PET and Drug Development
Jerry M. Collins
Development of a drug is a process that depends heavily on knowledge of its distribution in the body and its effects on the body. As phrased in Table 13.1, researchers want to know where the drug goes, what it does there, and if there are apparent relationships between drug localization and drug effects.
With positron emission tomography (PET) imaging it is possible to obtain direct information about both the distribution and the functional effects of a drug. PET can provide unique, value-added contributions to the drug development process. Perhaps even more important than its exquisite sensitivity, PET provides spatial information on drug distribution and drug effects that is virtually never attained in living humans by other means.
There are many similarities between the development of therapeutic agents and probes for PET imaging of in vivo functions (the term probe is used in this chapter to designate the positron-emitting compound used to image biochemical, molecular, and/or physiologic pathways). Both treatment and imaging approaches attempt to exploit differences between some process within the target and in other tissues. Another very practical characteristic for both therapeutics and probes is that the development process can be viewed as a pipeline or process that stretches over several years. The time at which a novel therapeutic agent enters clinical testing is far too late to decide that it would be useful to pursue imaging of its impact. Once a target for screening of new therapeutics has been identified, it also becomes a target to be considered for imaging. Even if developed from the same screening approach, the ideal probe molecule may or may not be the same as the optimal therapeutic molecule.
One of the major challenges is development of new probes. Fluorine-18 ([18F])-fluorodeoxyglucose (FDG) is the only functional imaging probe readily available at all PET facilities. Pilot trials have been reported for various other potential probes, but a concerted effort is required to prioritize targets and probe development projects. The recent announcement by the U.S. Food and Drug Administration on exploratory investigational new drugs has been especially useful for the initial human experiences with novel PET imaging agents (1).
Although drug development and probe development can be interrelated, they can also be separate processes. At one level, the role of a probe is to support the development of the therapeutic. In some cases, the probe might assist in the choice of doses of the therapeutic for the pivotal trials, leading to marketing approval. In such cases, the approval of the therapeutic is based on the success of the trials, not the imaging studies. The probe itself may never be used again. It is thus to be expected that some highly specialized probes may be used in drug development that does not have an ultimate clinically practical applicability.
In other cases, a general functional probe may be valuable for an entire class of drugs, or even many classes of drugs. The probe itself becomes a diagnostic tool that might be marketed in its own right. Fluorine-18-FDG is the most advanced example of this pattern for PET probes.
As described in the next two sections, useful probes for PET imaging can be obtained by positron labeling of either the therapeutic substance itself or the indicators (ligands, substrates, tracers) of functional status. For drug distribution studies, the most relevant information is obtained when the drug itself can be labeled with a positron-emitting atom.
Functional imaging evaluates processes occurring at the cellular or tissue/organ level: physiologic (e.g., transport carrier system), molecular (e.g., receptor binding), or biochemical (e.g., enzymatic activity). Thus, for functional imaging, the most important factor is a clear concept of the process to be probed. Focus on specific targets at these levels is part of a general shift in the paradigm of clinical diagnosis and treatment.
In addition to the value of imaging for drug development, the same tools could be used for customizing patient-specific treatment. Physicians have long sought tests that could assist in the choice of the most appropriate therapy for the individual patient. Traditional selection of therapy for individuals has relied on prognostic factors derived from large populations. For example, in anticancer therapy, the histology of the tumor is a key factor. Once therapy has been selected, it is generally continued until there is an obvious failure to control disease (e.g., tumor growth) or to control symptoms (e.g., pain).
By shifting emphasis toward underlying targets, functional imaging with PET has the potential to improve the selection and subsequent evaluations of therapy. PET provides the opportunity to
measure several characteristics of a disease target serially and noninvasively.
measure several characteristics of a disease target serially and noninvasively.
Table 13.1 Question-based Drug Development | |
---|---|
|
For some imaging targets (e.g., enzymes, receptors, and transporters), patients can be phenotyped before initiation of treatment. The goal is to obtain information that can be used to optimize the match between drugs and the patient-specific characteristics of the target. In addition to the potential for improving efficacy, these procedures would be valuable for avoiding needless toxicity from treatment regimens that are inappropriate for the individual patient being assessed.
Regardless of whether pretreatment phenotyping is feasible, all potential targets should be able to provide therapeutic assessment once treatment is under way. Rather than waiting until overt failure is demonstrated, which could be many months for conditions that are difficult to assess, the goal is to determine as quickly as possible whether the particular therapy is working for a specific patient. The optimal imaging time will depend on the mechanism of action for the therapy, but the ideal situation would be immediately after the first dose so a decision can be made regarding further treatment. Positive findings would be greatly appreciated by all. Negative results would provide the opportunity to explore other therapeutic approaches before further deterioration of the patient’s condition and ability to tolerate therapy.
The examples provided in the next two sections elaborate on these concepts. It is important to keep in mind that all of these examples are works in progress. There are currently very few PET imaging probes that are “accepted” for routine use by the nuclear medicine community except [18F]-FDG.
Assessment of Drug Delivery with PET
The ultimate goal of drug delivery (pharmacokinetics) is to get the active molecule to the target site. In classic drug development, systemic exposure to the drug is assessed by serial measurements of the drug concentration in plasma. Monitoring plasma concentrations to determine the systemic exposure component of drug delivery is certainly helpful, but there remain fundamental questions that can only be addressed by examining the second component of drug delivery, namely, the interaction between systemic exposure and local transport processes. In the preclinical phases of drug development, drug distribution studies are conducted in animal species to gain confidence that the active molecules reach the target sites of interest. In contrast, once human testing begins, researchers no longer have access to invasive sampling of many tissue sites. Without the noninvasive tools of imaging, the second component of local drug delivery is invisible, except for the potential effects of the drug on the target, and researchers can only evaluate the systemic component.
Anti-infective Drugs: Penetration to Target
The success of an anti-infective drug depends on its ability to reach bacteria, viruses, fungi, or other parasites wherever they may reside in the body. There is particular concern about delivering adequate concentrations of drugs to so-called sanctuary sites such as the brain or prostatic fluid. Comprehensive testing is conducted to determine the intrinsic sensitivity of various invading organisms, with the results often focusing on concentrations required to achieve 90% kill or 90% inhibition of growth—that is, the IC90. No matter how impressively potent a drug might be in attacking the target in laboratory tests, it cannot be successful in patients unless it is delivered to the target site.
Fig. 13.1 provides an excellent demonstration of the role PET imaging can play in determining if a particular drug is likely to reach the critical tissue of interest. Fischman et al. (2) labeled the antifungal drug, fluconazole, with [18F] and administered it to human volunteers in a phase I study. As illustrated, the time course of distribution for [18F]-fluconazole was followed for 2 hours in eight body areas. Because humans do not metabolize fluconazole, only the parent drug is present. Thus, the absolute quantitation of radiolabel provided by PET imaging can be translated into traditional units of drug concentration (in micrograms per milliliter) that can then be compared against the therapeutic goals defined by IC90 testing. In this case, with a single study early in drug development,
a positive finding can help sustain interest in the lead candidate. Alternatively, the outcome of such a study could definitively show serious obstacles to further development. Although such a result would be disappointing, it is surely better to find out early in development, before clinical efficacy studies in patients are started.
a positive finding can help sustain interest in the lead candidate. Alternatively, the outcome of such a study could definitively show serious obstacles to further development. Although such a result would be disappointing, it is surely better to find out early in development, before clinical efficacy studies in patients are started.
Transporters and Their Modulation
As mentioned at the beginning of this section, local transport processes can control access of a drug to its target. The understanding of these transporters or pumps has blossomed dramatically in the past few years. Several families of pumps have been discovered and classified phenotypically. In addition, the genes have been cloned, and transporters are now recognized as one of the largest categories of expression products of the mammalian genomes.
Because of the key role of these transporters in regulating drug delivery to target sites, development of probes to assess their operation is a major opportunity for PET imaging. Developmental programs for probes of drug uptake into the cell and efflux from the cell are in early stages of development but build on a tradition of monitoring PET probes of the transporters for amino acid entry into the cell (3).
There is movement away from the study of the distribution of a particular drug and toward a general functional probe for a transporter. Some groups have sought a general probe because there are too many drugs to study each of them. With the recent explosion in the catalog of transporters, one might also say that there are too many transporters to have a probe for each of them. The choice of approach not only is philosophical but also should be driven by the specific question under study.
Drug Delivery to Tumors
Although transport processes can influence all categories of drugs, their role is critical for drug delivery to tumors, due to the narrow therapeutic index of anticancer drugs. Efflux pumps can mediate resistance to anticancer drugs. As the drug approaches its target, the tumor may pump it out. Thus, concentrations at the target are very low, making them ineffective and actually promoting the development of further resistance mechanisms. There are a number of efforts under way to develop modulators that block these transport systems.
PET imaging is a tool that can focus directly on drug delivery at the target of interest. Delivery of a drug to the tumor does not guarantee successful therapy. However, if the drug never gets to the target or gets pushed away as soon as it arrives, it certainly will not be effective. The earlier researchers discover the problem, the sooner they can implement strategies for attempting to modulate this pharmacokinetic issue and the sooner they can consider alternative therapy. Many of these concepts are illustrated in Fig. 13.2. Consider the case in which a drug (e.g., paclitaxel) has been selected for treating a patient with a tumor, based on the tumor’s histology and other prognostic factors. If paclitaxel is labeled with a positron emitter, it becomes a suitable probe to seek an answer to the question at the top of Fig. 13.2: does the drug accumulate in the tumor? If the answer is no (the drug fails to accumulate in the tumor), one option is to conclude that paclitaxel can only cause toxicity for this patient and another therapeutic approach should be taken. Substantial validation would be required to confirm the logical scheme, but the outline for decision making is clear.
If the answer is yes (the drug does accumulate in the tumor), there is no guarantee that the tumor will respond to paclitaxel, but the probability of success has been enhanced because at least some nonresponders have been eliminated from the population. Accumulation of drug in the tumor is essential but is not the sole determinant of chemosensitivity. In other words, accumulation is necessary, but not sufficient for response. If the tumor fails to respond despite adequate drug accumulation, one of the most common mechanisms of resistance has been ruled out, and other reasons must be considered.
Patients with tumors that fail to accumulate paclitaxel can become candidates for therapy with drugs that are intended to modulate paclitaxel delivery to tumors—that is, to “fix” resistance due to transport. If the labeled paclitaxel still fails to accumulate in the tumor, then the modulator has failed, at least for the dose and schedule chosen. Once again, it may be desirable to spare the patient the toxicity of paclitaxel and to seek other therapeutic approaches.
If the use of the modulator does permit labeled paclitaxel to accumulate in the tumor, transport resistance has been fixed. Once again, there is no guarantee that transport resistance is the sole mechanism of resistance, but at least a population has been identified with an enhanced probability that the modulator will permit benefit from paclitaxel to be attained. Critical to the success of a radiolabeled drug as a targeting probe is that it is not extensively metabolized. PET only traces the radiolabel but does not indicate to what the radiolabel is attached. For extensively metabolized drugs, the PET imaging approach will be complicated by the distribution in vivo of radiolabeled metabolites.
Cellular and Molecular Targets for PET Imaging
Some of the major categories of targets for PET probes are shown in Table 13.2. The first two categories—energy metabolism and DNA synthesis—are very general downstream approaches to the determination of tumor functional status. Although the contemporary emphasis is on precise molecular classification of targets, it is still quite desirable to have these “universal” probes as a check on the relevance of hypotheses, as well as for the situations in which an appropriate target-specific or drug-specific probe is simply not available.
As described earlier, FDG is the leading functional PET probe and has remarkable versatility. Evaluation of the rate of DNA
synthesis and cellular proliferation is another approach toward a general probe. In the laboratory, tritiated thymidine (dThd) has been used for decades to evaluate DNA synthesis in cell culture. In anticancer therapy, the ability to monitor the impact of conventional cytotoxic regimens on DNA synthesis is easily appreciated. In addition, therapeutic approaches of many contemporary targets are ultimately intended to reduce proliferation and may be evaluated using dThd analogues (dThd analogues are discussed in more detail later in this section).
synthesis and cellular proliferation is another approach toward a general probe. In the laboratory, tritiated thymidine (dThd) has been used for decades to evaluate DNA synthesis in cell culture. In anticancer therapy, the ability to monitor the impact of conventional cytotoxic regimens on DNA synthesis is easily appreciated. In addition, therapeutic approaches of many contemporary targets are ultimately intended to reduce proliferation and may be evaluated using dThd analogues (dThd analogues are discussed in more detail later in this section).
Table 13.2 Categories of PET Functional Imaging Targets | |
---|---|
|
A much more target-specific approach is represented by the last two categories of probes. Receptors and enzymes are well established as drug targets across the full spectrum of disease areas, and they are the cornerstones of modern therapeutic developmental programs in the areas of cell signaling and regulation. Thus, according to the paradigm for probe development, receptors and enzymes are also potential targets for functional imaging. Although the imaging of these targets remains in the pilot stage for most diseases, the feasibility for monitoring in situ receptor occupancy and enzyme activity has been solidly established in neuropharmacology, as described below.
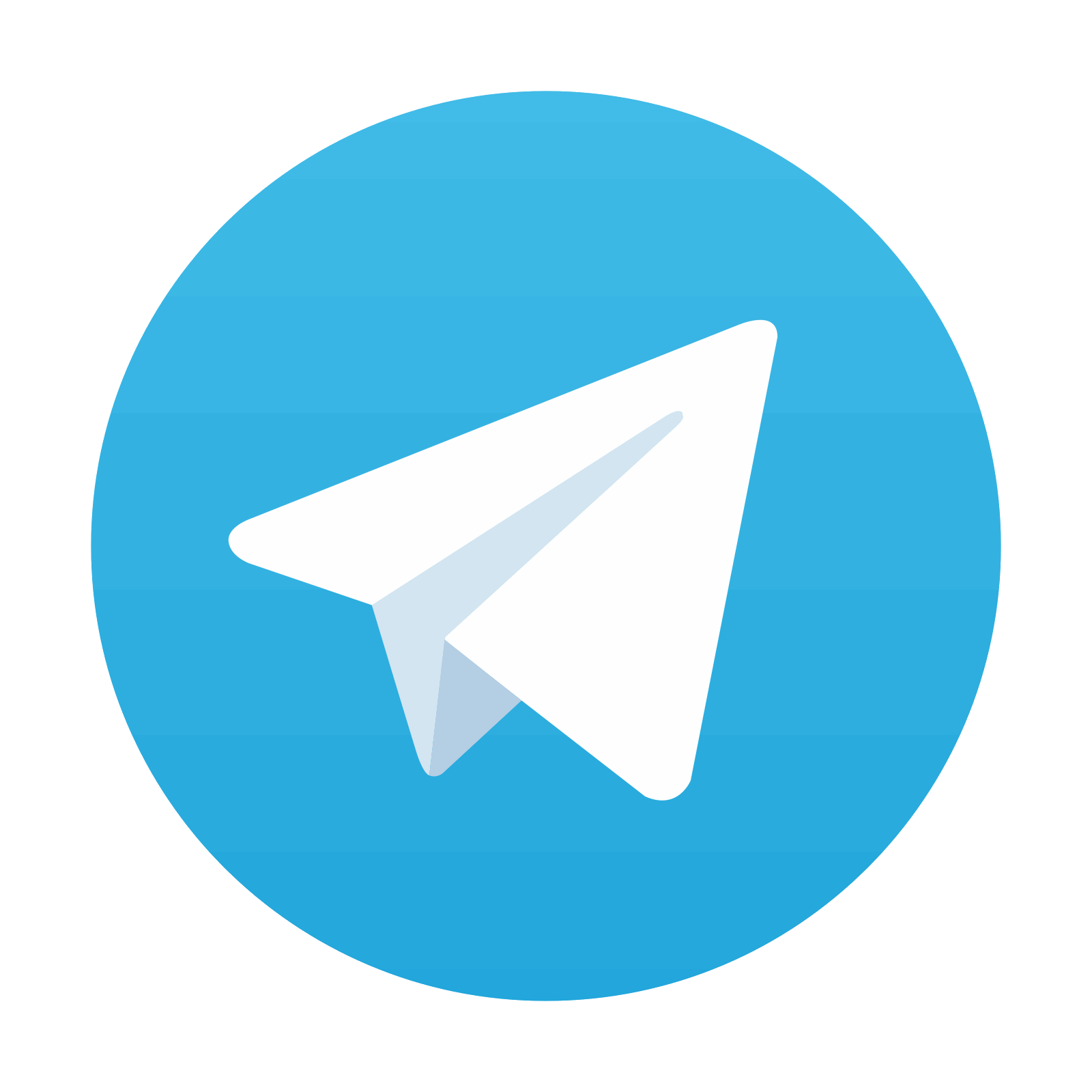
Stay updated, free articles. Join our Telegram channel

Full access? Get Clinical Tree
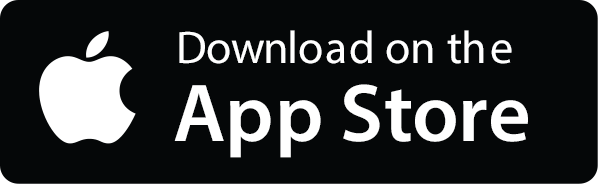
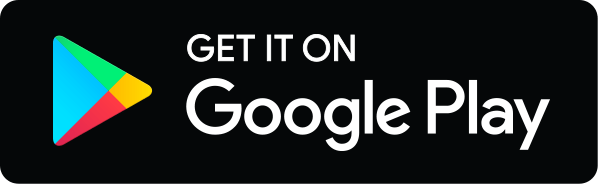