Fig. 21.1
(a) Areas of reduced regional cerebral blood flow (rCBF) (as assessed with [99mTc]-HMPAO-SPECT) in patients with heart failure (HF) relative to healthy controls are highlighted in yellow, overlaid on transaxial slices of a reference magnetic resonance imaging scan that approximates the Talairach and Tournoux Stereotactic Atlas (1988) (for details, see Alves et al. 2005). Numbers have been placed to indicate the foci that retained statistical significance at a p < 0.05 threshold, corrected for multiple comparisons, involving, respectively, the right lateral temporoparietal and posterior cingulate cortices (number 1) and the right and left precuneus and cuneus (number 2). The numbers at the bottom of each frame represent standard coordinates in the y-axis. (b) “See-through” statistical parametric maps showing all the foci of reduced rCBF in HF patients relative to controls that surpassed the p < 0.01 statistical threshold, containing 50 or more contiguous voxels (A anterior, I inferior, L left, P posterior, R right, S superior)
Recently, there have also been large studies in this field using [18F]FDG-PET (see Table 21.1 for details). Reiman et al. (2010) searched for significant associations between serum cholesterol levels and cerebral metabolic rates of glucose metabolism (CMRgl) in 117 cognitively normal middle-aged and elderly individuals (47–68 years of age). Higher serum total cholesterol levels were associated with lower CMRgl bilaterally in the precuneus, lateral parietal neocortex (encompassing the superior parietal lobule, angular, and supramarginal gyri), lateral temporal neocortex (involving the superior temporal gyrus), and lateral prefrontal cortex (including the superior frontal gyrus), in a pattern that shows a substantial degree of overlap with the profile of regional brain functional changes commonly seen in subjects with mild AD (Reiman et al. 2010).
Table 21.1
Findings of PET studies indicating reduced glucose metabolism in proportion to the degree of cardiovascular risk
Cardiovascular risk factors | Study design and sample | Imaging methodology (dose of injected radioisotope) | Equipment | Correction for partial volume effects | Findings (resting state) | Reference |
---|---|---|---|---|---|---|
Obesity | Cross-sectional | [18F]FDG (4–6 mCi) | Siemens-CTI ECAT HR+ scanner | No | Negative correlation between BMI and metabolic activity in prefrontal/cingulate cortices | Volkow et al. (2009) |
Adult subjects (n = 21; BMI range 19–37 kg/m2) | ||||||
Age: 34 ± 7 years | ||||||
Levels of glycemia: a | ||||||
Combined cardiovascular risk factors (FCHDR) | Cross-sectional | [18F]FDG (10 mCi) | Siemens-CTI ECAT EXACT (921) scanner | Yes | Higher FCRP associated with lower metabolism in prefrontal cortex | Kuczynski et al. (2009) |
Elderly subjects (n = 58) ranging from normal cognition to dementia | ||||||
Age: 75 ± 7 years | ||||||
Levels of glycemia: a | ||||||
Higher serum total cholesterol levels | Cross-sectional | [18F]FDG (5–8 mCi) | Siemens-CTI ECAT HR + scanner | No | Higher serum cholesterol levels associated with lower metabolism in precuneus, lateral temporoparietal neocortex, and prefrontal córtex | Reiman et al. (2010) |
Cognitively normal midlife to old adult subjects (n = 117) | ||||||
Age: 47–68 years | ||||||
Levels of glycemia: a | ||||||
Insulin resistance | Cross-sectional | [18F]FDG (5 mCi) | Not mentioned in the study | No | Greater insulin resistance associated with reduced metabolism in posterior cingulate gyrus, precuneus, and lateral temporoparietal neocortex | Baker et al. (2011) |
Elderly subjects (n = 29, including individuals with prediabetes, diabetes, and normal fasting glucose rates) | ||||||
Age: 74 ± 7 years | ||||||
Levels of glycemia: 100 ± 6 mg/dL (normal) and 107 ± 12 mg/dL (prediabetes and diabetes) | ||||||
Elevated blood pressure | Cross-sectional | [18F]FDG (5–8 mCi) | Siemens-CTI ECAT HR+ scanner | No | Higher blood pressure associated with lower metabolism in prefrontal cortex | Langbaum et al. (2012) |
Cognitively normal midlife to old adult subjects (n = 26) | ||||||
Age: 47–68 years | ||||||
Levels of glycemia: a | ||||||
Combined cardiovascular risk factors (FCHDR) | Cross-sectional | [18F]FDG (10 mCi) | Siemens Biograph-16 PET-CT scanner | Yes | Metabolic reductions in the high-risk group relative to the low-risk group in the precuneus, posterior cingulate gyrus, and temporoparietal cortex | Tamashiro-Duran et al. (2013) |
Cognitively normal elderlies (n = 59) divided into three groups according to their cardiovascular risk | ||||||
Age: 66–75 years | ||||||
Levels of glycemia: 95 ± 10 mg/dL (low risk), 101 ± 9 mg/dL (medium risk), and 104 ± 12 mg/dL (high risk) |
Investigating a subgroup of the same cohort, Langbaum et al. (2012) detected lower frontotemporal glucose metabolism in proportion to elevated blood pressure indices (Langbaum et al. 2012). In additional studies with relatively modest samples, findings of regional cerebral hypometabolism as assessed with [18F]FDG-PET have been described in association with other CVRF, including insulin resistance (Baker et al. 2011) and obesity (Volkow et al. 2009). Taken together, these studies have described significant associations between those risk factors and reduced CMRgl, variably implicating the precuneus, posterior cingulate gyrus, lateral parietal neocortex, and lateral temporal neocortex (Baker et al. 2011; Langbaum et al. 2012), as well as the lateral prefrontal cortex (Volkow et al. 2009; Langbaum et al. 2012).
In conclusion, the above [18F]FDG-PET and rCBF SPECT studies assessing non-demented individuals with high levels of CVRF have provided support to the “vascular hypothesis” of AD, demonstrating the presence of localized brain functional changes in such subjects in the same brain regions known to be primarily affected in AD.
21.3.1 The Impact of Combined Cardiovascular Risk Factors on Brain Functioning
CVRF rarely occur in isolation in elderly populations (Meigs et al. 1997; Razay et al. 2007), and therefore the approach of investigating the impact on the brain of single risk factors may be limited. The Framingham Coronary Heart Disease Risk (FCHDR) index is a composite measure that takes into account multiple risk factors (such as age, gender, blood pressure, smoking status, total cholesterol and high-density lipoprotein cholesterol levels, as well as the presence of diabetes) to assess the 10-year risk that an individual has for coronary heart disease (Wilson et al. 1998). This risk score has been widely used in epidemiological studies of elderly populations (Jeerakathil et al. 2004; Seshadri 2006).
Using FCHDR indices, our group has recently conducted a morphometric MRI study of a relatively large (n = 248) population-based sample of cognitively intact elderly individuals (age range 66–75 years) with no silent brain infarcts, in order to investigate the influence of combined CVRF on regional brain volumes (de Toledo Ferraz Alves et al. 2011). Using an automated voxel-based morphometry (VBM) approach with the SPM program, we detected regionally decreased gray matter volumes in direct proportion to the degree of cardiovascular risk, involving the lateral parietal and temporal neocortices (de Toledo Ferraz Alves et al. 2011).
A similar approach has now started to be used in [18F]FDG-PET studies (see Table 21.1). For instance, Kuczynski et al. (2009) obtained measures of regional glucose metabolism and cardiovascular risk as assessed with the FCHDR in a sample of elderly subjects (n = 58, age >55 years), focusing specifically on the frontal lobe. Their study sample included a proportion of demented individuals in addition to healthy elderlies. They observed a significant inverse association between FCHDR scores and CMRgl in different frontal lobe portions including the lateral (i.e., superior frontal gyrus and ventrolateral prefrontal cortex) and medial (i.e., superior medial frontal and superior orbital frontal gyri) prefrontal cortices (Kuczynski et al. 2009) (Table 21.1).
With the aim of extending the above findings to more cognitively preserved elderly subjects, our group has recently acquired [18F]FDG-PET data in a subset (n = 59) of the cognitively intact elderly population recruited for the VBM investigation cited above (de Toledo Ferraz Alves et al. 2011). Subdividing these individuals in three groups according to their degree of cardiovascular risk, we detected significantly reduced glucose metabolism in the precuneus, posterior cingulate gyrus, and lateral temporal and parietal neocortices when comparing the subgroup with high FCHDR scores to the subgroup with low scores (Tamashiro-Duran et al. 2013) (Fig. 21.2). Such pattern of [18F]FDG-PET results provide further evidence of the substantial degree of overlap in regard to the location of brain foci of hypofunction across imaging studies of AD and CVRF. It should be noted that, as we excluded individuals with a diagnosis of diabetes mellitus from this PET investigation, such pattern of functional brain deficits related to CVRF can only be claimed to be representative of high-risk elderly samples without a history of diabetes mellitus.
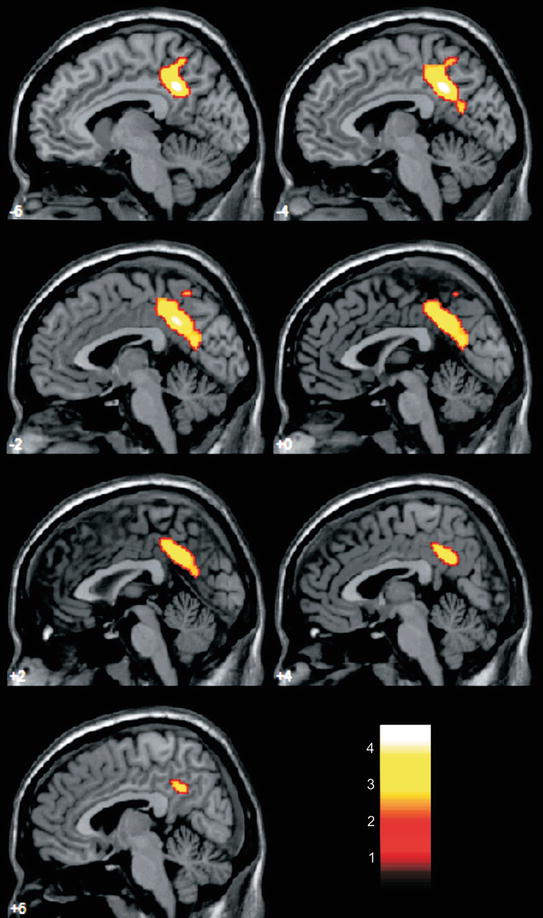
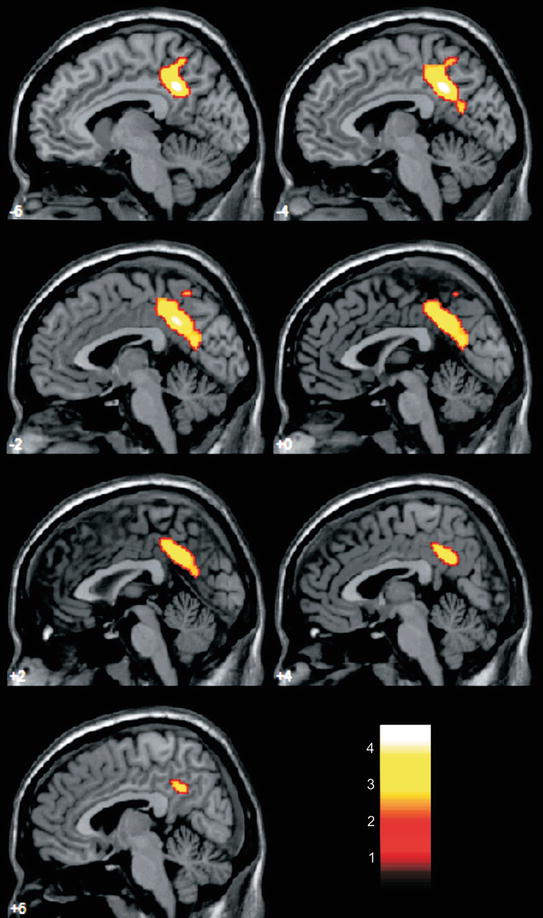
Fig. 21.2
Areas of reduced cerebral glucose metabolism (as assessed with [18F]FDG-PET) in a group of cognitively intact elderlies with high cardiovascular risk (according to Framingham Coronary Heart Disease Risk Profile scores) compared to an age-matched group with low cardiovascular risk are highlighted in yellow, overlaid on sagittal slices of a reference magnetic resonance imaging scan that approximates the Talairach and Tournoux Stereotactic Atlas (1988) (for details, see Tamashiro-Duran et al. 2013). Data were analyzed using voxel-based, statistical parametric mapping methods and findings reached significance at a p < 0.05 threshold, corrected for multiple comparisons. Between-group differences were most prominent in the left precuneus and posterior cingulate gyrus, in a pattern of location that resembles the findings of functional imaging studies of early stages of Alzheimer’s disease. Such findings cannot be simply accounted for group differences in the proportion of carriers of the APOE ε4 allele, as the analysis was controlled for the presence of this gene polymorphism. The color bar represents T-values. The numbers associated with each frame represent standard coordinates in the x-axis
It is interesting that our [18F]FDG-PET findings of reduced CMRgl in proportion to the degree of cardiovascular risk (Tamashiro-Duran et al. 2013) were more extensively distributed than those described in our VBM investigation of gray matter volume deficits in cognitively intact healthy subjects (de Toledo Ferraz Alves et al. 2011), despite the fact that the subsample investigated with [18F]FDG-PET was much more modest in size. This suggests that [18F]FDG-PET studies may unravel CVRF-related localized brain metabolic deficits in brain regions related to AD before any subtle brain atrophic changes can be detected in cognitively preserved individuals.
One other feature of our [18F]FDG-PET results that is reminiscent of findings reported in functioning imaging studies of incipient AD is the lack of hypometabolism in frontal regions (Tamashiro-Duran et al. 2013). This is in contrast both with the recently reported findings of cardiovascular risk-related prefrontal hypofunctioning in elderly subjects classified using the FCHDR index (Kuczynski et al. 2009) and the results of other [18F]FDG-PET imaging studies that investigated the influence of single CVRF on brain functioning (Volkow et al. 2009; Reiman et al. 2010; Baker et al. 2011; Langbaum et al. 2012) (Table 21.1). One important difference between our PET study and the one by Kuczynski et al. (2009) is the fact that the authors of the latter study did not exclude subjects with lacunar infarcts. They actually suggested that findings of frontal lobe hypometabolism in individuals with higher FCHDR scores could be determined by the greater incidence of lacunar infarcts in those subjects, leading to localized frontal metabolic changes (Kuczynski et al. 2009). This reasoning may explain the absence of frontal metabolic changes in our [18F]FDG-PET study, since we excluded subjects with vascular-related silent brain lesions as assessed by MRI, including lacunar infarcts. It is therefore plausible to argue that in the absence of lacunar infarcts, the frontal lobe is not especially vulnerable to the damaging effects of CVRF in cognitively intact individuals.
Very recently, Beason-Held and collaborators (2012) used FCHDR scores to investigate the relationship between baseline cardiovascular risk and subsequent changes in resting rCBF (as assessed with PET) in cognitively preserved elderlies (n = 97) who underwent repeated annual imaging assessments over a period of up to 8 years. The authors found a significant relationship between higher baseline FCHDR scores and greater progression of rCBF deficits in the precuneus, as well as in frontal regions, insula, and the brain stem. This is the first longitudinal PET study that provides evidence of progressive functional brain deficits in individuals with high cardiovascular risk load (Beason-Held et al. 2012).
21.3.2 Influence of APOE Gene Polymorphisms on the Relationship Between Cardiovascular Risk Factors and Functional Brain Deficits
One other aspect that may significantly affect the results of brain imaging studies in samples of elderly individuals is the influence of apolipoprotein E (APOE) gene polymorphisms, with its three allele variants (epsilon2/3/4). In imaging studies evaluating the impact of CVRF on brain functioning, it is of critical importance to investigate the influence of the APOE epsilon4 (APOE ε4) allele, given that the presence of this gene variant is an important risk factor for cardiovascular disease (Irie et al. 2008; Kivipelto et al. 2008). The APOE ε4 allele is also known to be a risk factor for pathological cognitive decline (Small et al. 2004). There have been PET studies documenting regional brain glucose metabolism variations as a function of the APOE genotype in non-demented individuals, with findings of glucose hypometabolism being associated with the presence of the APOE ε4 allele (Drzezga et al. 2005; Mosconi et al. 2008a). Recent studies have also suggested a “protective” role of the APOE ε2 allele on brain ageing and cognition, indicating a more complex role of APOE polymorphisms rather than a simple classification between APOE ε4 allele carriers and noncarriers (Berlau et al. 2009; Liu et al. 2010).
In the [18F]FDG-PET study by Reiman et al. (2010) reviewed earlier in this chapter, the sample of cognitively normal middle-aged and elderly individuals was subdivided in APOE ε4 allele homozygous (n = 24), heterozygous (n = 38), and noncarriers of this genetic variant (n = 55). The authors found that in some cortical regions, the relationship between hypometabolism and CVRF had greater salience in APOE ε4 allele carriers than in noncarriers. They postulated that higher cholesterol levels, particularly in association with the presence of the APOE ε4 allele, would increase the risk of AD by accelerating some of the brain changes associated with normal ageing (Reiman et al. 2010).
In our own [18F]FDG-PET study (Tamashiro-Duran et al. 2013), we wished to investigate whether the associations between reduced CMRgl and elevated FCHDR scores in brain regions implicated in the pathophysiology of AD would be present regardless of the presence of the APOE ε4 allele. After controlling for the presence of the APOE ε4 allele, our findings of CVRF-related regional brain hypofunctional patterns retained statistical significance in the precuneus and posterior cingulate gyrus (Fig. 21.2). This strongly suggests that findings of brain hypometabolism similar to those seen in AD subjects can be seen in association with the severity of CVRF in samples of cognitively preserved individuals who are at no increased genetic risk for AD (as far as the APOE genotype is concerned). Other findings of reduced [18F]FDG uptake in the high-risk group, involving the lateral temporoparietal neocortices, lost their significance when the analysis was repeated after controlling for the effects of the presence of the APOE ε4 allele (Tamashiro-Duran et al. 2013). This may indicate that findings of reduced glucose metabolism in those latter regions may be influenced by the presence of the APOE ε4 allele, as suggested by previous PET studies of non-elderly, cognitively preserved healthy subjects (Reiman et al. 2004, 2010; Langbaum et al. 2010).
Up until now, there have been no PET or SPECT investigations of CVRF in healthy individuals evaluating the possible protective role of the APOE ε2 allele.
21.3.3 Methodological Aspects in Functional Neuroimaging Studies of Cardiovascular Risk: The Impact of Partial Volume Effects
Although several rCBF SPECT and [18F]FDG-PET studies have investigated the influence of cardiac diseases and CVRF on brain functioning, the impact of partial volume effects (PVE) on the results of these studies has not been addressed regularly. Given the intrinsic limitations in spatial resolution of PET and SPECT methods, PVE may degrade the quantitative accuracy of the data acquired, causing an apparent metabolic reduction in areas with steep gray matter atrophy and artificial metabolic preservation in areas with less pronounced gray matter reductions (Meltzer et al. 2000; Aston et al. 2002; Yanase et al. 2005; Kochunov et al. 2009). We were recently able to directly document this in our sample of cognitively preserved elderlies evaluated with both [18F]FDG-PET (Tamashiro-Duran et al. 2013) and morphometric MRI (de Toledo Ferraz Alves et al. 2011), in an analysis investigating the relationship between chronological age and glucose metabolism deficits (Curiati et al. 2011). Thus, when our [18F]FDG-PET data were analyzed with no correction for PVE, we found significant linear correlations between age and glucose metabolism in two large clusters located, respectively, in the left orbitofrontal cortex and the right temporolimbic region; however, when the PET images were PVE corrected using the structural MRI data of the same subjects, there were no significant findings, indicating that the former relationship between metabolic deficits and age was largely secondary to the degree of regional brain atrophy in the same brain locations (Curiati et al. 2011).
From all of the functioning imaging studies of CVRF reviewed in this chapter, only the [18F]FDG-PET study by Kuczynski et al. (2009) and our own PET investigation (Tamashiro-Duran et al. 2013) included analyses corrected for PVE using MRI data from the same subjects (Table 21.1). In our study (Tamashiro-Duran et al. 2013), it was of critical relevance to include the step of PVE correction, as the VBM-based analysis of MRI data in our extend sample had revealed findings of regional gray matter atrophy in proportion to the degree of cardiovascular risk (de Toledo Ferraz Alves et al. 2011). However, most of our PET results of regional hypometabolism in proportion to CVRF retained their statistical significance after PVE correction. This assured us that the findings of CVRF-related brain hypofunctioning can be assumed to represent true metabolic deficits, found in excess of any brain tissue reduction in the same brain locations (Tamashiro-Duran et al. 2013).
21.4 Microstructural and Molecular Mechanisms Underlying Cardiovascular Risk-Related Brain Functioning Deficits
The continued influence of CVRF and cardiac disorders that lead to chronically reduced rCBF are thought to lead to numerous local pathological consequences (de la Torre 1999), including structural deformities of brain microvessels (Bell et al. 2009; Grammas 2011), microembolism, decreased oxygen and nutrient supply, metabolic deficits, toxic disturbances (Kumar-Singh 2008; Bangen et al. 2009; Menon and Kelley 2009), and endothelium dysfunction (Gimbrone 2010). Such deficits at the microstructural level are all likely to contribute to the findings of regional brain hypofunctioning detectable using [18F]FDG-PET and rCBF SPECT in clinical studies with humans.
It is also important to consider the possibility of direct links between microvascular changes secondary to chronic CBF reductions and the triggering of the characteristic neuropathological AD changes (de la Torre et al. 2003). The most reliably documented molecular abnormality underlying the typical AD-related neuropathological changes is the accumulation of amyloid β peptide (Aβ) (Hardy and Selkoe 2002). The Aβ peptide results from the proteolytic processing of the amyloid precursor protein by the β- and γ-secretase enzymes (Evin and Weidemann 2002) and insoluble Aβ deposits from the senile plaques characteristically found in the brains of patients with AD (Iwatsubo et al. 1994). The Aβ accumulation also leads to hyperphosphorylation of the tau protein, and this is the molecular basis of the microtubule changes that lead to the formation of neurofibrillary tangles (De Felice et al. 2008). Recently, molecular models have implicated circulatory defects (i.e., alterations in vascular smooth muscle cells of meningeal arterioles due to CVRF) as critical to impairments in the removal of Aβ from the brain across the blood-brain barrier (Bell et al. 2009). Chronic inflammatory processes, tightly linked to endothelial dysfunction, have also been increasingly implicated as relevant to the development of the Aβ-related molecular changes underlying the symptoms of AD (Grammas 2000; Zlokovic 2005, 2010; Salmina et al. 2010; Grammas et al. 2011).
Specific CVRF are associated with nonvascular microstructural and molecular changes which may also contribute to the presence of cognitive deficits and related functional imaging deficits as assessed with PET and SPECT. In the case of smoking, for instance, nicotine self-administration has been shown to decrease neurogenesis and neuroplasticity and increase cell death in the hippocampus (dentate gyrus) of rats and mice (Abrous et al. 2002; Oliveira-da-Silva et al. 2009). In the case of diabetes, there are specific inflammatory changes involving protein kinase C activation, excess production of reactive oxygen species, protein glycosylation, and cellular activation of the receptor for advanced glycation end products (Sims-Robinson et al. 2010; Taguchi 2009). Finally, recent research on glycogen synthase kinase-3 (GSK-3) provides one other interesting link between AD and diabetes. GSK-3 is a pivotal enzyme in glycogen synthesis and is thought to participate in the development of insulin resistance primarily by inhibiting glycogen synthase activity and, thus, decreasing the synthesis of glycogen (Lee and Kim 2007). GSK-3 has been implicated in the pathophysiology of AD because it promotes tau phosphorylation (Forlenza et al. 2000; Lei et al. 2011), and its activity is regulated by the Aβ peptide (Hernandez et al. 2009).
21.5 Conclusions and Future Directions
The functional imaging studies reviewed in this chapter provide strong indication that there are brain metabolic deficits detectable by PET and SPECT techniques in association with CVRF even in cognitively intact elderlies, involving brain regions previously shown to be particularly vulnerable to AD-related brain changes. Such findings provide support to the view that the combination of different CVRF may contribute critically to the development of AD-related neuropathological changes. Such literature findings also underscore the importance that should be given to the evaluation of vascular risk factors in any [18F]FDG-PET or rCBF SPECT studies of elderly populations, suggesting that interindividual differences in terms of cardiovascular risk load may account for the often high degree of intersubject variability of brain metabolic indices in elderly samples.
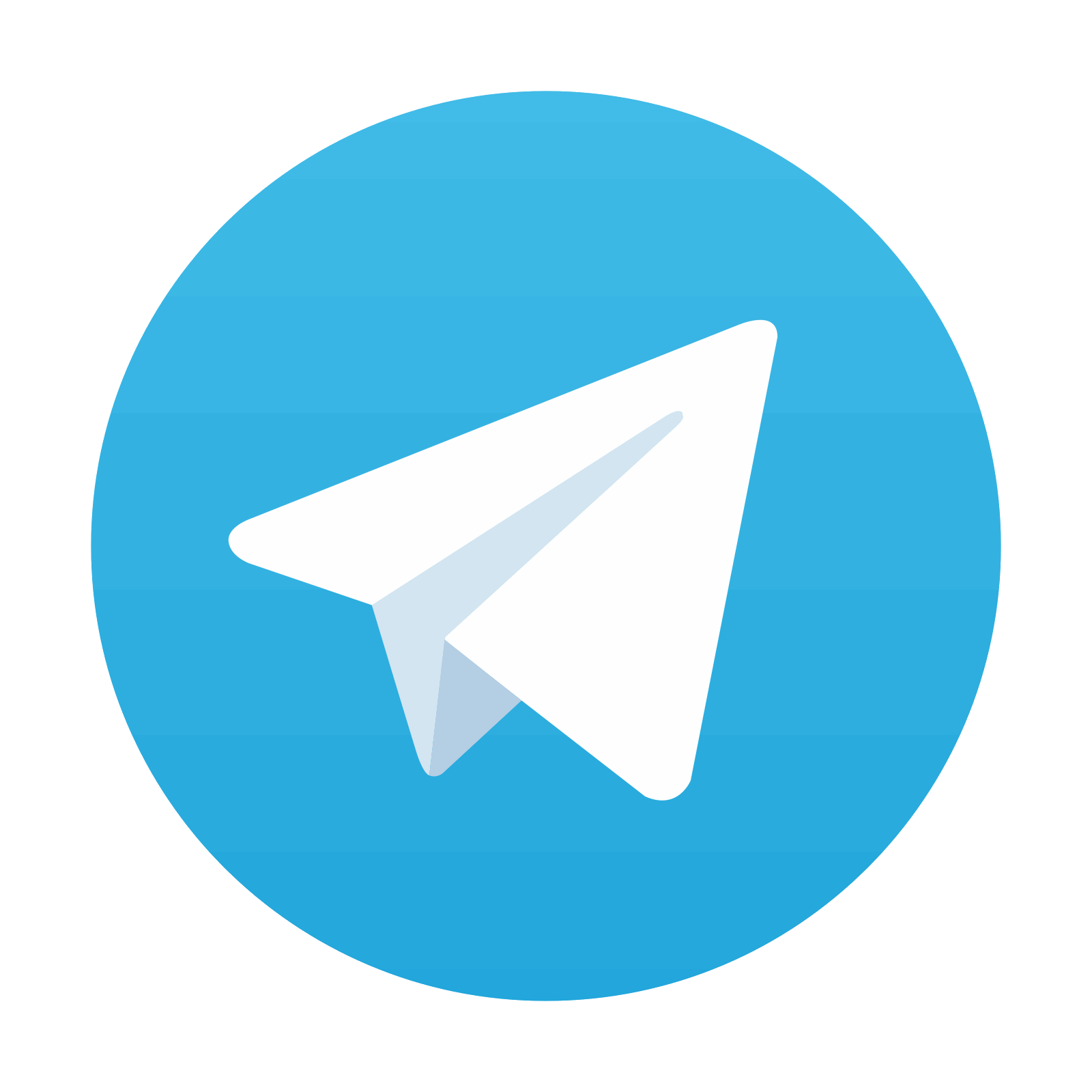
Stay updated, free articles. Join our Telegram channel

Full access? Get Clinical Tree
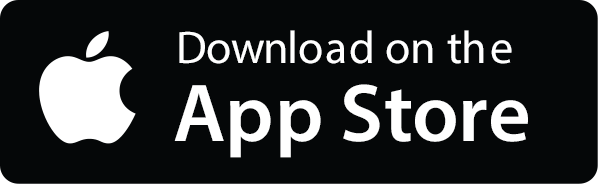
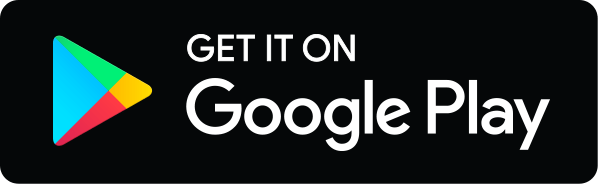