Fig. 25.1
Sequential PET images of CBF, CMRO2, and OEF of MCA occlusion in cats compared to images of patients after stroke. Left columns: in the left cat, the progressive decrease of CMRO2 and the reduction of OEF predict infarction and cannot benefit from reperfusion. Only if OEF is increased until start of reperfusion it can be salvaged (right cat). Middle columns: in the patient, the areas with preserved OEF are not infarcted and can survive in spontaneous course (posterior part of ischemic cortex in left, anterior part in right patient as indicated on late MRI and CT). Right columns: in patients receiving rtPA treatment, measurements of CMRO2 and OEF are not feasible, but flow determinations show the effect. If reperfusion occurs early enough and before tissue damage, tissue can be salvaged (left patient). If reperfusion is achieved too late, tissue cannot be salvaged despite hyperperfusion in some parts (right patient)
Flow measurements in the first hours after a stroke permitted to identify various compartments of tissue and their contribution to the final infarct on CT/MRI. If the threshold for probable infarction was set to the conventional value of 12 ml/100 g/min and that for the upper limit of penumbra to 18 ml/100 g/min, a large compartment of the final infarct (70 %) was perfused below 12 ml/100 g/min, i.e., at the level predicting necrosis; a smaller portion (18 %) had flow values in the penumbra range (12–18 ml/100 g/min); and a fairly small compartment (12 %) had perfusion at a higher level (Heiss et al. 1999b).
Measurement of blood flow values and determination of oxygen extraction fraction by PET require arterial blood sampling, and the clinical applicability is further limited by the complex logistics and instrumentation involved; isolated flow determinations at a single time point might be confusing as long as the pattern over time is not known. A marker of neuronal integrity is needed that can identify irreversibly damaged tissue irrespective of the time elapsed since the vascular attack and irrespective of the variations in the blood flow over time, which also does not require arterial blood sampling. The central benzodiazepine receptor ligand flumazenil (FMZ) binds to the GABA receptor abundant in the cerebral cortex. These receptors are sensitive to ischemic damage and can therefore be used to identify early neuronal loss. In transient MCA occlusion in cats, irrespective of the level of reperfusion, deficits in FMZ binding 2–3 h after MCAO were closely related to areas with severely depressed oxygen consumption and predicted the size of the final infarcts, whereas preserved FMZ binding indicated an intact cortex (Fig. 25.2a) (Heiss et al. 1997). Using FMZ as a marker of neuronal integrity and H2 15O for flow determinations, the pathophysiological changes early after ischemic stroke could be accurately specified: 55 % of the volume of the final infarct had FMZ uptake decreased below the limit of 95 % probability for infarction in the first hours after stroke; 21 % of the final infarct had flow below 14 ml/100 g/min, the 95 % probability threshold for survival in this study, but FMZ uptake above the critical value, thereby indicating penumbra tissue (Fig. 25.2b). In only 13 % of the final infarct, neuronal integrity was indicated by FMZ and CBF values were above the penumbra range. These results indicate the potential and the limits of therapy in acute stroke: early reperfusion cannot reverse already developed infarction, but is crucial for salvaging the penumbra; the small compartment which is sufficiently perfused might be damaged by delayed mechanisms and might benefit from neuroprotective measures.
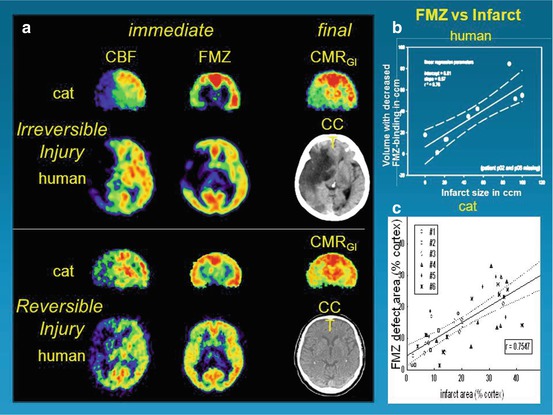
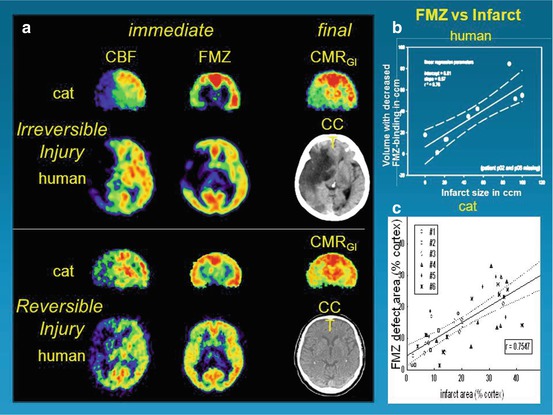
Fig. 25.2
Flumazenil as a molecular marker of neuronal integrity and for prediction of effect of reperfusion therapy. (a): PET images of early CBF and FMZ binding immediately following 1-h transient occlusion in cats or just before the start of thrombolysis in patients. Upper trace: both the cat and the human study demonstrate good correspondence of early deficits detected in FMZ images with final deficits depicted in CMRglc and CCT images, respectively. Lower trace: in both cat ischemia and human stroke, moderate perfusional disturbance does not lead to severe deficits in FMZ binding in the early stage. Consequently, only minor final deficits, depicted in CMRglc and CCT images, develop. (b): Linear regression between final infarct volume on MRI/CT and volume of decreased FMZ binding on initial PET. Broken lines indicate 95 % confidence limits. (c): Comparison between size of infarct ~ 15 h after MCAO and extent of defect in FMZ uptake 2–3 h after reperfusion following 1-h MCAO. Size of altered regions in relation to entire cortical regions of respective hemisphere (% cortex) is shown (number of compared regions per individual cat depends on histological sections that could be matched to PET images)
25.3 Comparison of PET and PW/DW MRI
Although PET remains the imaging gold standard for identification of the penumbra in stroke patients, MR studies using diffusion and perfusion imaging might provide a differentiation between the core and the penumbra: the early diffusion-weighted imaging (DWI) lesion might define the ischemic core and adjacent critically hypoperfused tissue might be identified with perfusion-weighted imaging (PWI) (Baird et al. 1997; Barber et al. 1998). Therefore, brain regions with hypoperfusion assessed by PWI but without restricted diffusion (PWI/DWI mismatch) were assumed to represent the penumbra. This surrogate definition of the penumbra has several uncertainties (Kidwell et al. 2003): the initial diffusion lesion does not only consist of irreversibly infarcted tissue; diffusion lesions may be reversed if blood flow is restored at an early time point. Critically perfused tissue (i.e., penumbra) cannot be clearly differentiated from tissue experiencing oligemia; the PWI abnormality often overestimated the amount of tissue at risk.
Several studies were performed in order to validate mismatch as a surrogate of penumbra on PET-derived discrimination of irreversibly damaged, critically perfused “at risk” and oligemic “not at risk” tissue. The studies demonstrated that the DWI lesion predicts more or less the finally infarcted tissue (Fig. 25.3) (Heiss et al. 2004) but contains up to 25 % false positive, i.e., surviving tissue. The degree of disturbance of oxygen consumption was variable within individual DWI lesions suggesting variable potential for recovery (Guadagno et al. 2006).
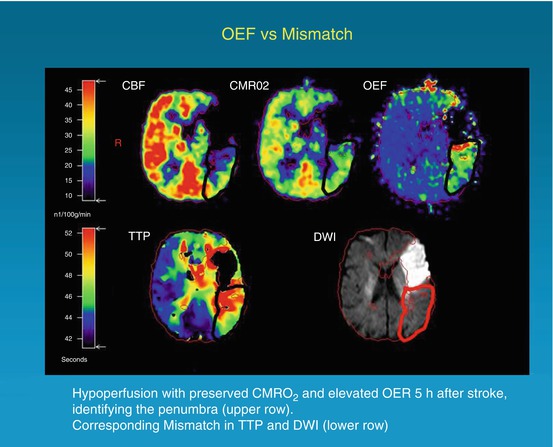
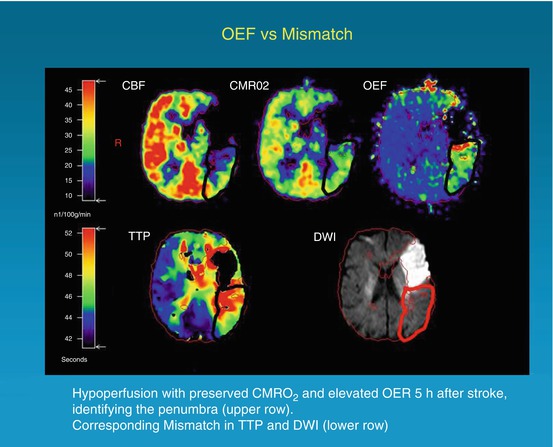
Fig. 25.3
Coregistered images of multitracer PET (upper row) and PW/DW MRI (lower row) in a patient with right-sided hemiparesis and expressive aphasia 5 h after symptom onset. The penumbra is characterized by hypoperfusion (CBF), preserved CMRO2, and elevated OEF. These findings correspond grossly to the mismatch between DWI hyperintensity and a TTP delay of 4 s compared with the unaffected hemisphere
The inaccuracy in defining the penumbra with PW/DWI mismatch is thought to be mainly related to PW data acquisition, which is a complex process, and the parameters used to estimate perfusion are variable and somewhat arbitrary (Ostergaard 2005). As a consequence, perfusion lesion size differs markedly depending on the parameters calculated (Kane et al. 2007) and usually is overestimated and extends into considerable areas with noncritical oligemia especially when short delays are used (Olivot et al. 2009). Time to peak delays of 4 and 6 s reliably identified hypoperfused and excluded normoperfused tissue (threshold arbitrarily set to 20 ml/100 g/min) but still overestimated the size of the critically perfused tissue (Sobesky et al. 2004) and therefore overestimated the volume of critically perfused but salvageable tissue, i.e., the penumbra (Sobesky et al. 2005): of 13 patients showing considerable PW/DWI mismatch, only eight had areas with elevated OEF typical for penumbra tissue, and these areas were always smaller on PET than on PW/DWI. Overall, the mismatch volume in PW/DWI as conventionally calculated does not reliably reflect misery perfusion, i.e., the penumbra as defined by PET. Several validation studies of various perfusion parameters calculated from PW MRI on quantitative flow values obtained from H215O-PET (Takasawa et al. 2008; Zaro-Weber et al. 2009) resulted in corrections permitting reliable classification of critical but potentially reversible ischemia (Tmax., CBF, time to peak). These thresholds have been implemented into recent clinical stroke trials in order to improve efficiency of therapeutic interventions (Olivot and Albers 2011). With the advance of arterial spin labeling (ASL) MR techniques (Hernandez et al. 2012) and of CT perfusion studies for the determination of cerebral perfusion (Campbell et al. 2011), it will be necessary to validate the results on flow values from quantitative methods.
25.4 PET as a Surrogate Marker for Treatment Efficiency
The efficacy of treatment in ischemic stroke can only be proven by controlled randomized double-blind clinical trials, as successfully performed for thrombolysis with iv rtPA (review in Lees et al. (2010)). Since such controlled trials require large patient populations collected in many stroke centers and therefore usually take long time and considerable funds, surrogate markers are applied to predict potential therapeutic effects in small groups of patients. It has to be kept in mind that proven effects on surrogate markers always must be confirmed in controlled trials based on sufficient patient populations. In recent years, identification of salvageable tissue by neuroimaging has gained much interest as a surrogate marker for treatment efficiency in stroke.
The effect of the only approved therapy for acute ischemic stroke was established also in imaging studies, in which reperfusion to penumbral tissue was followed by improvement in neurological deficits: reperfusion was significantly increased in rtPA-treated patients compared to controls (Grotta and Alexandrov 1998). The volume of tissue salvaged by reperfusion was established in a study in which CBF, as determined by H2 15O-PET within 3 h of stroke onset, was compared with the volume of infarction determined on MRI 3 weeks after the ictus (Heiss et al. 1998). The percentage of initially critically ischemic voxels (i.e., with a flow below the threshold of 12 ml/100 g/min) that became reperfused at almost normal levels clearly predicted the degree of clinical improvement achieved within 3 weeks. Overall, only 22.7 % of the gray matter that was initially perfused at rates below the conventional threshold of critical ischemia became necrotic after thrombolytic therapy in this small sample of 12 patients. This means that a considerable portion of the critically hypoperfused tissue was probably salvaged by the reperfusion therapy. Another PET study in 11 patients (Heiss et al. 2000) indicated that hypoperfused tissue could benefit from reperfusion only as long as cortical flumazenil binding was not reduced to or below 3.4 times the mean uptake in white matter. This marker of neuronal integrity can therefore serve as an indicator for irreversibly damaged tissue that is not amenable to treatment.
25.5 PET for Prediction of “Malignant Infarction”
A multitude of electrical and biological disturbances interact in the progression of irreversible cell damage in ischemia (review in Hossmann (2006)). Peri-infarct spreading depression-like depolarizations (PIDs) play an important role in triggering and continuously stimulating this pathogenic molecular/biochemical cascade of cell injury, they are related to stepwise increases in irreversible damage, and their frequency correlates to size of ischemic lesions in experimental ischemia (Nakamura et al. 2010). Their occurrence might predict a malignant course with large space-occupying infarcts in patients (Dohmen et al. 2008). Such malignant brain infarcts develop in about 10 % of patients with ischemic stroke in the middle cerebral artery (MCA) territory. Invasive treatment strategies, especially decompressive hemicraniectomy, might improve mortality and morbidity of these patients (Juttler et al. 2011). The selection of patients who can benefit and the determination of the best time for these interventions however are still controversial and require a better assessment of the extent of irreversible ischemic damage. In experimental MCA occlusion in cats, a malignant course was indicated by severe ischemia in 55–75 % of the hemisphere at the end of 3 h occlusion. Hyperperfusion after reopening of the MCA turned into hypoperfusion and finally led to severe global ischemia with transtentorial herniation. In the animals with a nonmalignant course, severe ischemia only covered 10–44 % of the hemisphere and recirculation only induced moderate hyperperfusion and delayed hypoperfusion. Intracranial pressure continued to rise in the malignant group throughout the observation period and reached values above 70 mmHg.
In 34 patients with ischemic changes of > 50 % of the MCA territory in early cerebral CT scans, PET was performed with 11C-FMZ to assess CBF and irreversible neuronal damage. Thereafter, probes for microdialysis and for measurement of intracerebral pressure and tissue oxygen pressure were placed into the ipsilateral frontal lobe (Dohmen et al. 2003). PET measurements within 24 h after stroke showed larger volumes of ischemic core (mean, 144.5 versus 62.2 cm3) and larger volumes of irreversible neuronal damage (157.9 versus 47.0 cm3) in patients with malignant course (i.e., edema formation with midline shift) than in patients with benign course (Fig. 25.4). Mean cerebral blood flow values within the ischemic core were significantly lower and the volume of the ischemic penumbra was smaller in the malignant than in the benign group. In patients with malignant course, cerebral perfusion pressure dropped to <50–60 mmHg, 22–72 h (mean, 52.0 h) after onset of symptoms; subsequently, PtO2 dropped and glutamate increased, indicating secondary ischemia. Maximal changes in the monitored variables reached significant levels for glutamate, aspartate, GABA, glycerol, lactate-to-pyruvate ratio, hypoxanthine, intracranial pressure, cerebral perfusion pressure, and PtO2 (Fig. 25.4). Corresponding to the experimental results, PEt allowed prediction of malignant MCA infarction within the time window suggested for hemicraniectomy. Neuromonitoring helped to classify the clinical courses by characterizing pathophysiological sequelae of malignant edema formation. In contrast to PET, however, it did not predict fatal outcome early enough for successful implementation of invasive therapies.
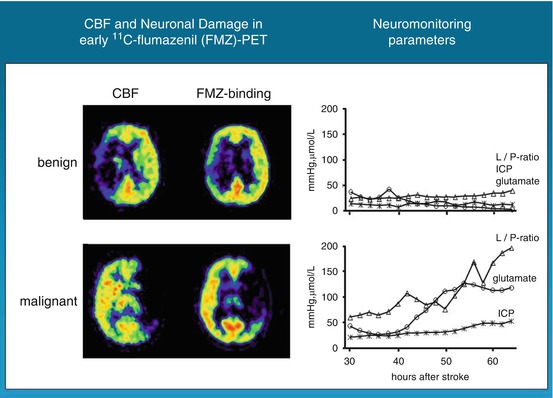
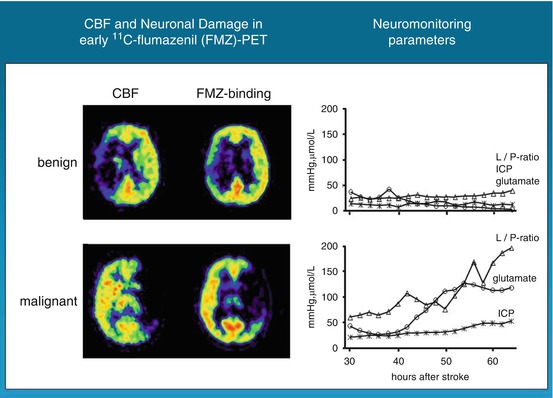
Fig. 25.4
FMZ distribution and FMZ binding in patients with large MCA infarcts: in benign infarcts, volume of severe hypoperfusion and reduced FMZ binding, i.e., neuronal damage, were smaller than in patients with malignant course. Neuromonitoring did not reveal significant deflections of variables, whereas in malignant patients, substrate concentrations and intracranial pressure rose progressively
25.6 Microglia Activation as an Indicator of Inflammation
Microglia constitutes up to 10 % of the total cell population of the brain. As resident macrophages of the central nervous system, microglia phagocytose cellular debris, present foreign antigens, and are sensors of pathological events including ischemia (Hanisch and Kettenmann 2007). Microglia changes from a resting to an activated state in response to central nervous system insults that stimulate them to function as phagocytes. In this activation process, they undergo a shift in their effector program by transforming their morphology, proliferating, releasing proinflammatory compounds, and increasing expression of immunomodulatory surface antigens (Weinstein et al. 2010). As one of the consequences, the translocator protein 18 kDa (TSPO), formerly known as the peripheral benzodiazepine receptor (PBR), is upregulated in mitochondria of activated microglia and may thus serve as a biomarker of inflammation. Several radioligands have been developed to image the activation of microglia in experimental models and in various diseases of the central nervous system (Thiel and Heiss 2011). Early studies in ischemia models using 3H-PK 11195 autoradiography demonstrated increased binding sites in the area of infarction and in the boundary zones between major arteries in hypertensive animals, which were associated with reactive glial cells and macrophages and reached a maximum 4–8 days after the induction of local ischemia. Applying high-resolution microPET to imaging expression of TSPO/PBR in transient experimental ischemia, a high signal was detected in the core of ischemia starting on day 4 and increasing to day 7, and this strong signal was related to microglia/macrophages. A less prominent signal indicating elevated TSPO expression was observed in the region surrounding the infarct at day 7, which could be attributed to reactive astrocytes. These results demonstrate cellular heterogeneity for TSPO/PBR expression depending on intrinsic features of inflammatory cells (Demerle-Pallardy et al. 1991; Rojas et al. 2007). In permanent ischemia in rats induced by macrosphere injection into the middle cerebral artery, no increase in 3H-PK 11195 binding was found in the infarct core 7 days after the attack, but the permanent middle cerebral artery ischemia caused increased tracer binding in the normoperfused peri-infarct zone, which was colocalized with an increased glucose metabolism and accumulated microglia and macrophages. This peri-infarct neuroinflammation might contribute to the extension of tissue damage (Schroeter et al. 2009).
Many histological studies have documented the presence of activated microglia in the ischemic brain after stroke in humans. Abundant reactive microglia/macrophages have been found in the ischemic core within 1–2 days after ischemic infarction. Over time they extended from the ischemic core into the peri-infarct zones (Weinstein et al. 2010). In several imaging studies, increased binding of 11C-PK11195 was observed around the outer border of ischemic lesions after several days (Fig. 25.5) but also in areas distant from the lesion (review in Thiel and Heiss (2011)). Increased tracer binding was also detected in the thalamus ipsilateral to the stroke and in the subcortical white matter tracts. This possible relationship between neuroinflammatory reaction and integrity of white matter tracts has recently been investigated more systematically by combining microglia positron emission tomography with diffusion tensor imaging (Fig. 25.5) (Radlinska et al. 2009). Diffusion tensor imaging acquires information about the anisotropic diffusion of water molecules along white matter fiber tracts, giving detailed information about tissue microstructure. Using diffusion tensor imaging-based definitions of the pyramidal tract, (Radlinska et al. 2009) demonstrated in a prospective controlled study that microglia activation occurs along the pyramidal tract anterograde to the lesion only in those patients with acute subcortical stroke, where the pyramidal tract was affected. These anterograde tract portions are those which will undergo Wallerian degeneration in the weeks and months after stroke. This relationship was further investigated in a similarly designed but longitudinal study (Thiel et al. 2010) in which the extent of anterograde microglia activity in the brain stem was found to be linearly related to the extent of pyramidal tract damage. This remote inflammatory activity was positively related with outcome, indicating a possible neuroprotective role or repair function of microglia cells along the tract portions undergoing Wallerian degeneration. In contrast, local microglia activity in the area of the infarct was only related to persisting tract damage in the chronic phase and correlated negatively with clinical outcome. On the other hand, the activation of microglia in the vicinity and in distant areas of an infarct might go along with increased deposition of amyloid leading to poststroke dementia. Experimental findings have identified a probable link between ischemia, inflammation, and amyloid deposition (Whitehead et al. 2007). This interaction is now investigated in a cooperative multitracer study in stroke patients applying 11C-PK11195 and PIB (Heiss et al. 2011).