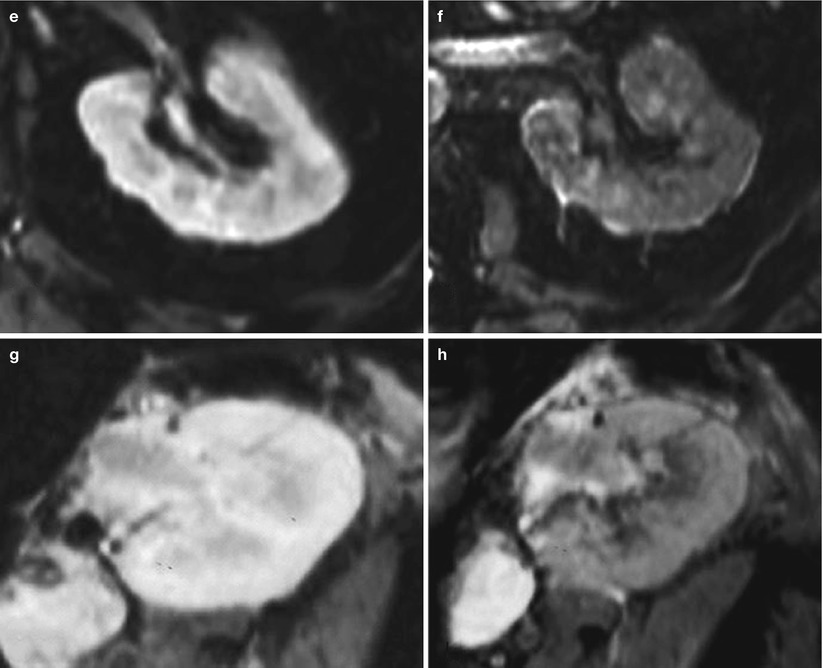
Fig. 6
Examples of USPIO-enhanced MR imaging in four patients presenting with (a, b) a chronic glomerulohyalinosis and glomerulosclerosis, (c, d) a drug-induced acute renal failure (ARF), (e, f) an extracapillary glomerulonephritis with numerous intraglomerular macrophages, and (g, h) a type Ia acute rejection with numerous interstitial macrophages. (a, c, e, g) T2*-weighted MR images before the injection of USPIO. (b, d, f, h) T2*-weighted MR images 72 h after the injection of USPIO (Adapted from Hauger et al. (2007), with permission)
2 MR Imaging of Stem Cell Tracking
Recovery of renal function after acute nephrotoxic or ischemic insult is dependent on the replacement of necrotic tubular cells by functional tubular epithelium (Gupta et al. 2002). This cellular regeneration may originate from renal resident cells or from extrarenal ones. Mesenchymal stem cells (MSCs) have been shown to be able to repair damaged tissues, whether genetically modified or not (Baksh et al. 2004; Pittenger and Martin 2004). They also per se have potential therapeutic effects such as those with regard to the degradation of the extracellular matrix (ECM) in the experimental models of fibrosis (Fang et al. 2004) or the facilitation of the grafting of transplanted bone marrow progenitor cells by providing a competent stroma (Bacigalupo 2004). They have a well-established ability to differentiate into the mesoderm lineage, which makes them potentially useful in strategies aiming at targeting the kidney mesangium for instance.
Recently, the possibility for bone marrow-derived MSC to differentiate into mesangial cells (Imasawa et al. 2001; Ito et al. 2001) and for hematopoietic stem cells into tubular cells was demonstrated in vivo (Lin et al. 2003), bringing great therapeutic promise for the future. Noninvasive imaging techniques allowing in vivo assessment of the location of stem cells could be of great value for experimental studies in which these cells are transplanted. It provides a tool to immediately verify if the grafted cells have reached the target organ, to estimate the number of cells that were seeded, and to assess the permanence of these cells over time with sequential imaging. Using SPIO preparations to magnetically label the cells, several groups have demonstrated the feasibility of grafting and subsequent visualization of the progenitor of different organs (Bulte et al. 1999; Hoehn et al. 2002; Kraitchman et al. 2003). A strong R2* effect was observed in vitro after MSC labeling for 48 h with 50 μg Fe/mL and increased linearly with the iron dose (Bos et al. 2004). After intravascular administration, the renal distribution of SPIO-labeled MSC has been investigated recently. When administered into the renal artery of normal kidneys, labeled MSC could be detected in vivo within the cortex as long as 7 days after injection at 1.5 T (Fig. 7) and iron-loaded cells were identified in renal glomeruli using histology (Bos et al. 2004). The same experiment was repeated at 3 T (Ittrich et al. 2007) with similar results in normal kidneys. In kidneys with acute renal injury (40′ ischemia-reperfusion), the improvement of renal function was accelerated in rats treated by cell graft: lower plasma creatinine levels at days 2 and 3 (Ittrich et al. 2007). In a model of acute experimental glomerulopathy (Thy1 + PAN), a homing effect was identified ex vivo, at 9.4 T, when the magnetically labeled MSCs were administered intravenously. A large proportion of cells were trapped within the liver precluding their detection in the kidneys in vivo at 1.5 T. When comparing the enhancing renal segments in ex vivo images and pathological lesions in histological sections, the areas of low signal intensity correlated well with alpha-actin and Prussian blue stains, indicating that MSCs specifically homed to injured tissues (Hauger et al. 2006) (Fig. 8).
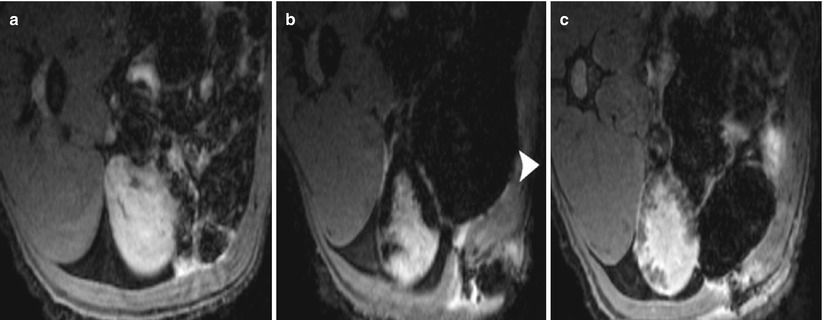
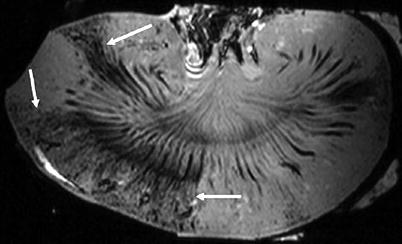
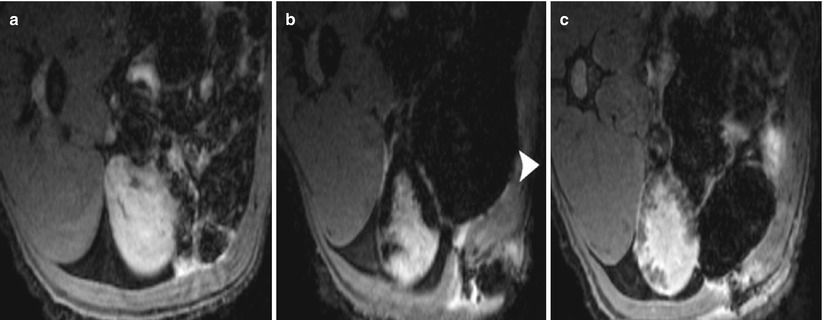
Fig. 7
Renal cell therapy by intra-arterial graft of mesenchymal stem cells (MSCs). Transverse T2*-weighted MR images of rat kidney before (a) and 1 h (b) and 7 days (c) after the injection of SPIO-labeled MSCs into the left renal artery. Arrowhead indicates the ventral side of the animal (From Bos et al. (2004), with permission)
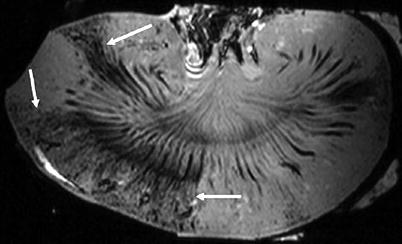
Fig. 8
Renal cell therapy by intravenous graft of MSC. Ex vivo sagittal T2*-weighted 9.4-T MR image of pathological kidney 6 days after intravenous injection of 107 labeled MSCs. Distinct areas of cortical signal intensity decrease are present in the superior and superior midportion (arrows) poles, where labeled MSCs were homed
3 MR Imaging and Monitoring of Gene Therapy
Up to now, MR imaging of transgene expression has been limited to the demonstration of proof-of-principle experiments, using a change of signal intensity under the control of the expression of a reporter gene. As with PET, two approaches have been proposed with MR imaging using either an enzymatic pathway or a receptor pathway. The first method is based on the expression of a reporter gene (Lac-z) coding for an enzyme (β-galactosidase), which is able to activate a Gd-chelate by giving access of water to the first coordination sphere of the Gd ions (Louie et al. 2000). The second method is based on the targeting, with iron oxide particles, of cells overexpressing the transferrin receptors on their membrane (Moore et al. 2001; Weissleder and Mahmood 2001). None of these methods have been applied in the field of urogenital diseases.
MR imaging could also play a role in controlling gene expression in space and in time. The possibility to limit the effect of a therapeutic gene to a target diseased tissue and during a predefined time window is a key challenge for the future of gene therapy. For such a purpose, expression of a transgene can be controlled by a heat-sensitive promoter, such as the promoter of the inducible heat shock protein systems, HSP70 (Rome et al. 2005). These promoters can activate gene expression several thousandfold in response to hyperthermia (Dreano et al. 1986). Heating of the deep parts of the body can be achieved with high-intensity focused ultrasound (HIFU), and temperature control is made possible by using temperature-sensitive MR sequences based on the proton resonance frequency (PRF) method (Quesson et al. 2000) and by using an automatic monitoring of the acoustic power.
This approach has been recently experimented in vivo within the rat kidney. First, modified MSC expressing the luciferase reporter gene under the control of the hsp70B promoter was administered through the left renal artery. Luciferase expression in MR-guided HIFU heated regions was first demonstrated in vitro, using immunostaining (Letavernier et al. 2007). More recently, using modified C6 cells with hsp70B-luc expression injected in the artery of superficialized rat kidneys, local luciferase expression was demonstrated for the first time in vivo, by using bioluminescence imaging (Eker et al. 2010). The optimal heating protocol was found to be 43° during 5 min to get reproducible significant expression without parenchymal deleterious effect of heating.
4 Imaging of Cell Receptors
Molecular MR imaging of cell receptors is a growing field and requires the development of specific contrast agents. The most developed strategy for targeting cells using MR imaging is to bind a contrast agent with a monoclonal antibody, an aptamer, or a peptide, able to recognize specifically a surface antigen expressed on the cell membrane surface. Up until now, the main applications focused on targeting inflammatory receptors, such as P-selectin (Chaubet et al. 2007), E-selectin (Reynolds et al. 2006), VCAM (Nahrendorf et al. 2006), ICAM (Choi et al. 2007), or tumor-specific receptors, such as HER2/neu (Daldrup-Link et al. 2005). To our knowledge, no application of these strategies involved the kidney.
Apoptosis and its regulatory mechanisms contribute to cell number regulation in ARF (Ortiz et al. 2003). Tubular cell apoptosis is promoted by both exogenous factors such as nephrotoxic drugs and bacterial products and endogenous factors such as lethal cytokines. Conversely to necrosis, which is a nonreversible process, apoptotic pathways are potentially accessible to therapeutic modulation (Rana et al. 2001). MR imaging for the detection of apoptosis requires labeling of apoptotic cells using USPIOs as a marker and phosphatidylserine as a target. This necessitates linking the superparamagnetic nanoparticle to annexin V or synaptotagmin I both of which bind to the phosphatidylserine present on the outer leaflet of the plasma membrane of apoptotic cells (Hakumaki and Brindle 2003). Several preclinical applications using MR imaging have been reported to target apoptotic cells within the heart (Hiller et al. 2006) or within tumors during chemotherapy (Zhao et al. 2001), but, to our knowledge, this approach has never been applied to any kidney model.
5 MR Imaging of Enzyme Synthesis or Activity
Whereas enzyme activity is highly involved in renal physiopathology of many renal parenchymal diseases and urinary cancers, nothing has been done in that field using MR imaging.
5.1 MR Imaging of Matrix Metalloproteases (MMPs)
Matrix metalloproteases (MMPs) are zinc-containing endopeptidases that are involved in the degradation and remodeling of the ECM, crucial for tissue development and homeostasis (Catania et al. 2007). They localize to the cell surface or extracellular compartments. Their spatial expression in the kidney is complex and has not been completely characterized. MMP activity is associated with tumor invasion and metastasis, justifying the development of MMP inhibitors as anticancer alternatives. Within the kidney, an aberrant MMP expression is related to a number of renal pathologies, both acute and chronic. In acute renal diseases, there is strong evidence that MMPs mediate acute kidney injury and are involved in changes in the vascular endothelium, glomeruli, and tubular epithelial cells. In ischemia-reperfusion model, increased MMP-9 activity was associated with an increased vascular and tubular permeability, due to the degradation of cell adhesion molecules (Caron et al. 2005). In chronic kidney diseases (CKD), a role for MMPs has been demonstrated in several experimental models. For example, decreased MMP-9 expression was correlated with the development of tubulointerstitial fibrosis and glomerulosclerosis in rats (Bolbrinker et al. 2006). A number of studies have also demonstrated a link between aberrant MMP expression and the progression of diabetic nephropathy in animal models (McLennan et al. 2002). Overexpression of a single MMP may be a critical mediator of CKD. However, the relationship between MMP activity and inflammation remains an important component of CKD that remains to be addressed.
A Gd-chelate coupled with a MMP inhibitor was recently developed for MR imaging of atherosclerotic plaques (Lancelot et al. 2008). The affinity of this agent in vitro was broad toward MMP-1, 2, 3, 8, 9, and 13. In vivo, its affinity and specificity allowed accurate discrimination of MMP-poor and MMP-rich plaques. Another type of MR contrast agent, specific for MMPs, was proposed by Lepage et al. (2007), based on the concept of solubility switch, from hydrophilic to hydrophobic, modifying its pharmacokinetic properties. This agent, associating Gd-DOTA and a proteinase-sensitive peptide, was designed to display a reduced solubility after cleavage by MMP-7, responsible for an increased retention, making the detection of its activity possible. Applications of such agents in the nephro-urological sphere, as acute and chronic renal diseases and urological cancers, are really attractive but must still be developed.
5.2 MR Imaging of Myeloperoxidase (MPO)
Myeloperoxidase (MPO) is one of the most abundant enzymes secreted by inflammatory mononuclear cells (neutrophils and macrophages), able to generate reactive oxygen species as hypochlorous acid/hypochlorite (HOCl/OCl–) from hydrogen peroxide in the presence of chloride ions. Within the kidney, this reaction leads to a variety of chlorinated protein and lipid adducts that in turn may cause dysfunction of cells in different compartments of renal parenchyma (Malle et al. 2003). MPO is an important pathogenic factor in glomerular and tubulointerstitial diseases: for example, MPO and HOCl-modified proteins can be seen in glomerular peripheral basement membranes and podocytes in human membranous glomerulonephritis; MPO antibody complexes induce and exacerbate the inflammation in necrotizing glomerulonephritis. Antineutrophil cytoplasmic antibodies with anti-MPO specificity have a key role in the pathogenesis of microscopic polyangiitis, which may damage the kidneys and other organs.
A MPO-sensitive Gd-based MR contrast agent has been developed recently (Chen et al. 2006). MPOs are able to activate this agent by inducing oligomerization and protein binding and, consequently, by increasing its relaxivity. Experiments have shown that this MPO activity could be targeted in vivo within reperfused ischemic myocardium (Nahrendorf et al. 2008) and within inflammatory demyelinating plaques in the brain (Chen et al. 2006, 2008). Applications of this technique within the kidney should be numerous in the future.
6 New Parameters of Renal Function
6.1 Imaging of Tubule Dysfunction
In ARF models, the primer site of renal injury (proximal or distal) remains uncertain (Dagher et al. 2003). Using Gd-dendrimer chelates, which are cleared by glomerular filtration, some authors could demonstrate the proximal tubule dysfunction in acute tubular damage induced by cisplatin in mice (Kobayashi et al. 2002). This contrast agent accumulates within the outer medulla, where the proximal straight tubules are located (Fig. 9a). In diseased kidneys, the bright SI of the outer medulla does not show, and the gradation of tubular damage assessed by dynamic MR imaging correlates with renal function (Fig. 9b, c).
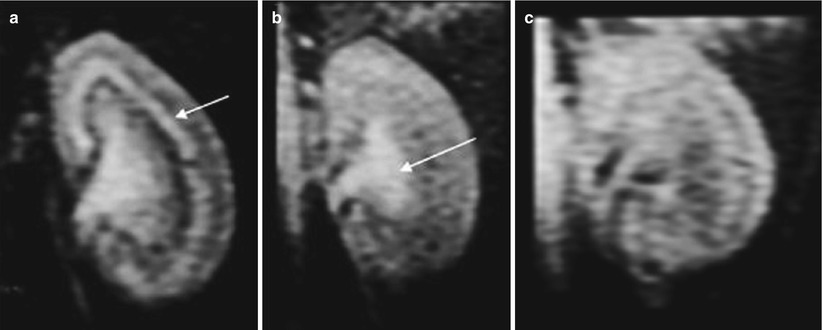
Get Clinical Tree app for offline access
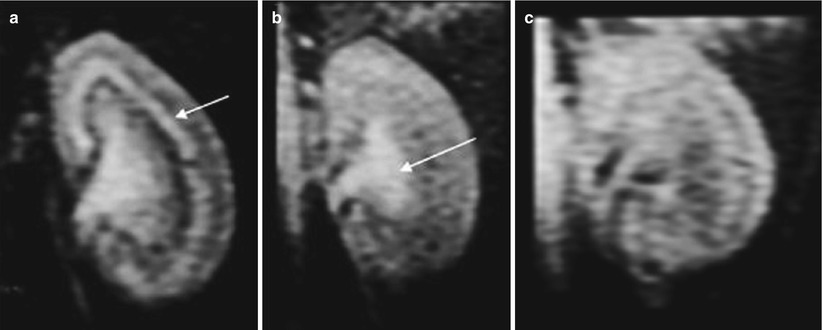
Fig. 9
Renal enhancement after the injection of dendrimer-based contrast agent: in a normally functioning kidney (a), we note a bright band within the outer stripe of the outer medulla (arrow) where the proximal straight tubules are located. In mildly damaged kidney (b), this bright band disappears and the late enhancement of the inner medulla predominates (arrow
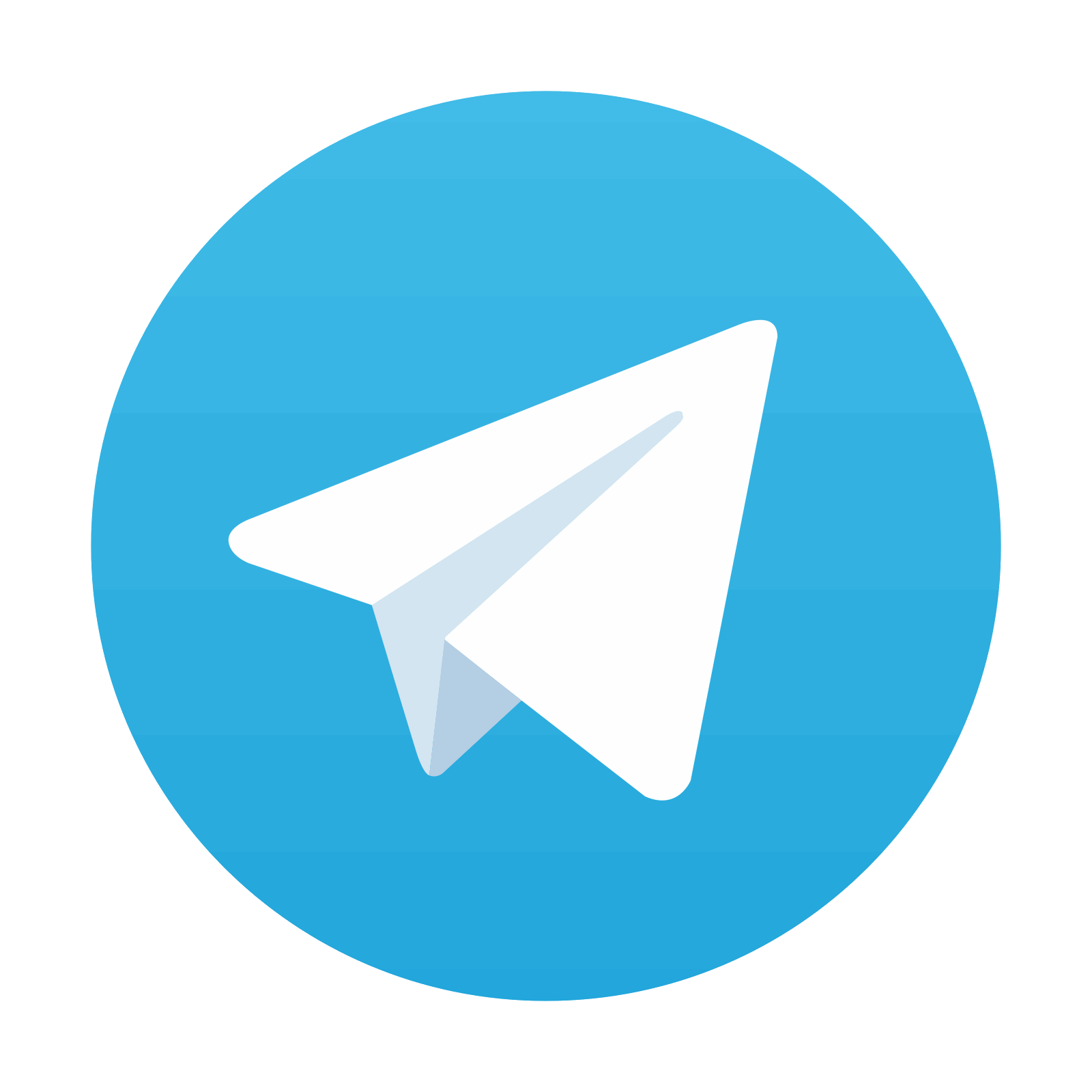
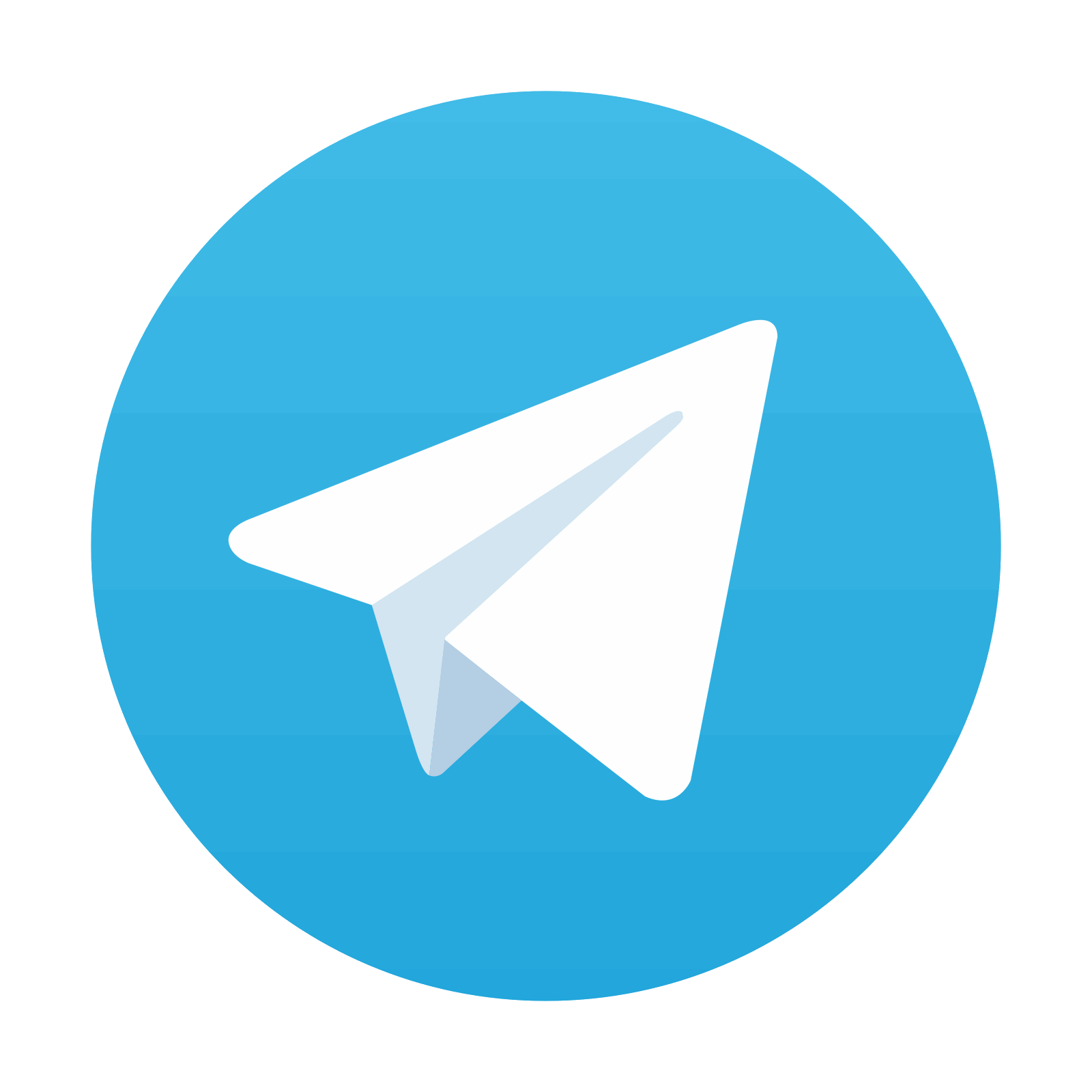
Stay updated, free articles. Join our Telegram channel

Full access? Get Clinical Tree
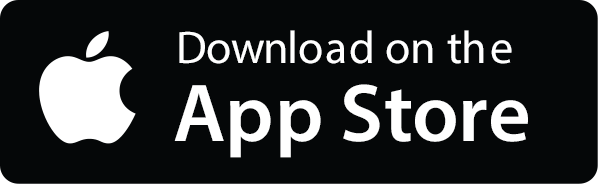
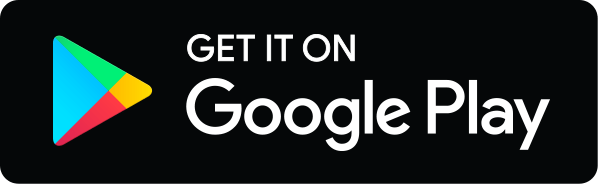
