Physical and Technical Fundamentals
General Principles of CT
Computed tomography is a special type of x-ray procedure that involves the indirect measurement of the weakening, or attenua-tion, of x-rays at numerous positions located around the patient being investigated. Basically speaking, all we know is
what leaves the x-ray tube,
what arrives at the detector and
the position of the x-ray tube and detector for each position.
Simply stated, everything else is deduced from this information. Most CT slices are oriented vertical to the body`s axis. They are usually called axial or transverse sections. For each section the x-ray tube rotates around the patient to obtain a preselected section thickness ( Fig. 6.1 ). Most CT systems employ the continuous rotation and fan beam design: with this design, the x-ray tube and detector are rigidly coupled and rotate continuously around the scan field while x-rays are emitted and detected. Thus, the x-rays, which have passed through the patient, reach the detectors on the opposite side of the tube. The fan beam opening ranges from 40° to 60°, depending on the particular system design, and is defined by the angle originating at the focus of the x-ray tube and extending to the outer limits of the detector array.
Typically, images are produced for each 360° rotation, permitting a high number of measurement data to be acquired and sufficient dose to be applied. While the scan is being performed, attenuation profiles, also referred to as samples or projections, are obtained. Attenuation profiles are really nothing other than a collection of the signals obtained from all the detector channels at a given angular position of the tube-detector unit. Modern CT systems ( Fig. 6.4 ) acquire approximately 1400 projections over 360°, or about four projections per degree. Each attenuation profile comprises the data obtained from about 1500 detector channels, about 30 channels per degree in case of a 50° fan beam. While the patient table is moving continuously through the gantry, a digital radiograph (“scanogramm” or “localizer”, Fig. 6.2 ) is produced on which the desired sections can be planned. For a CT examination of the spine or the head, the gantry is angled to the optimal orientation ( Fig. 6.3 ).
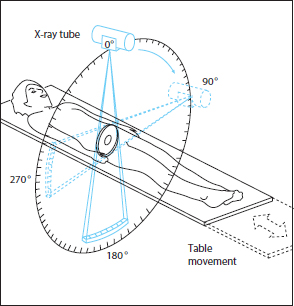
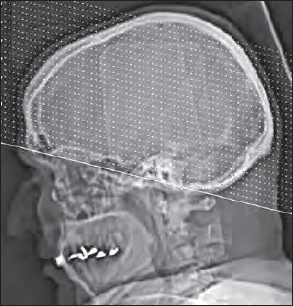
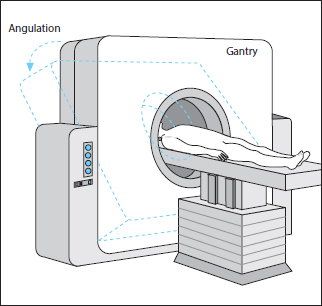
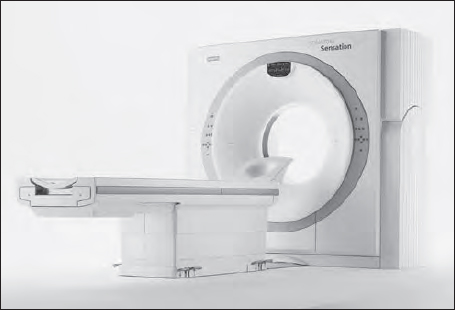
Multiple-Row Detector Spiral CT
Multiple-row detector CT (MDCT) is the latest scanner development. Rather than one detector row, multiple detector rows are placed opposite the x-ray tube. This shortens the examination time and improves the temporal resolution, allowing, for instance, the determination of the rate of vascular enhancement.
The detector rows along the z-axis opposite the x-ray tube are unequal in width, with the outer rows wider than the inner rows to provide better conditions for image reconstruction after data acquisition (see pages 9–11 and 206).
Dual Source CT
This newest technique features two detector units and two X-ray tubes in one gantry and is described in more detail on pages 198–201.
Comparison of Conventional CT with Spiral CT
In conventional CT, a series of equally spaced images is acquired sequentially through a specific region, e.g. the abdomen or the head ( Fig. 7.1 ). There is a short pause after each section in order to advance the patient table to the next preset position. The section thickness and overlap/intersection gap are selected at the outset. The raw data for each image level is stored separately. The short pause between sections allows the conscious patient to breathe without causing major respiratory artifacts.
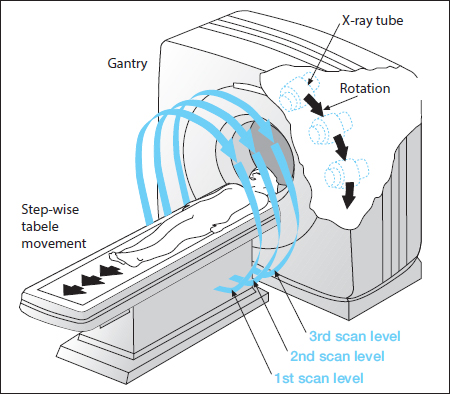
However, the examination may take several minutes, depending on the body region and the size of the patient. Proper timing of image acquisition after i.v. contrast media is particularly important for assessing perfusion effects. CT is the technique of choice for acquiring complete 2D axial images of the body without the disadvantages of superimposed bone and / or air as seen in conventional x-ray images.
Both single-row detector CT (SDCT) and multiple-row detector CT (MDCT) continuously acquire data of the patient while the examination table moves through the gantry. The x-ray tube describes an apparent helical path around the patient ( Fig. 7.2 ). If table advance is coordinated with the time required for a 360° rotation (pitch factor), data acquisition is complete and uninterrupted. This modern technique has greatly improved CT because respiratory artifacts and inconsistencies do not affect the single dataset as markedly as in conventional CT. The single dataset can be used to reconstruct slices of differing thickness or at differing intervals. Even overlapping slices can be reconstructed.
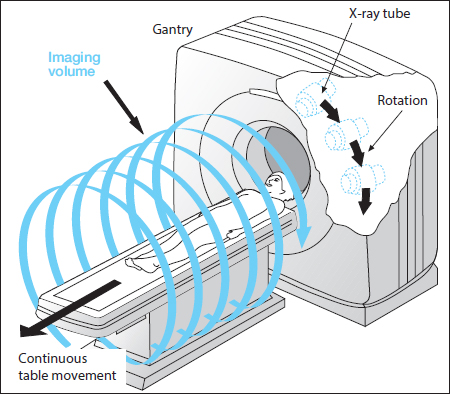
In modern MDCT units with 16 up to 64 detector rows, data acquisition time for e.g. the thorax no longer exceeds the duration most patients can hold their breath: Even with narrow collimations, the entire thorax can be scanned within 7-10 seconds [47]. Even a CTA of the carotid arteries and circle of Willis with a pitch of 1.5 and rotation time of 0.37 seconds/rotation requires only 5 seconds for a scan range of 350 mm (64 x 0.6 mm collimation). Due to increased speed, most modern CT units would pass the renal excretion of CM, so that a longer delay time or a short break are required to document the patient`s renal excretion function. Therefore, the „neck of the bottle“ in the workflow is no longer the data acquisition time, but sometimes the size of the corresponding data files in cases of complex MIP- / MPR-recontructions at the local workstation.
One of the advantages of the helical technique is that lesions smaller than the conventional thickness of a slice can be detected. Small liver metastases (7) will be missed if inconsistent depth of respiration results in them not being included in the section ( Fig. 7.3a ). The metastases would appear in overlapping reconstructions from the dataset of the helical technique ( Fig. 7.3b ).
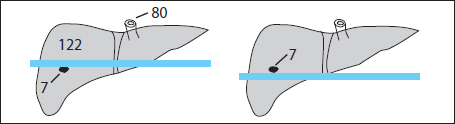
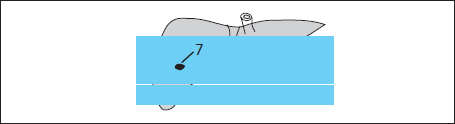
Spatial Resolution
The reconstructed images should have a high temporal resolution to separate even small structures from each other. This generally creates no problem along the x- or y-axis of the image since the selected field of view (FOV) typically encompasses 1024 x 1024 or more picture elements (pixel). These pixels appear on the monitor as grey values proportionate to their attenuation ( Fig. 8.1b ). In reality, however, they are not squares but cubes (voxel = volume element) with their length along the body axis defined by the section thickness ( Fig. 8.1a ).
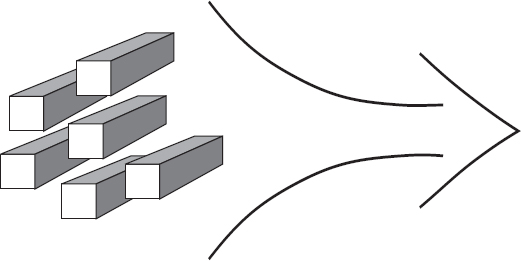
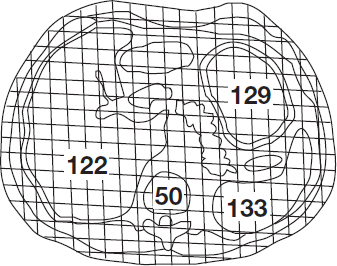
The image quality should improve with smaller voxels, but this only applies to the spatial resolution since a thinner section lowers the signal-to-noise ratio. Another disadvantage of thinner sections is the inevitable increase in the radiation dose to the patient (see page 175). Nonetheless, smaller voxels with identical measurements in all three dimensions (isotropic voxels) offer a crucial advantage: The multiplanar reconstruction (MPR) in coronal, sagittal or other planes displays the reconstructed images free of any step-like contour ( Fig. 8.2 ). Using voxels of unequal dimension (anisotropic voxels) for MPR is burdened by a serrated appearance of the reconstructed images ( Fig. 8.3 ), which, for instance, can make it difficult to exclude a fracture ( Fig. 148.5b ).
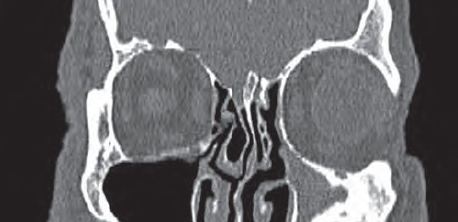
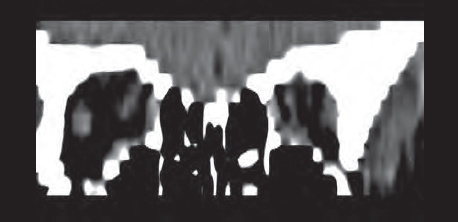
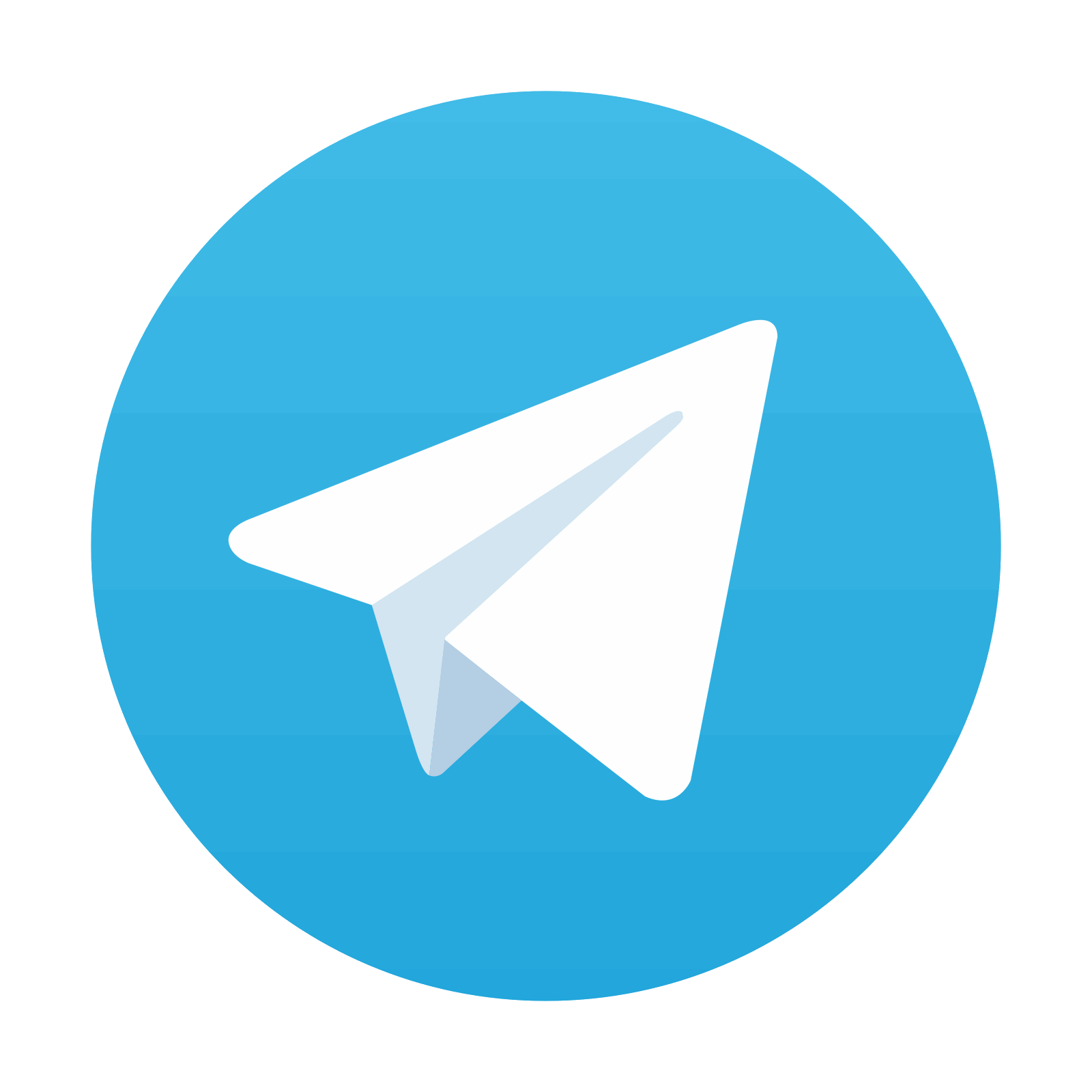
Stay updated, free articles. Join our Telegram channel

Full access? Get Clinical Tree
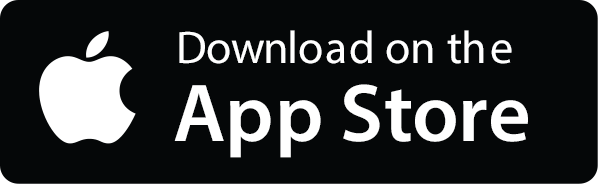
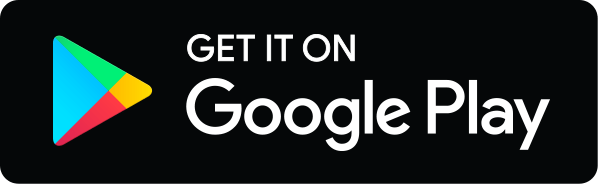