Fig. 7.1
Early brain image from a CT head scanner. Reconstruction performed on a 1602 matrix
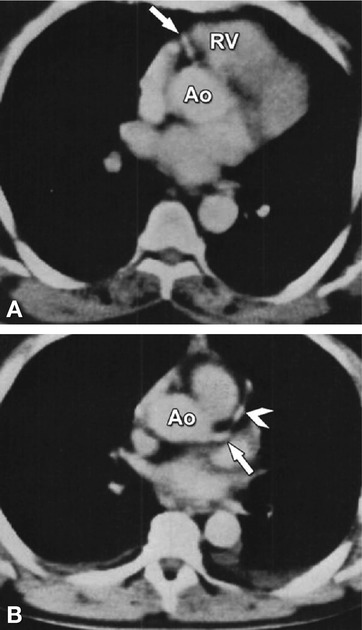
Fig. 7.2
First images of coronary CTA. (Panel a) shows the proximal right coronary artery (arrow) and (Panel b) the left main (arrow) and left anterior descending coronary artery in another patient (arrowhead). Ao aorta, RV right ventricle (With permission from Guthaner et al. AJR 1979 (American Roentgen Ray Society))
It took about 25 years of research and development after Harell’s initial experiment to develop CT scanners suitable for routine cardiac imaging. Good in-plane spatial resolution and good low-contrast resolution were already accomplished with the single detector row CT axial scanners available in 1976. Volume acquisitions became feasible with the introduction of helical single-row CT in 1989, and a small slice thickness of about 1 mm was available as early as 1981. However, it took the introduction of helical 4-row CT scanners with rotation times below 1 s in 1998 to combine small slice thickness and sufficient volume coverage within one breath-hold. With the 4-row CT scanners it became possible for the first time to assess cardiac function and coronary arteries in a clinical setting. Further developments leading to 16- and 64-row CT scanners provided considerable improvements in image quality (Chap. 2). Even more sophisticated performance in cardiac CT is offered by recent achievements, such as second-generation dual-source CT (Somatom Definition Flash, Siemens; Chap. 9b) and volumetric second-generation cone-beam CT (Aquilion ONE, Toshiba; Chap. 9a).
CT images are typically reconstructed using a 512 × 512 axial image matrix. The numerical value assigned to each pixel represents the average linear X-ray attenuation coefficient of the tissues within the associated voxel relative to the attenuation coefficient of water, and are given in Hounsfield units, see Table 7.1. The Hounsfield unit for a voxel that contains different tissues expresses the average attenuation within the voxel; this averaging is referred to as the partial volume effect.
Table 7.1
Attenuation values of different tissues and materials
Substance | Hounsfield unit (HU) | Range of HUs |
---|---|---|
Compact bone | +1,000 | (+300 to +2,500) |
Contrast-enhanced blooda | +400 | (+200 to +600) |
Calcified plaque | +400 | (+130 to +1,000) |
Noncalcified fibrotic plaque | +80 | (+30 to +130 |
Noncalcified lipid plaque | +10 | (−40 to +40) |
Liver | +60 | (+50 to +70) |
Blood | +55 | (+50 to +60) |
Kidneys | +30 | (+20 to +40) |
Muscle | +25 | (+10 to +40) |
Brain, gray matter | +35 | (+30 to +40) |
Brain, white matter | +25 | (+20 to +30) |
Water | 0 | |
Fat | −90 | (−100 to −80) |
Lung | −750 | (−950 to −600) |
Air | −1,000 |
The basic acquisition parameters for a CT scan are the number of active detector rows, slice thickness (mm), X-ray tube rotation time (s), tube current (mA), and tube voltage (kV). In axial scans, the table translation between axial acquisitions is referred to as the table increment, in helical CT the pitch factor defines the table increment per rotation. These parameters must be optimized to achieve contradictory goals such as minimal motion artifacts, coverage within one breath-hold, good spatial resolution, good contrast resolution, and minimal radiation exposure. The parameters relevant for high-quality reconstruction in CT are the reconstruction field of view, the reconstructed slice thickness, the reconstructed slice increment, and the reconstruction filter.
7.2 Physics of Cardiac CT
Users of CT scanners aim at producing images of good diagnostic quality whilst maintaining the radiation exposure of the patient as low as reasonably achievable. In cardiac CT it is crucial to achieve excellent spatial resolution (to be able to visualize the small coronaries), excellent temporal resolution (to avoid motion-induced blurring), and short scan time (to avoid breathing artifacts). In addition, image noise should be low enough and the contrast-to-noise ratio high enough for visualization of the coronary arteries. What distinguishes cardiac CT from most other CT applications is the need for appropriate synchronization of the image reconstruction with the simultaneously recorded ECG.
7.2.1 Spatial Resolution
Spatial resolution plays an important role in coronary CTA, particularly in the visualization of distal segments with diameters down to less than 1 mm. A voxel size of about 0.5 × 0.5 × 0.5 mm or smaller in combination with an intrinsic spatial resolution (expressed as FWHM 1) of about 0.5–0.7 mm is sufficient for coronary imaging. However, for accurate visualization of very small structures, such as early atherosclerotic lesions within the coronary wall, even better spatial resolution is required but not possible with current CT scanners. Spatial resolution in the reconstructed images also depends on the reconstruction filter used; in cardiac CT for example a dedicated stent or coronary reconstruction filter. Small objects such as stents and calcifications may also be visualized inappropriately due to artifacts related to motion, partial volume effect, and beam hardening.
7.2.2 Temporal Resolution
Good temporal resolution is required in cardiac CT to guarantee that the motion of the fast moving coronary arteries does not lead to substantial artifacts. This can be achieved by hardware that allows fast data acquisition, e.g., a fast rotating gantry and/or a gantry equipped with two X-ray tubes. The rotation time of the X-ray tube should be as short as possible, but is limited by engineering since short rotation times lead to very high g-forces, up to 20–30 g, on all components mounted on the rotating gantry. Rotation times of current CT scanners are in the range of 0.27–0.35 s. Optimal temporal resolution can also be enhanced by dedicated reconstruction algorithms. CT images are generally reconstructed from one full (360°) rotation. In cardiac CT, images are reconstructed from half (180°) rotations because, according to the mathematics of CT, this yields the minimum required amount of information. Fig. 7.3 illustrates that hundreds of views are required to reconstruct artifact-free images. Temporal resolution can be further improved by a factor of two with the incorporation of two X-ray tubes (dual-source CT). Improved temporal resolution can also be achieved by including attenuation profiles acquired during two or more heartbeats in the reconstruction. In this latter case, raw data from multiple heartbeats are combined during the reconstruction. This is referred to as a multisegment reconstruction, i.e., more than one RR interval is used for image reconstruction.
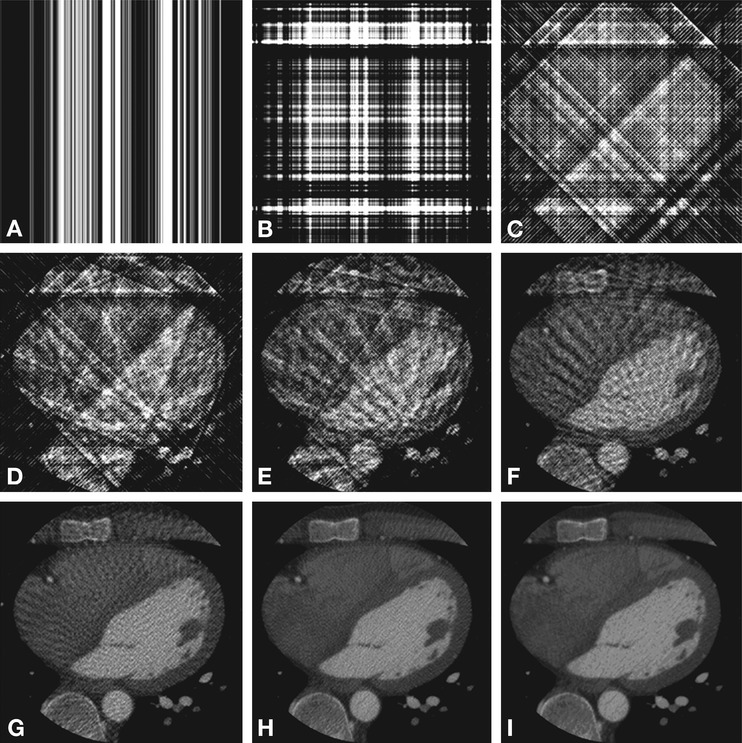
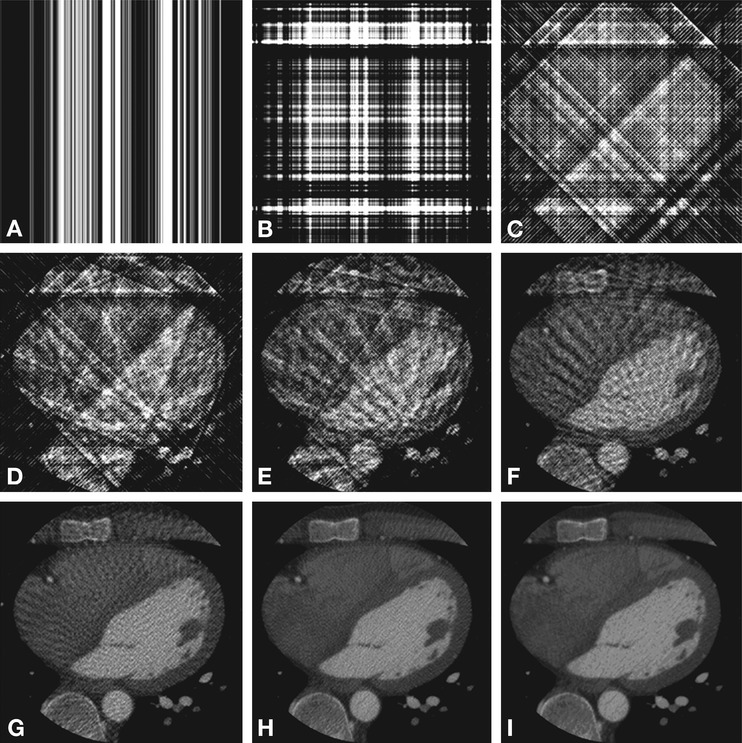
Fig. 7.3
Simulation of the effect of the number of backprojections on image quality. Reconstructed axial cardiac CT images are shown using 1, 2, 4, 8, 16, 32, 64, 128, and 1,024 backprojections in (Panels A–I), respectively
7.2.3 Tube Voltage and Tube Current
Low image noise provides better image quality. Image noise may be decreased by raising the tube current (mA), but this comes at the cost of increased patient exposure. Currently, the choice of tube current in cardiac CT acquisitions is based on clinical experience and provides a balance between low patient dose and sufficient image quality (Chap. 10). Computer simulations of the image quality of cardiac CT resulting from a lower tube current can be performed and may provide an evidence base for selection of the most appropriate tube current (Fig. 8.11). In addition, image quality, and the contrast-to-noise ratio, in cardiac CT may be enhanced by selecting a lower tube voltage (e.g., 100 or 80 kV), which improves the visualization of iodine. Particularly in patients with a normal or low body mass index, a lower tube voltage can be used to achieve better image quality at the same radiation exposure or to reduce the dose whilst maintaining image quality.
7.2.4 Retrospective ECG Gating and Prospective ECG Triggering
Essential in cardiac CT is synchronization of the image reconstruction with the patient’s ECG and selection of the best cardiac phase. Current cardiac CT scanners only provide good image quality, without motion artifacts, when the reconstructed images correspond to the optimal rest phase of the coronary arteries. The principles of retrospective ECG-gated reconstruction and prospective ECG-triggered reconstruction are illustrated in Fig. 7.4.
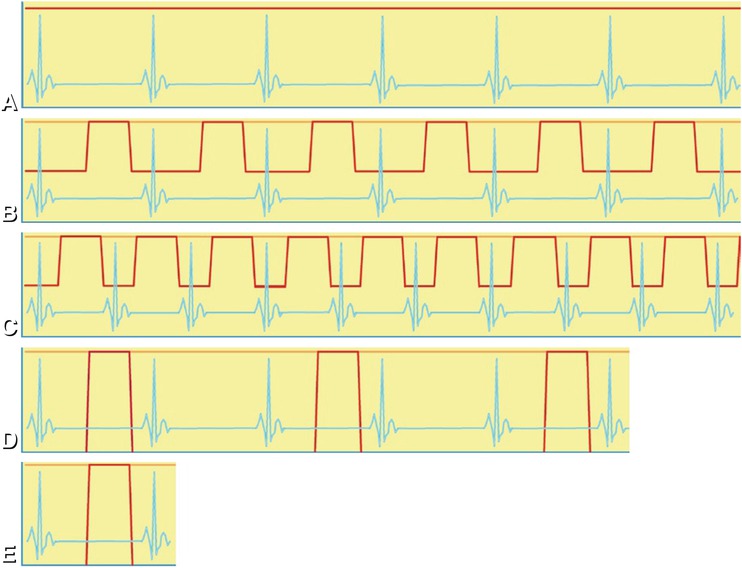
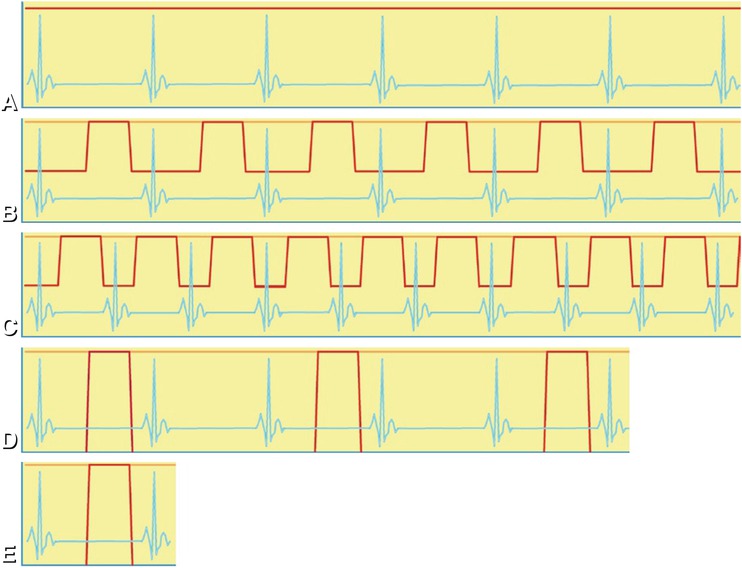
Fig. 7.4
ECG (blue lines) and tube current during different acquisitions (red lines). (Panel A) shows that during a retrospective helical acquisition with a 64-row CT scanner, the tube current remains constant during six RR intervals. (Panel B) shows how the tube current can be modulated during a helical acquisition based on the recorded ECG to achieve good image quality for assessment of the coronary arteries (where the tube current remains high, peaks of the red line) and at the same time sufficient image quality for assessment of cardiac function (where the tube current is reduced). (Panel C) shows that at a higher heart rate, there is less opportunity to reduce tube current during the helical acquisition and less opportunity to reduce patient exposure. All helical acquisitions depicted in (Panels A–C )allow assessment of cardiac function. (Panel D) shows how an acquisition on a 64-row CT scanner is done in five heartbeats with axial scans (“step-and-shoot”) covering the entire heart with lower dose at the assumed coronary rest phase. Different axial scans are separated by at least one heartbeat to allow for translation of the patient. (Panel E) shows that some CT scanners allow prospective scanning of the entire heart within a single heartbeat: a fast dual-source CT scanner is capable of performing a helical acquisition of the entire heart within one heartbeat (Siemens Definition Flash), and a wide cone beam CT scanner performs an axial acquisition of the entire heart (Toshiba Aquilion ONE) within one heartbeat. Such novel “single heartbeat” techniques carry the promise of further dose reduction and image quality improvement
Reconstruction based on retrospective cardiac phase selection requires helical registration of the raw data and the ECG during several complete cardiac cycles. Figure 7.4A shows that retrospective ECG gating allows reconstruction of the scanned volume at any cardiac phase. Effective dose can be reduced using ECG-triggered tube current modulation (Fig. 7.4B). Figure 7.4C shows that at higher heart rates, there is less opportunity to reduce the tube current during helical acquisitions.
An alternative to retrospective helical ECG-gated reconstruction is prospective axial (“step-and-shoot”) acquisition (Fig. 7.4D). Axial scans have the advantage of reducing patient dose, but the required stitching of several volumes is prone to artifacts at the borders of the prospectively acquired slabs, especially in arrhythmic patients. Of course, prospectively acquired axial scans (step-and-shoot) do not allow assessment of cardiac function since they only cover the (assumed) best cardiac phase. Also, prospective ECG triggering is preferred in patients with low and stable heart rates, in whom the center of the acquisition window is located at approximately 70–80% of the RR interval. Some CT scanners allow prospective scanning of the entire heart within a single heartbeat: a fast dual-source CT scanner is capable of performing a helical acquisition of the entire heart (Siemens Definition Flash), and a wide cone beam CT scanner performs an axial acquisition of the entire heart (Toshiba Aquilion ONE) within one heartbeat (Fig. 7.4E). Such novel “single heartbeat” techniques carry the promise of further dose reduction. The appearance of helical and axial scans on film that is exposed on the CT table is shown in Fig. 7.5
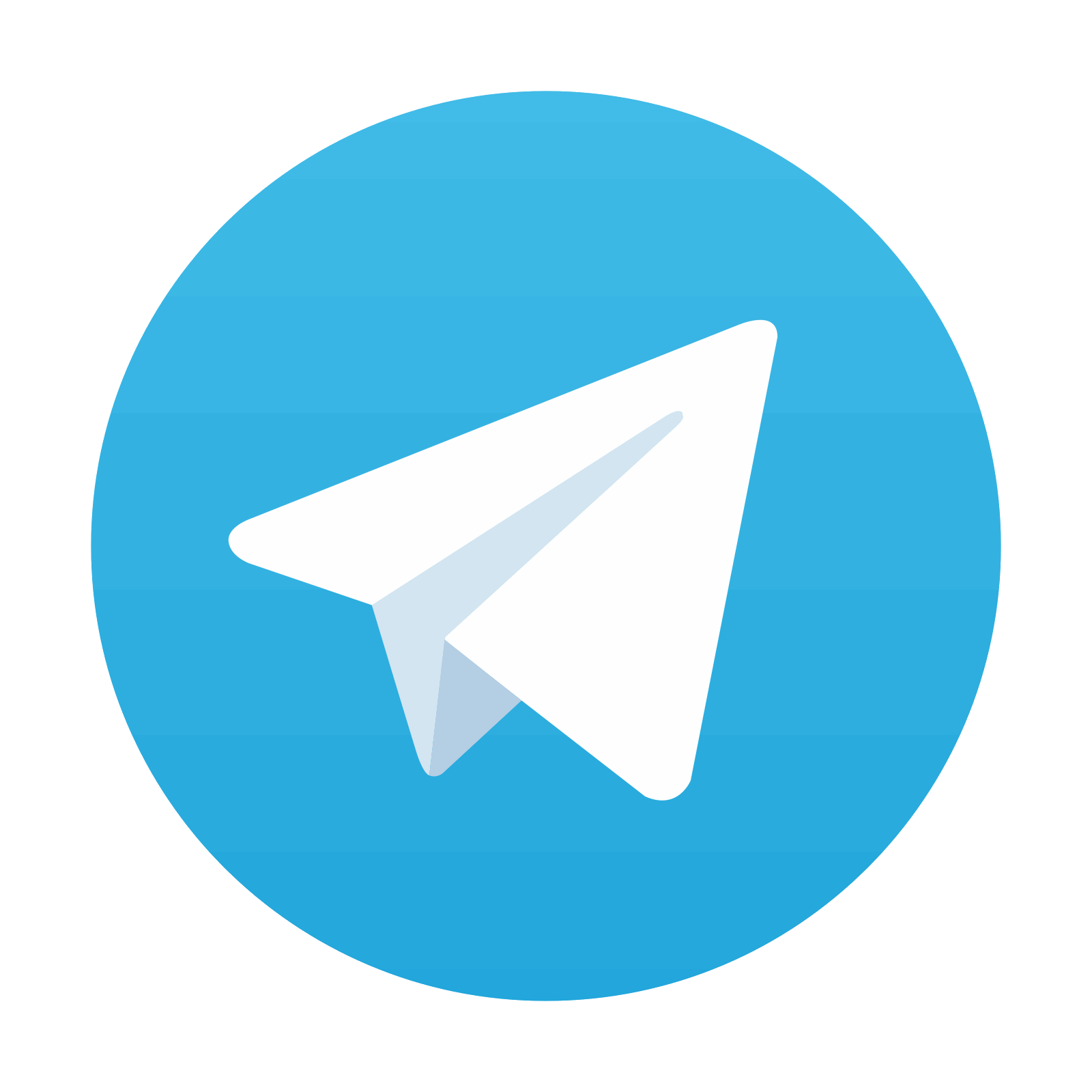
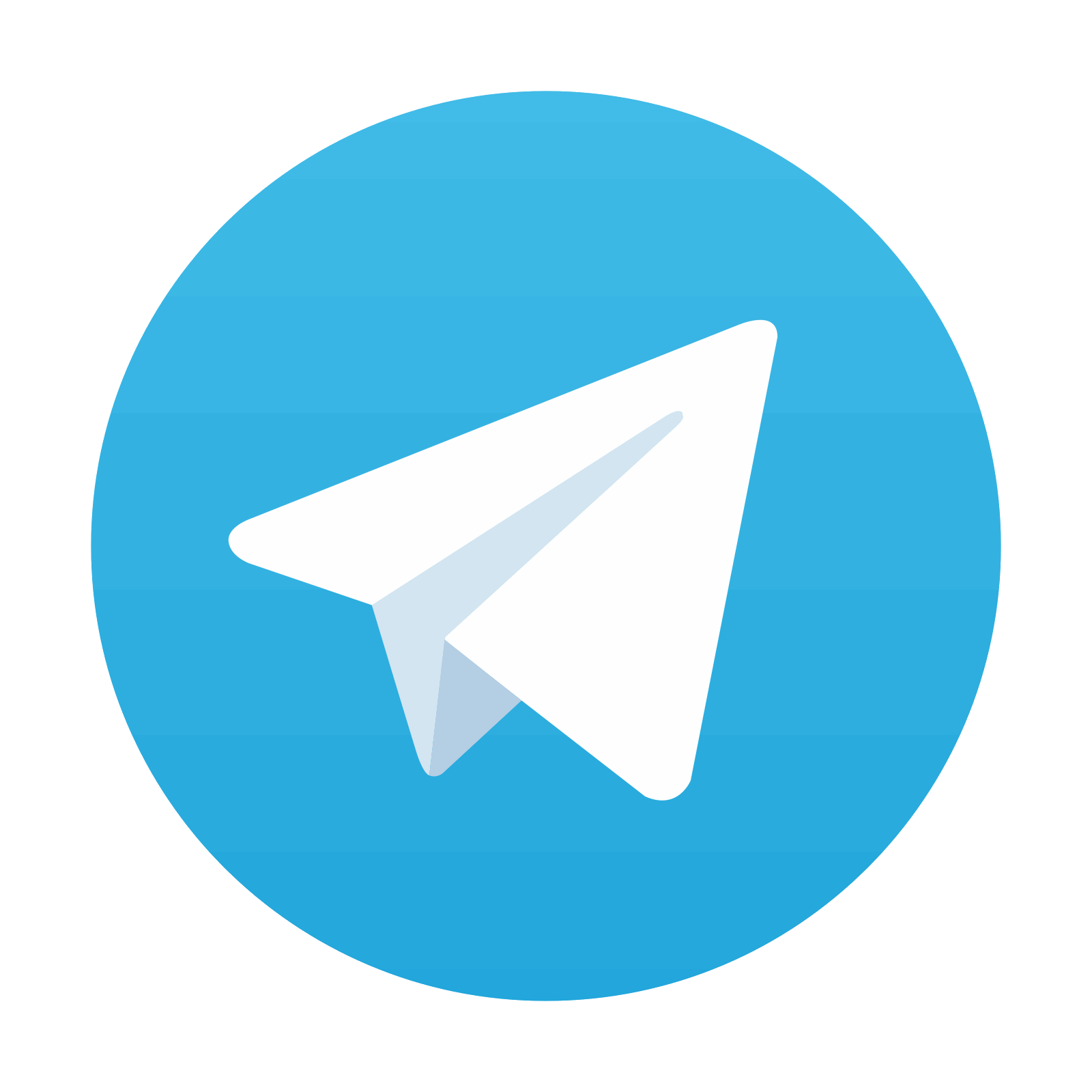
Stay updated, free articles. Join our Telegram channel

Full access? Get Clinical Tree
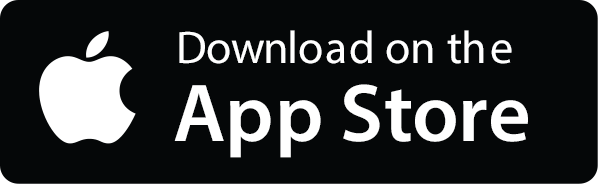
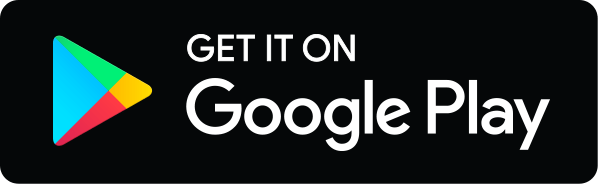