PET-CT and SPECT-CT in Pediatric Tumors and Infections
Martin Hutchings
Lise Borgwardt
Annika Loft
Liselotte Højgaard
The use of integrated positron emission tomography (PET)–computed tomography (CT) and integrated single-photon emission computed tomography (SPECT)–CT in pediatric oncology is based on a relatively limited number of systematic reports. Most of the evidence is derived from studies of adult populations. However, a number of studies show a high clinical value for PET and to a lesser extent for SPECT in the management of brain tumors in childhood (see Fig. 67.1). Fluorodeoxyglucose (FDG)-PET and FDG-PET-CT are useful for the staging and therapy monitoring of childhood lymphoma. These methods may be important tools for modern lymphoma management, which is tailored to the individual patient’s prognosis in order to avoid under- and overtreatment. In osteosarcoma and Ewing sarcoma, FDG-PET is more sensitive than bone scintigraphy and magnetic resonance imaging (MRI) in detecting bone and soft-tissue involvement. However, due to false-positive findings on PET, the specificity is higher for MRI. The false-positive rate is expected to decrease with PET-CT, but no data are yet available to confirm this. High FDG uptake in residual tumor and in metastatic lesions of rhabdomyosarcoma in pediatric patients has been documented, but the value of FDG-PET-CT has not yet been investigated. In the future, new more specific tracers may contribute to our understanding of childhood diseases, and the use of combined functional and anatomical imaging is likely to develop rapidly.
Iodine 123 (123I)-MIBG SPECT and SPECT-CT are recommended as the methods of choice for diagnosis and follow-up in childhood neuroblastoma. The role of PET and PET-CT with FDG and more specific tracers still remains to be established. Wilms tumor shows pathological FDG uptake. PET-CT might gain a role in the diagnosis of Wilms tumor in the future, especially for smaller tumors, which otherwise can be difficult to discriminate from FDG excretion in the normal kidney tissue.
Experience is limited on the use of SPECT-CT and PET-CT in inflammation and infection, but the technique seems very promising in a number of cases. The improved anatomical localization of foci that is offered by the image fusion methods is likely to increase the value of established inflammation imaging methods in pediatric nuclear medicine.
Introduction
Childhood cancer is rare, accounting for only 2% to 3% of all cancers, but cancer is second only to trauma as a cause of childhood death in the United States and Europe. In the Western world, the cancer incidence is 12 to 14 per 100,000 children under 15 years per year (1). During the last decades, the treatment of pediatric malignancies has been greatly improved, so the overall survival rate is more than 75% (2,3,4).
The neoplasms affecting children differ from those commonly found in adults, and several types of cancers are virtually unique to childhood. Neuroblastoma, Wilms tumor,
rhabdomyosarcoma, and retinoblastoma are predominantly or exclusively pediatric cancers (5). In addition, leukemias, lymphomas, central nervous system (CNS) tumors, and primary bone malignancies are of relatively greater importance in children than in adults. The most frequent malignancies in childhood are brain tumors, followed by neuroblastoma, osseous sarcoma, and lymphoma. Carcinomas of the lung, breast, stomach, large bowel, and prostate are extremely rare among children (6).
rhabdomyosarcoma, and retinoblastoma are predominantly or exclusively pediatric cancers (5). In addition, leukemias, lymphomas, central nervous system (CNS) tumors, and primary bone malignancies are of relatively greater importance in children than in adults. The most frequent malignancies in childhood are brain tumors, followed by neuroblastoma, osseous sarcoma, and lymphoma. Carcinomas of the lung, breast, stomach, large bowel, and prostate are extremely rare among children (6).
Experience with positron emission tomography (PET) and integrated PET–computed tomography (CT) has accumulated more slowly in pediatric than in adult cancers. The initial studies of PET applications in children have focused on disorders of the brain and congenital heart disorders. PET is rapidly becoming more widely available, and the clinical value of PET for childhood tumors is currently under investigation. PET has gained a dominant position within the nuclear medicine applications in pediatric oncology (7), but single-photon emission computed tomography (SPECT) and SPECT-CT play an important role in the management of certain pediatric tumors. Regarding both PET-CT and SPECT-CT, most of the available body of evidence still derives from studies of adult cancer patients. Very few data exist on the application of PET-CT and SPECT-CT in infectious and inflammatory diseases. However, a number of case reports indicate that the methods have a high value in these diseases.
Central Nervous System Tumors in Childhood
CNS tumors are the most common solid tumor in childhood (8), accounting for 20% to 25% of all cancers (see also Chapters 22 and 23). Although the prognosis has improved considerably over the last 2 decades, the overall cure rate today is approximately 60% (9), with the best prognosis for benign astrocytoma localized to the cerebellum (almost 100%) and the worst for brainstem gliomas (5% to 10%) (9). Brain tumors are the leading cause of cancer mortality in children (8). Descriptive classification by histological examination is crucial for the appropriate management of CNS tumors. Theoretically, all types might develop in children. However, the tumor types of special importance in childhood are significantly fewer than in adults and are dominated by medulloblastoma, pilocytic astrocytoma, diffuse astrocytoma, ependymoma, and craniopharyngioma (8).
Tumors localized centrally can rarely be totally resected without severe neurological deficits, and even biopsies can be a major risk. Thus, the burden of late effects is troublesome (9,10). Survivors of childhood CNS tumors often have severe neurological, neurocognitive, and psychosocial sequelae (10,11,12,13,14), either due to the tumor or the treatment necessary to control it.
Diagnostic imaging with CT and MRI (with MRI as the first priority) is generally used to monitor the effect of treatment on tumor and recurrence, but the image interpretation is impaired by changes in the brain tissue related to surgery, glucocorticosteroids, radiotherapy, and chemotherapy leading to non-tumor-associated post-treatment contrast enhancement. Thus, other noninvasive diagnostic modalities, as PET and SPECT (15) and MR spectroscopy (MRS) (16), are suggested for monitoring treatment effect and recurrence, as they add functional dimensions to brain scanning (Fig. 67.1).
PET Imaging
FDG is widely used for PET studies of brain lesion metabolism. The use of PET to grade tumor malignancy is based on the assumption that malignant tumors have high FDG uptake and benign tumors have reduced FDG uptake (17) compared with the average value of brain FDG uptake. FDG demonstrates enhanced uptake in the majority of malignant tumors, and the uptake is positively correlated with tumor malignancy in childhood CNS tumors (18,19,20). The diagnostic value of FDG-PET for grading malignancy in adults has previously been investigated (21,22,23,24,25). The studies showed that the specificity for malignancy grading was not sufficient, as a great deal of overlap between high-grade and low-grade tumors existed; however, PET-MRI coregistration and image fusion was shown to improve the accuracy of malignancy grading with FDG-PET (26,27).
A limited number of PET studies on CNS tumors in children have been published (18,19,20,24,28,29,30,31,32,33,34,35). Four small retrospective pre- and post-therapeutic studies (19,20,32,33) and two with a pretherapeutic prospective design (18,34) all reported a potential clinical diagnostic value for FDG-PET. Four of these studies were of malignancy grading (18,19,20,34), and they found a correlation between FDG and tumor malignancy, but with an overlap making malignancy grading difficult. One of these studies systematically combined PET with MRI coregistration and image fusion and showed an improved diagnostic value for FDG-PET in malignancy grading. Digitally performed coregistration and image fusion of PET with MRI was shown to increase the specificity in 90% of cases in terms of tumor location, tumor extent, and of heterogeneity (Figs. 67.2 and 67.3) (18). However, the remaining problem to be solved is still the hypermetabolic benign tumor (31).
The significant physiological FDG uptake in normal gray matter is a potential source of error in the interpretation of FDG-PET studies. Therefore, other tracers with higher tumor-to-background contrast, such as labeled amino acids, have been proposed as alternatives in preoperative malignancy grading, but the amino acids seem more useful for differentiation between low-grade neoplastic and non-neoplastic lesions than for tumor grading (34,35,36).
FDG-PET has also been proposed as a tool to improve the quality of biopsies from brain tumors in adults (37) and in children (30), but the delineation of tumor is difficult in the cerebral cortex. Carbon 11 [11C]methionine (MET) PET provides additional information in terms of
delineation of tumor extent for local management of pediatric brain tumors (34,38). Recently O-(2-[18F]fluoroethyl)-L-tyrosine (FET) PET has been found useful for biopsy guidance in adults (39). No pediatric publications concerning this issue are yet available.
delineation of tumor extent for local management of pediatric brain tumors (34,38). Recently O-(2-[18F]fluoroethyl)-L-tyrosine (FET) PET has been found useful for biopsy guidance in adults (39). No pediatric publications concerning this issue are yet available.
FDG-PET has been used to differentiate post-treatment contrast enhancement on MRI from recurrent tumor (40,41,42,43,44). Some studies in adults report a high accuracy (40,41,45,46,47), whereas others report that PET is neither sensitive enough nor specific enough to be used routinely (48,49). FDG-PET has also been used to describe the metabolic effects of various therapies on brain tumor metabolism (17,50,51,52,53) in adults, and a number of small clinical adult trials have indicated that quantification of changes in FDG uptake may provide an early and sensitive dynamic marker of the effect of chemotherapy and radiotherapy (50). Only a few studies have investigated the possible value of FDG-PET in the monitoring and diagnosing of
recurrent disease in childhood tumors. Plowman et al. found FDG-PET to be useful in the distinction of active tumor from post-treatment sequel in ten young patients with different brain tumors (33). In a study by Holthoff et al. that included 15 children and young adults (range, 0.5 to 26.0 years of age) with histologically confirmed brain tumors, FDG-PET was found to be useful for evaluating the metabolic activity of pediatric brain tumors over time and for assessing the response to treatment (20). Borgwardt et al. found FDG-PET with MRI coregistration to be useful for monitoring hypermetabolic childhood brain tumors (54).
recurrent disease in childhood tumors. Plowman et al. found FDG-PET to be useful in the distinction of active tumor from post-treatment sequel in ten young patients with different brain tumors (33). In a study by Holthoff et al. that included 15 children and young adults (range, 0.5 to 26.0 years of age) with histologically confirmed brain tumors, FDG-PET was found to be useful for evaluating the metabolic activity of pediatric brain tumors over time and for assessing the response to treatment (20). Borgwardt et al. found FDG-PET with MRI coregistration to be useful for monitoring hypermetabolic childhood brain tumors (54).
MET-PET was found to be useful for evaluating post-treatment contrast enhancement in childhood CNS tumors in general (34,35). However, this tracer has considerable nonprotein metabolism, since a significant fraction seems to be incorporated into phospholipids through the S-adenyl-methionine pathway and to generate substantial amounts of nonprotein metabolites. This makes quantification of protein synthesis difficult if required (55). Other amino acids, such as [11C]tyrosine and [11C]leucine, have been proposed as better protein synthesis rate imaging agents, but clinical experience with these radiotracers is still limited. Carbon 11–labeling is a limiting factor for routine clinical use and for regional distribution, because of the shorter half-life. Tyrosine can also be labeled with 18F, with high yields and specific activity, and 18F-ethyl-tyrosine appears promising as replacement for MET and as a complement for FDG in tumor diagnosis.
Recent studies have shown the potential usefulness of [11C]choline in brain tumors (56,57); it may be helpful in discriminating between benign and malignant brain tumors (58). Choline analogs are phospholipid precursors and have been shown by MRS to be present in increased concentrations in brain tumors, particularly high-grade lesions, probably representing the activation of choline uptake and phosphorylation in tumor cells. Choline metabolism in tumor cells is directed primarily toward membrane synthesis, de novo synthesis of choline is negligible in tumor cells, and the cell membranes are duplicated at the same rate as cell duplication. The recently developed [18F]choline is believed to be superior to 11C-labeled choline because of its longer half-life and shorter positron range (59), making synthesis and patient handling much easier. No pediatric studies are yet available.
In addition, studies have begun evaluating tumor proliferation imaging with radiolabeled nucleoside analogs that measure DNA synthesis and thereby tumor cell proliferation as a noninvasive means of assessing tumor growth potential and grade of malignancy and of identifying the most rapidly proliferating regions of the tumor. The radiolabeled nucleoside analogs [18F]-3′-flouro-thymidine (60) and the [2′-deoxy-2′-[18F]fluoro-beta-D-arabinofuranosyl nucleosides (61) seem promising, but studies on the use of these tracers for human brain tumor imaging have not yet been published.
Most of the interest in PET lies in the labeling of specific molecules such as drugs. An example is [11C]temozolomide, whose differential uptake in tumor and uninvolved brain can be determined over time as a way to assess regional kinetics (62). These studies suggest that PET has the potential to improve the clinical outcome of childhood brain tumors even more at different stages of the disease by increasing the accuracy of the choice or targeting of therapy. This becomes more important as the range of available tracers evolves.
SPECT Imaging
SPECT scans generally have poorer resolution than PET, but the use of this modality expands with the number of tracers available and hence with the targets that can be probed.
SPECT has been established as potentially useful tool for the assessment of brain tumors (63,64,65). In adults with gliomas, SPECT using thallium 201 (201Tl) has been applied to predict histological grade (66) and help differentiate between residual tumor and radiation necrosis (67). Although some studies have suggested a similar usefulness for thallium SPECT in childhood brain tumors (63,68), other prospective studies have failed to demonstrate any clinical advantage over MRI (69). In a comparison of [201Tl]chloride SPECT and FDG-PET in childhood brain tumors, Maria et al. stated that thallium SPECT images could be interpreted in 18 of 19 patients without MRI confirmation, whereas none of the 19 FDG-PET studies could be interpreted without MRI (70). The authors concluded that thallium SPECT seems to be the most promising imaging modality in this area. In a small group of patients with spinal cord tumors, FDG-PET did not provide additional useful information.
SPECT has been established as potentially useful tool for the assessment of brain tumors (63,64,65). In adults with gliomas, SPECT using thallium 201 (201Tl) has been applied to predict histological grade (66) and help differentiate between residual tumor and radiation necrosis (67). Although some studies have suggested a similar usefulness for thallium SPECT in childhood brain tumors (63,68), other prospective studies have failed to demonstrate any clinical advantage over MRI (69). In a comparison of [201Tl]chloride SPECT and FDG-PET in childhood brain tumors, Maria et al. stated that thallium SPECT images could be interpreted in 18 of 19 patients without MRI confirmation, whereas none of the 19 FDG-PET studies could be interpreted without MRI (70). The authors concluded that thallium SPECT seems to be the most promising imaging modality in this area. In a small group of patients with spinal cord tumors, FDG-PET did not provide additional useful information.
SPECT has also been used for monitoring of tumor activity in low-grade gliomas of childhood. Molenkamp et al. found 123I-alpha-methyltyrosine SPECT to be superior to CT, MRI, and FDG-PET in a small retrospective comparative trial (28).
It has been suggested that [99mTc]methoxyisobutylisonitrile (MIBI), or [99mTc]sestamibi, offers advantages over thallium for imaging of brain tumors (71). This molecule does not penetrate the intact blood–brain barrier and is taken up by normal choroid plexus, pituitary, scalp, and nasopharyngeal tissues (63). 99mTc-MIBI uptake by viable tumor cells and brain malignancies is well established (63). Human brain malignancies, such as astrocytoma, show accumulation of 99mTc-MIBI (71). 99mTc-MIBI-SPECT can add valuable information to CT for the differentiation of radiation necrosis from recurrent disease in adult brain malignancy (72). Despite the potential advantages and some experience with 99mTc-MIBI-SPECT in children, a clear advantage over other modalities, including 201Tl-SPECT, in imaging brain tumors has not been established (63,68,73).
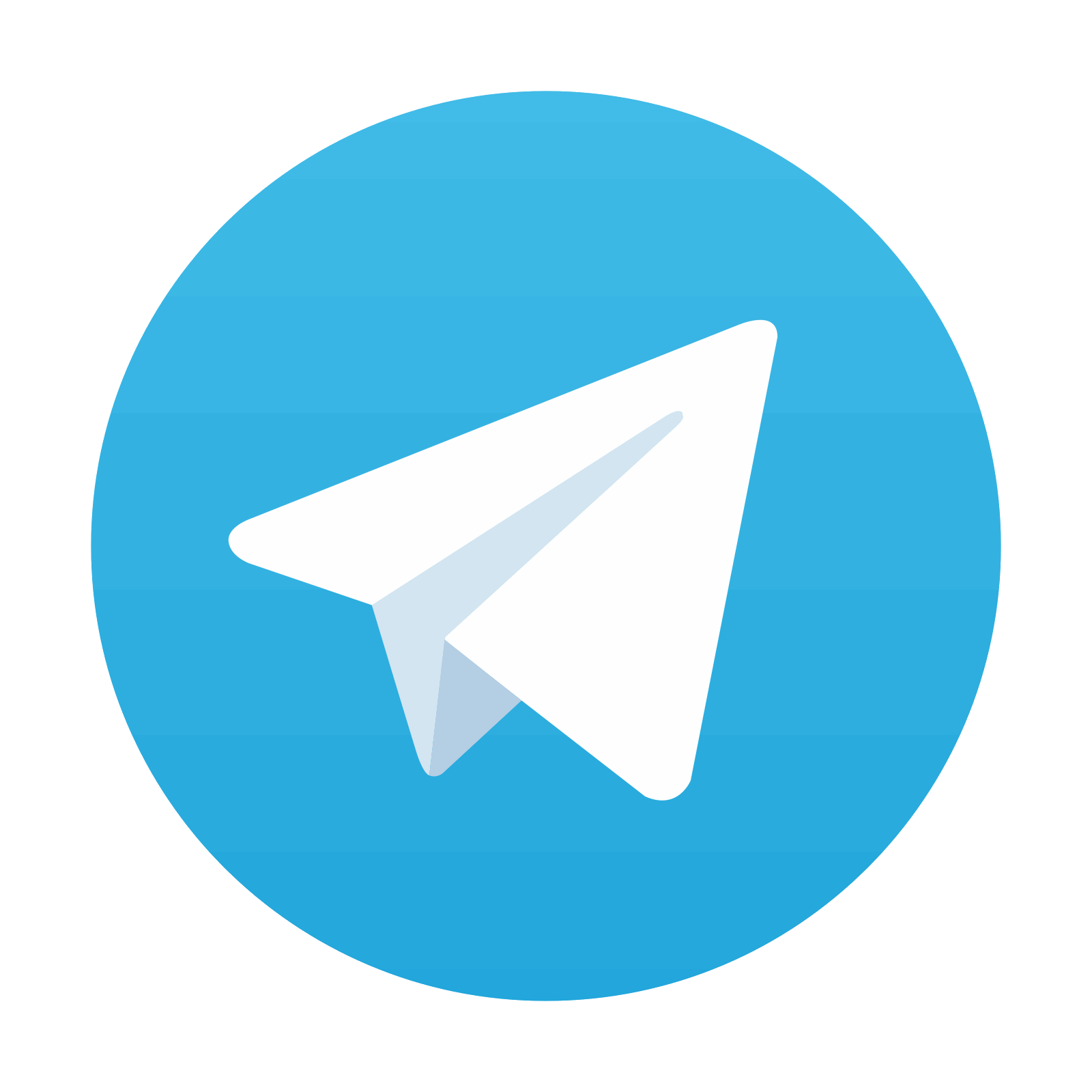
Stay updated, free articles. Join our Telegram channel

Full access? Get Clinical Tree
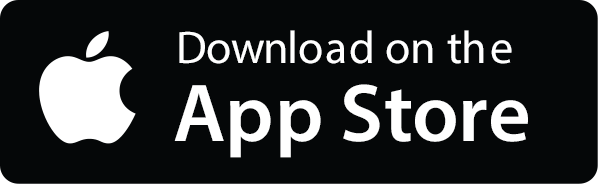
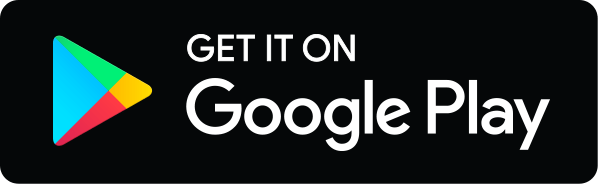