Authors
N
Diagnosis
Time since insult (month(s))
Main findings
Levy et al. (1987)
7
UWS
1–72
60 (53–67 %) decrease in metabolism
DeVolder et al. (1990)
7
UWS
1–4
53 % (43–65 %) decrease in metabolism
Tommasino et al. (1995)
10
UWS
2–24
56 % decrease in metabolism
Plum et al. (1998)
3
UWS
50 % decrease in metabolism with significant regional variations
Laureys et al. (1999a)
4
UWS
<1–60
Frontoparietal hypometabolism and disconnections
Laureys et al. (1999b)
1
UWS
1
38 % decrease in metabolism; recovery of consciousness = frontoparietal recovery
Rudolf et al. (1999a)
24
UWS
<1–6
25 % decrease in metabolism (17 % for acute patients (<3 months) and 33 % for chronic patients (>3 months))
Schiff and Plum (1999)
1
UWS
240
50 % decrease in metabolism with significant regional variations
Laureys et al. (2000b)
1
UWS
4
Recovery of consciousness = thalamocortical reconnections
Rudolf et al. (2000)
9
UWS
<1
Decrease in benzodiazepine receptor density in VS/UWS
Laureys et al. (2002a)
30
UWS
1–5
56 % (37–72 %) decrease in metabolism
Schiff et al. (2002)
5
UWS
6–240
50 % (31–87 %) decrease in metabolism with regional variations
Beuthien-Baumann et al. (2003)
16
UWS
2–12
58 % decrease in metabolism; frontoparietal hypometabolism
Tengvar et al. (2004)
1
MCS
6
47–65 % frontoparietal hypometabolism
Juengling et al. (2005)
5
UWS
1–48
Frontoparietal and thalamic hypometabolism
Nakayama et al. (2006)
30
17 UWS 13 MCS
6–60
Frontoparietal and thalamic hypometabolism in VS/UWS
Less impaired in MCS
Majerus et al. (2009)
36
MCS
1–265
Hypometabolism in language-processing regions (left superior, middle and inferior temporal gyri, left inferior frontal gyrus, and the right inferior temporal gyri)
Schnakers et al. (2008)
1
MCS
24
Changes in the fronto-temporo-parietal network and the sensorimotor area
Silva et al. (2010)
10
UWS
2–24
Frontoparietal hypometabolism
Lull et al. (2010)
17
UWS and MCS
12
Thalamus hypometabolism
Bruno et al. (2010)
10
UWS
3
Frontoparietal hypometabolism (VS/UWS without fixation = patients with fixation)
Phillips et al. (2011)
13 8
UWS LIS
<1–290
Automatic classification between VS/UWS and healthy controls or LIS using the “relevance vector machine”
Garcia-Panach et al. (2011)
17
UWS and MCS
12
Cortico-thalamo-cortical hypometabolism correlate with less favorable outcomes
Bruno et al. (2012)
27
13 MCS
1–70
Left-sided metabolism MCS+ (command following) >MCS− (low level of nonreflexive behaviors)
14 MCS+
Broca’s region disconnection from language network, mesiofrontal, and cerebellar areas in MCS-
Thibaut et al. (2012)
70
24 UWS
<1–270
Extrinsic and intrinsic network hypometabolism in VS/UWS
28 MCS
Intrinsic network hypometabolism in MCS
Table 47.2
Summary of PET-H2O15 studies on patients with disorders of consciousness during sensory stimulations
Authors | N | Diagnosis | Time since insult (day(s) and month(s)) | Passive stimulation | Level of activation |
---|---|---|---|---|---|
De Jong et al. (1997) | 1 | UWS | 2 months | Auditory (familiar voice) | High |
Menon et al. (1998) | 1 | UWS | 3 months | Visual (familiar face) | Low |
Laureys et al. (2000a) | 5 | UWS | 3–38 days | Auditory (click) | Low |
Laureys et al. (2002b) | 15 | UWS | 1 month | Pain (electrical stimulation) | Low |
Schiff et al. (2002) | 5 | 3 UWS 2 UWS | 6–300 months | Auditory (click), tactile | Low high |
Owen et al. (2002) | 3 | 1 UWS 2 UWS | 4 months | Visual (familiar face), auditory (noise, words) | Low high |
Kassubek et al. (2003) | 7 | UWS | 3–48 months | Pain (electrical stimulation) | High |
Boly et al. (2004) | 20 | 15 UWS 5 MCS | 1–4 months | Auditory (click) | Low high |
Laureys et al. (2004b) | 1 | MCS | 6 months | Auditory (noise, cries, own name) | High |
Owen et al. (2005) | 1 | UWS | 4 months | Auditory (speech) | High |
Giacino et al. (2006) | 5 | UWS | 1–3 months | Visual (flash) | Low |
Boly et al. (2008a) | 20 | 15 UWS 5 MCS | 1–4 months | Pain (electrical stimulation) | Low high |
Silva et al. (2010) | 5 | UWS | 2–22 months | Tactile | Low |
Table 47.3
Summary of PET studies on healthy subjects during sleep
Authors | N | Stage | Main findings | |
---|---|---|---|---|
Maquet et al. (1990) | 11 | FDG | SWS | Metabolism decrease 40 % (> thalami) |
REMS | Metabolism = wakefulness | |||
Maquet et al. (1996) | 7 | FDG | REMS | Metabolism increase in tegmentum, left thalamus, amygdala, ACC, and right parietal operculum |
Metabolism decrease in dorsolateral prefrontal, parietal, PCC, and precuneus | ||||
Braun et al. (1997) | 37 | H2O15 | SWS REMS | Metabolism decrease in centrencephalic and heteromodal association regions and increase in S1-S2 |
Metabolism increase in centrencephalic and S1-S2 and decrease in the frontoparietal association cortices | ||||
Hofle et al. (1997) | 11 | H2O15 | SWS | rCBF negatively correlate with sigma power (EEG) in the thalamus bilaterally |
Maquet (1997) | 11 | H2O15 | SWS | Metabolism decrease in dorsal pons and mesencephalon, thalami, basal ganglia, basal forebrain/hypothalamus, orbitofrontal cortex, anterior cingulate cortex, and precuneus |
Andersson et al. (1998) | 19 | H2O15 | SWS | Metabolism decrease in the thalamus and the frontal and parietal association areas and increase in the cerebellum |
Kajimura et al. (1999) | 18 | H2O15 | SWS | Metabolism decrease in midbrain tegmentum, cerebellar vermis, basal forebrain, caudate nucleus, posterior cingulate gyrus, and neocortical regions |
Nofzinger et al. (1997) | 8 | FDG | REMS | Metabolism increase in limbic regions and orbitofrontal, cingulate, entorhinal, and insular cortices |
Peigneux et al. (2001) | 12 | H2O15 | REMS | Correlation during REMS between the density of REMS and rCBF in the occipital and the lateral geniculate bodies of the thalamus |
Nofzinger et al. (2002) | 14 | FDG | SWS | Metabolism decrease in frontal, parietal, temporal and occipital cortices and thalamus |
Increases in the dorsal pontine tegmentum, hypothalamus, basal forebrain, ventral striatum, anterior cingulate cortex, and mesial temporal lobe | ||||
Dang-Vu et al. (2005) | 23 | H2O15 | SWS | Association between rCBF in the ventromedial prefrontal regions and delta power (EEG) |
Maquet et al. (2005) | 207 | H2O15 | REMS | Metabolism decrease in frontal and parietal areas |
Table 47.4
PET studies on hypnosis, at rest and during pain stimulation
Authors | N | PET | Paradigm | State | Main findings |
---|---|---|---|---|---|
Maquet (1999) | 9 | H2O15 | Rest | Hypnotic state | Widespread metabolism increase, mainly left sided (occipital, parietal, precentral, premotor, and ventrolateral prefrontal cortices) |
Rainville et al. (1999b) | 10 | H2O15 | Pain | Hypnotic suggestion | Increased metabolism in ACC and in the inferior frontal gyri |
Decreased metabolism in the right inferior parietal lobule, the left precuneus, and the posterior cingulate gyrus | |||||
Rainville et al. (1997) | 11 | H2O15 | Pain | Hypnotic suggestion | Increase in ACC |
Rainville et al. (1999a) | 22 | H2O15 | Pain | Hypnotic state | Increase in ACC, insula, S1 and S2 |
Faymonville et al. (2000) | 11 | H2O15 | Pain | Hypnotic state | Metabolism increase in right-sided extrastriate area and the ACC |
Hofbauer et al. (2001) | 10 | H2O15 | Pain | a. Hypnotic state | a. Activation of S1 and S2, ACC, and insula |
b. Hypnotic suggestion | b. Significant increase in pain-evoked activity within S1 and ACC | ||||
Rainville et al. (2002) | 10 | H2O15 | Rest | Hypnotic state | Metabolism increase in ACC, thalamus, and pontomesencephalic brainstem |
Decrease in the inferior parietal lobule, the precuneus, and the posterior temporal cortices | |||||
Faymonville et al. (2003) | 19 | H2O15 | Pain | Hypnotic state | Increased functional connectivity between midcingulate cortex and insula, pregenual, frontal, pre-SMA, brainstem, thalamus, and basal ganglia |
Nusbaum et al. (2011) | 14 | H2O15 | Rest (patients with chronic low-back pain) | Hypnotic suggestion | Metabolism increase in frontotemporal, insula, caudate, acumens, lenticular nuclei, and ACC |
Derbyshire et al. (2004) | 33 | H2O15 | Rest | Hypnotic suggestion | Both pain and hypnotically induced pain experience and pain stimulation induced metabolic modulations within the thalamus, ACC, insula, and prefrontal and parietal cortices |
47.2 Disorders of Consciousness Following a Brain Injury
Following severe brain damage with loss of consciousness, patients usually evolve through different clinical stages before fully or partially recovering consciousness. Coma is defined as complete absence of arousal and awareness, with no eye opening and only presenting reflexive behavior, in other words, an “unarousable unresponsiveness” (Posner et al. 2007). When eye opening and rest-wake cycles are reestablished, but the patients still only exhibit reflexive behaviors, they are said to be in a vegetative state (VS), now called unresponsive wakefulness syndrome (UWS) (The Multi-Society Task Force on PVS 1994; Laureys et al. 2010). Progression from VS/UWS usually leads to the minimally conscious state, where reproducible but fluctuating signs of consciousness are present, such as eyes tracking motion or response to verbal or tactile stimuli (Giacino et al. 2002), but these patients are, by definition, unable to effectively communicate. We recently proposed a subcategorization of MCS patients based on the complexity of their behavior in two entities: “MCS minus” and “MCS plus.” “MCS minus” describes patients with minimal level of behavioral interactions without command following (e.g., visual pursuit, localization of noxious stimulation, and/or smiling/crying in contingent relationship to external stimuli). “MCS plus” patients show higher-level behavioral responses such as command following (Fig. 47.1) (Bruno et al. 2012). The emergence of the MCS is characterized by the recovery of functional communication (verbal or nonverbal) or functional use of objects (Giacino et al. 2002). In rare cases, following brainstem lesions, patients can fully recover consciousness but remain totally paralyzed and aphonic, in the aptly named locked-in syndrome (Laureys et al. 2005a). These patients are able to communicate only with small eye movements (American Congress of Rehabilitation Medicine 1995). The difficulty in disentangling reflexive from voluntary responses, the fluctuation of vigilance, and the additional cognitive or sensory deficits lead to a high rate of misdiagnosis between VS/UWS and MCS (Andrews et al. 1996; Childs and Mercer 1996; Schnakers et al. 2009) and locked-in syndrome (Bruno et al. 2009). The diagnosis may influence decisions about the patients’ care, regarding crucial matters such as end-of-life decisions (Demertzi et al. 2011) or pain management (Demertzi et al. 2009). In this context, objective measures independent of the integrity of the motor pathways are needed to supplement behavioral assessments at the bedside. Neuroimaging provides a promising means to this end (for a review, see Schiff (2006) and Laureys and Schiff (2012)).
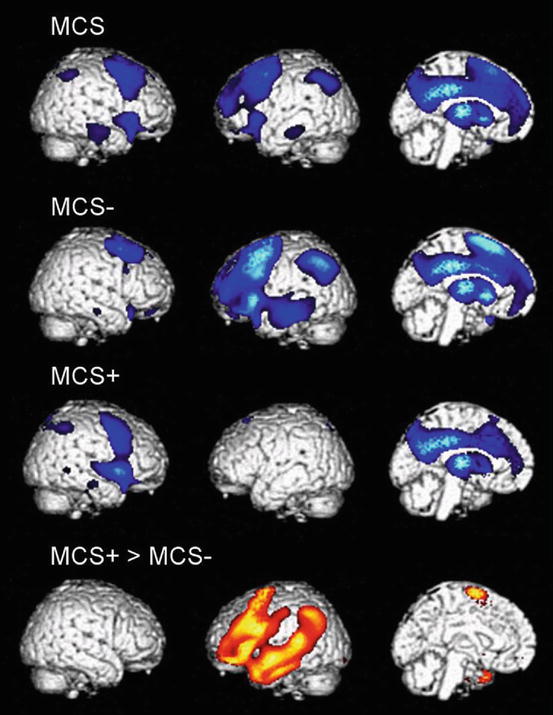
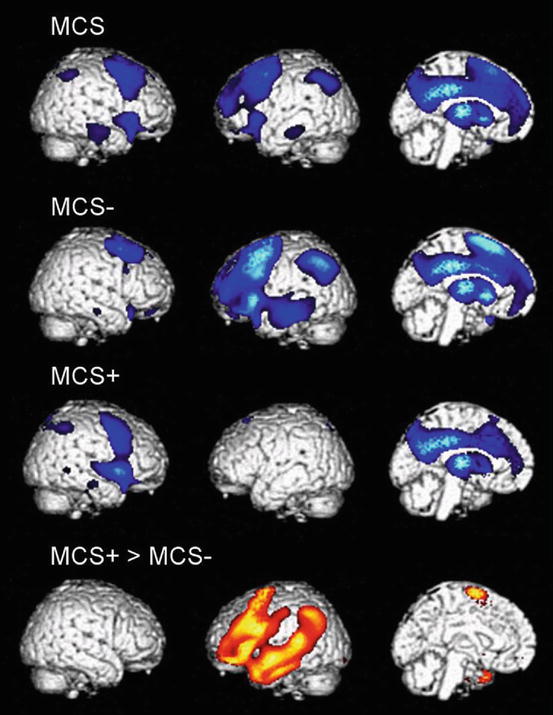
Fig. 47.1
Areas with impaired metabolism (shown in blue) in patients in a minimally conscious state (MCS), MCS− (showing nonreflex behavior), and MCS+ (showing command following). The lowest panel illustrates areas with higher metabolism in MCS + as compared to MCS− (shown in orange). All results are shown on a 3D MRI template and thresholded at false discovery rate corrected p < 0.05 (From Bruno et al. (2012))
47.3 PET Scan and Disorders of Consciousness
47.3.1 Measuring the Brain at “Rest”
In 1987, the first FDG-PET study on VS/UWS patients showed a global decrease in brain metabolism of about 40–50 % (Levy et al. 1987). These results were reproduced in several studies (DeVolder et al. 1990; Tommasino et al. 1995; Laureys et al. 1999b; Rudolf et al. 1999b). Patients in locked-in syndrome had brain metabolism levels closer to normal than VS/UWS patients (Levy et al. 1987). Results from studies by Laureys et al. indicated that no supratentorial cortical areas show significantly lower metabolism in acute and chronic locked-in patients when compared to age-matched healthy controls (Laureys et al. 2003, 2004a, 2005a). However, the global brain metabolism is not directly correlated with the level of consciousness. A recovery from VS/UWS does not always imply substantial changes in global brain metabolism (Laureys et al. 1999b). Moreover, some healthy controls show metabolic rates of glucose comparable to VS/UWS patients (Laureys 2005). Those results suggest that some brain areas are more important for the emergence of consciousness than others (Laureys et al. 1999b, 2000c, 2004a). Studies on regional brain metabolism were performed to identify areas specifically involved in loss of consciousness, comparing brain metabolism of VS/UWS and MCS patients with age-matched healthy controls. Results highlighted a widespread impairment of the frontoparietal network, encompassing midline (i.e., anterior cingulate cortex (ACC)/mesiofrontal and posterior cingulate cortex (PCC)/precuneus) and lateral (i.e., prefrontal and posterior parietal) associative cortices being associated with a decreased level of consciousness (Laureys et al. 1999a, b; Beuthien-Baumann et al. 2003; Juengling et al. 2005; Nakayama et al. 2006; Lull et al. 2010; Silva et al. 2010; Bruno et al. 2012; Thibaut et al. 2012). Preserved areas were the midbrain and brainstem structures, known to be involved in autonomous functions such as sleep-wake cycles, thermoregulation, and respiration (Laureys et al. 2002a). Moreover, functional connectivity studies also highlighted a cortico-cortical (Laureys et al. 1999a, 2000b, 2002b) and thalamocortical (Laureys et al. 2000b) disconnection syndrome in VS/UWS patients. A recent study showed that a cortico-thalamo-cortical hypometabolism seems to be correlated with less favorable outcome (Garcia-Panach et al. 2011). These observations led to the hypothesis that consciousness is an emergent property of frontoparietal functional connectivity (Baars et al. 2003; Laureys 2005). A recent clinical trial (Giacino et al. 2012) has shown effects of amantadine, a mixed noradrenergic and dopaminergic agonist, toward improving the speed of recovery from disorders of consciousness. A case study reported by Schnakers et al. highlighted effects on brain metabolism of the drug, in a widespread fronto-temporo-parietal network and the sensorimotor area (Schnakers et al. 2008). These data suggest a modulation of polymodal associative cortical metabolism and motor function by amantadine. Alongside, they underline the behavioral improvements associated with amantadine treatment (e.g., recovery of command following). Within this frontoparietal network, the precuneus seems to be a critical region (Vogt and Laureys 2005). This area is the most active in conscious resting states (Raichle and Snyder 2007) and seems to be the most widely impaired in patients with disorders of consciousness (Laureys et al. 2005b). A study on traumatic brain-injured patients found a hypometabolism in the ACC and PCC, the medial frontobasal regions, and the thalamus. The metabolism in these regions was mostly impaired in VS/UWS patients compared to MCS. Moreover, hypometabolism was more widespread and prominent in the MCS group as compared to patients who had emerged from the MCS (Nakayama et al. 2006). Within this network, it seems possible to distinguish areas involved in external (related to external/sensory awareness) or internal consciousness (related to internal/self-awareness) (Laureys et al. 1999a; Tian et al. 2007; Boly et al. 2008b; Vanhaudenhuyse et al. 2011). These results demonstrated that both external (encompassing lateral frontoparietal cortices) and internal (midline areas) networks were impaired in VS/UWS patients, while MCS patients only had decreased metabolism in the internal network, even less affected in patients emerging from the MCS (Thibaut et al. 2012).
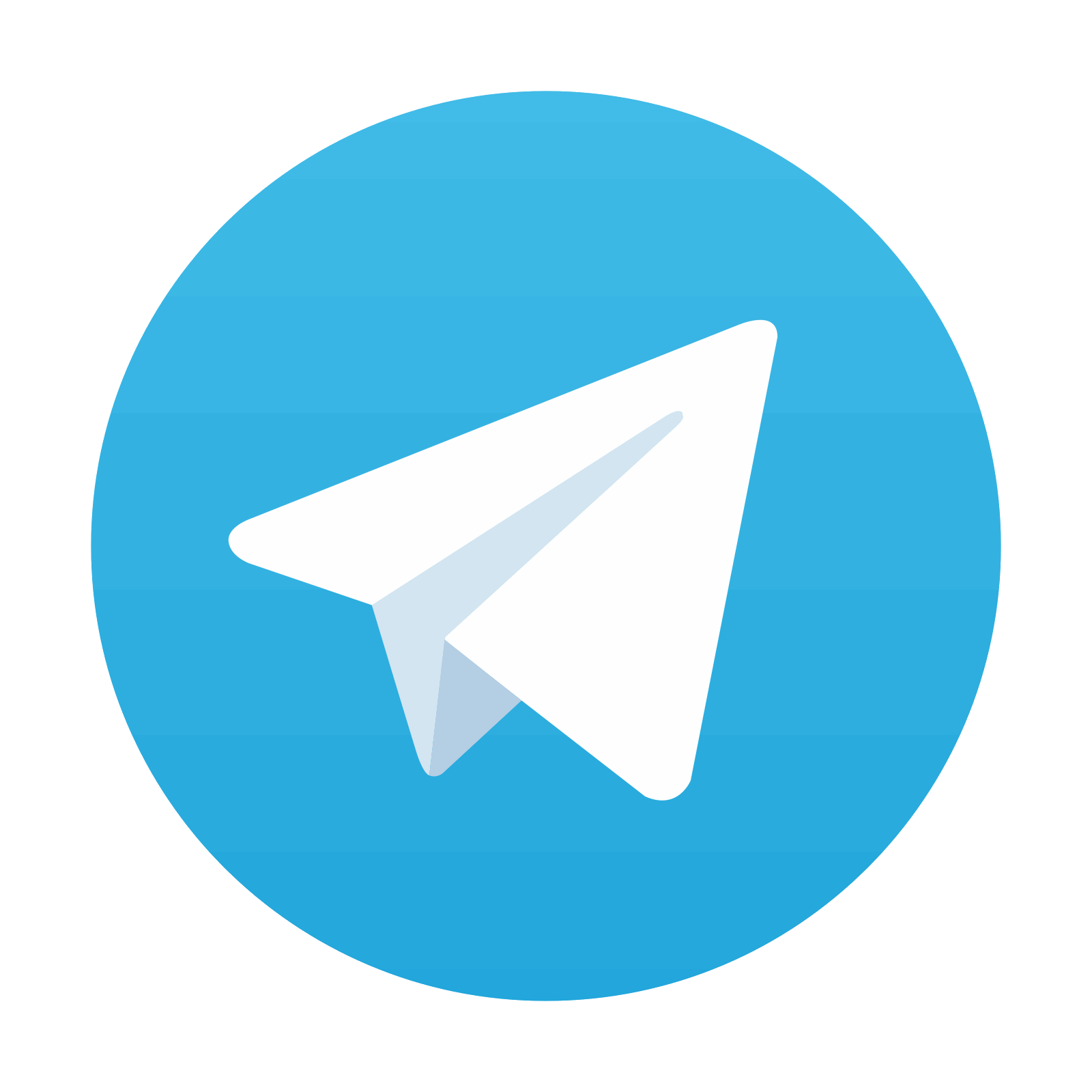
Stay updated, free articles. Join our Telegram channel

Full access? Get Clinical Tree
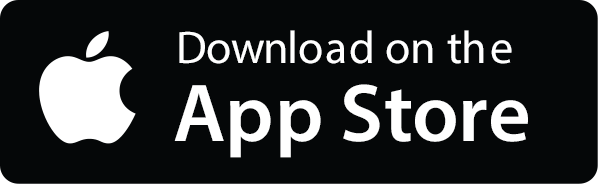
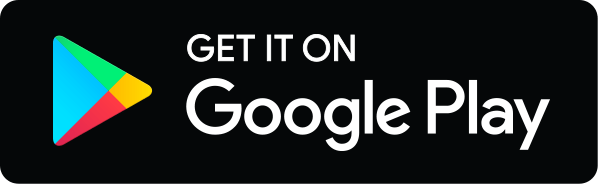