Fig. 17.1
Subpulmonary pleural effusion. Chest radiograph on frontal view (a) shows elevation of the right hemidiaphragm with flattening of its medial aspect. CT scan (b) confirms the presence of pleural effusion in the subpulmonic region
Approximately 75 ml of fluid is required to blunt the posterior costophrenic angle on a lateral chest radiograph. Almost 200 ml of fluid is required to blunt the lateral costophrenic angles on frontal radiographs (Moskowitz et al. 1973). On supine radiographs, it is possible to overlook large amounts of fluid, because the pleural fluid layers posteriorly. A pleural effusion must be suspected when there is increased hazy opacity on the side of the affected hemithorax without obscuration of the vascular markings (Fig. 17.2), when there is blunting of the costophrenic angles or when there is an apical cap. Loculated effusions, defined as effusions that do not shift freely in the pleural space, occur when there are adhesions between the visceral and parietal pleura (Light 1990) and are more frequently seen in exudative effusions, empyema, and hemothorax. When a loculated effusion collects within the interlobar fissures or along the mediastinum, it may simulate a mass (Fig. 17.3). Sonography is a useful tool in determining whether pleural fluid is present and also in guiding aspiration. The majority of pleural collections are identified at sonography as anechoic or hypoechoic collections delineated by the echogenic line of the visceral pleura and lung. Pleural septated effusions and those with echogenic patterns are always exudates, while hypoechoic effusions may be transudates or exudates (O’Moore et al. 1987; Yang et al. 1992). Pleural fluid can be identified on CT scans; however, CT is of limited value in differentiating transudates from exudates. Acute hemorrhage can be identified on the basis of the increased attenuation of the pleural fluid or by the presence of a fluid–fluid level (McLoud and Flower 1991a) (Fig. 17.4).
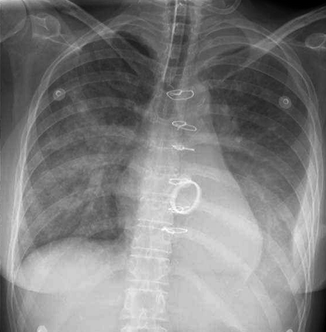
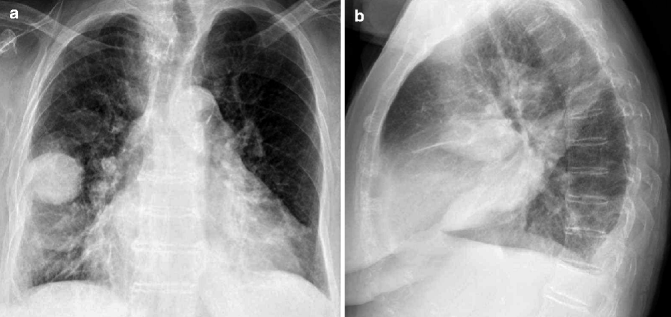
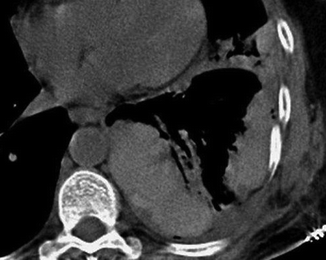
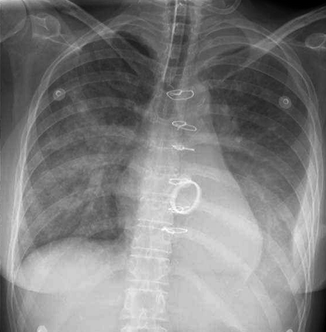
Fig. 17.2
Bilateral pleural effusion. On supine chest radiograph, there is an increased opacity of both lungs without obscuration of the vascular markings, a finding suggestive of bilateral pleural effusion; blunting of the left costophrenic angles is also present
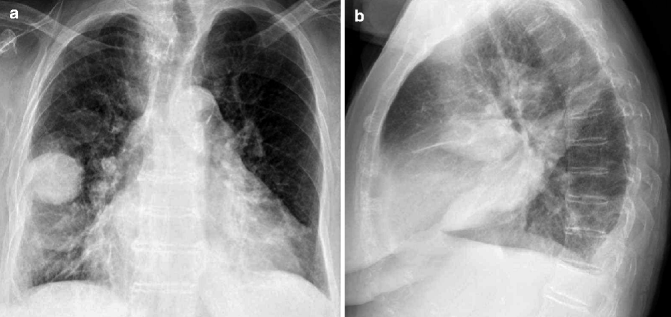
Fig. 17.3
Loculated intrafissural effusion. Chest radiograph on frontal view (a) shows a round well-defined opacity in the right hemithorax at the level of the small fissure simulating a mass. On the lateral view, (b) the opacity has a lenticular shape following interlobar fissures; these findings suggest the diagnosis of loculated intrafissural effusion
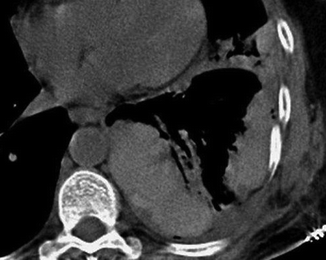
Fig. 17.4
Left acute hemothorax. Unenhanced CT scan through the lower chest depicts a left pleural effusion with increased attenuation representing an acute hemothorax
17.1.2 Empyema
Empyema is inflammatory fluid and debris in the pleural space. It results from an untreated pleural space infection that progresses from free-flowing pleural fluid to a complex collection in the pleural space. The diagnosis is made when the pleural fluid is grossly purulent, when organisms are identified on the basis of Gram stain or culture, or when the fluid has a white blood cell count greater than 5 × 109 cells/l (Light 1990). Radiographic findings are almost always the same as for any pleural collection, but certain CT findings are suggestive of empyema. The typical empyema is lenticular. Nonenhanced CT scans can demonstrate atypical pleural effusions along the mediastinum, thickened pleurae, loculations in the fissures, septa, or gas bubbles in the pleural space. Gas bubbles in the pleural space strongly suggest an empyema in the proper clinical context (i.e., in the absence of recent thoracentesis). Lung windows can demonstrate pneumonia adjacent to the abnormal pleural collection. With most empyemas, CT scans after intravenous injection of contrast medium demonstrate enhancement of the parietal and visceral pleura, the so-called split pleura (Fig. 17.5), thickening of the extrapleural subcostal tissues and increased attenuation of the extrapleural fat (Williford and Godwin 1983). It is necessary to perform a differential diagnosis with an abscess when the collection is peripheral: an empyema generally has a lenticular shape with obtuse margins toward the chest wall, whereas a peripheral lung abscess usually has a spherical shape and forms acute angles with the chest wall. An empyema has thin, smooth walls along its inner margin; lung abscess usually has thick, irregular walls, especially internally. Finally, the adjacent lung is frequently compressed by an empyema, resulting in displacement of peripheral pulmonary vessels and bronchi, while the parenchyma surrounding a lung abscess is often infected, and the pulmonary vessels and bronchi extend directly toward the periphery of the lesion. Empyemas and associated parapneumonic effusions with pH below 7.0 or pleural fluid glucose level less than 40 mg/dl must be treated with a chest tube, inserted surgically or by image guidance with sonography or CT (McLoud and Flower 1991a). The success rates of imaging-guided drainage ranges from 70 to 90 % and are higher than those for surgical tubes guided only by the findings on the chest radiograph (Silverman et al. 1988).
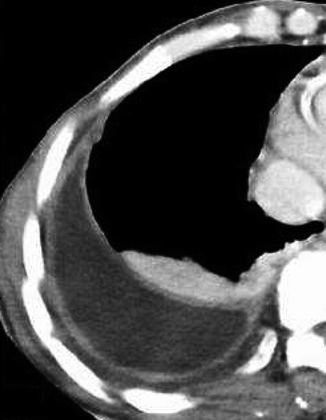
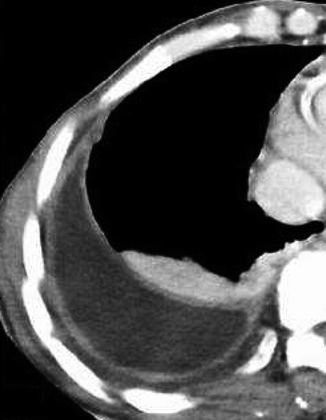
Fig. 17.5
Right pleural empyema. Enhanced CT scan shows a right loculated pleural effusion and a smooth and regular thickening both of the visceral and parietal pleura with enhancement (the so-called “spit pleura sign”); note also the expansion and increased attenuation of the extrapleural fat
17.1.3 Pneumothorax
Pneumothorax is defined as the presence of air or gas in the pleural cavity. Spontaneous pneumothorax is very rare in the elderly population but more frequent in young men; it generally occurs in people without underlying lung disease and in the absence of an inciting event. However, many patients, whose condition is labeled as primary spontaneous pneumothorax, have subclinical lung disease, such as pleural blebs, that can be detected by CT scanning. Pneumothorax secondary to pulmonary pathology is most common in elderly patients with chronic obstructive pulmonary disease, asthma, or chronic infiltrative lung disease (including idiopathic pulmonary fibrosis, sarcoidosis, eosinophilic granuloma, lymphangioleiomyomatosis). Other causes are radiation pneumonitis, silicosis, and tuberous sclerosis.
17.1.3.1 Radiological Features
Free intrapleural air moves preferentially to the less dependent pleural spaces. In the upright patient, free intrapleural air accumulates in an apicolateral location; 50 ml of pleural gas can be visible. The radiographic diagnosis of pneumothorax is based on visualization of the visceral pleura line between the radiolucent lung and radiolucent free air in the pleural space. On supine radiographs, approximately 500 ml of pleural gas is needed to visualize a pneumothorax (Tocino et al. 1985). Anteromedial and subpulmonary pneumothoraces appear to represent the majority of the air collections. In the supine position, air accumulates in front of the lung and surrounds the anterior mediastinal structures: sharp visualization of the superior vena cava (on the right) and of the left subclavian artery (on the left) are initial signs of an anteromedial pneumothorax. Infrahilar anteromedial pneumothorax accounts for a sharp delineation of the heart border and the inferior vena cava and for a deep lucent cardiophrenic sulcus (Ziter and Wescott 1981). In subpulmonary pneumothorax, a hyperlucent upper quadrant of the abdomen with visualization of the superior surface of the diaphragm can be expected; other signs are deep costophrenic sulcus and a sharp hemidiaphragm (Ziter and Wescott 1981) (Fig. 17.6). Occasionally a double-diaphragm sign is present when both the anterior and the posterior surfaces of the diaphragm are surrounded by air. In patients with lung affections in whom the heart or diaphragmatic borders are not visible due to the lack of ventilation of the adjacent parenchyma, one sign of pneumothorax can be the disappearance of the “silhouette sign,” which allows us to visualize the border of the heart or diaphragm once again. The disappearance of the silhouette sign is due to the interposition of the air situated in the pleural space between the affected lung and the mediastinum or the diaphragm. Apicolateral pneumothorax is uncommon in a supine radiograph and suggests that a large volume of pleural air has accumulated and has displaced the visceral pleura medially. Because of the difference in compliance of the lung when affected by parenchymal diseases like pneumonia or adult respiratory distress syndrome, segments of the lung may collapse under pneumothorax, whereas others more severely involved are seen beyond the pneumothorax; therefore, the presence of lung markings beyond a pleural line does not exclude pneumothorax in a supine plain film. When the amount of air accumulated is small, the first sign of apicolateral pneumothorax in the right side is a lack of contact between the minor fissure and the chest wall (Ziter and Wescott 1981). It is important to bear in mind that skinfolds, dressings, tubing, and bags can mimic pneumothorax on some images. Care must be taken when obtaining chest radiographs to minimize these artifacts.
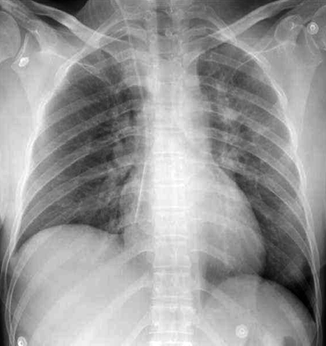
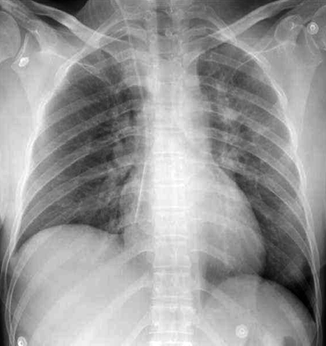
Fig. 17.6
Pneumothorax in the supine position. On frontal chest view, a deep left costophrenic sulcus and a sharp delineation of the hemidiaphragm are clearly seen suggesting the diagnosis of a left pneumothorax
A tension pneumothorax is a life-threatening condition that develops when air is trapped in the pleural cavity under positive pressure (Gilbert and McGrath 1993), displacing mediastinal structures and compromising cardiopulmonary function. Prompt recognition of this condition is life saving. Because tension pneumothorax occurs infrequently and has a potentially devastating outcome, a high index of suspicion and knowledge of basic emergency thoracic decompression procedures are important for all healthcare personnel. Immediate decompression of the thorax is mandatory when tension pneumothorax is suspected. Signs of tension pneumothorax are diaphragm inversion, displacement of the anterior junction line to the contralateral side, or displacement of the azygoesophageal recess. The sign that correlates best with clinical findings of tension pneumothorax is flattening of the heart border and other vascular structures. This sign is due to the impairment to the normal venous return to the heart that occurs in tension pneumothorax (Gilbert and McGrath 1993).
17.1.4 Pleural Plaques
Pleural plaques constitute the most frequent benign pleural pathology in asbestos-exposed patients and are radiologically detectable in 20–60 % of workers exposed to high concentrations of asbestos. A history of asbestos exposure may be demonstrated in over 80 % of subjects with bilateral pleural plaques. Pleural plaques represent circumscribed collections of hyalinized collagen in the submesothelial layer of the parietal pleura of chest wall or diaphragm; they rarely affect mediastinal pleura. Pleural plaques almost always involve the parietal pleura alone, but occasionally they may be seen in the visceral pleura and in the interlobular fissures. They generally appear 15–20 years after the initial exposure. The pathogenesis of asbestos-related pleural plaques is unknown; it is presumed that asbestos fibers penetrate the visceral pleura and irritate the parietal pleura, inciting collagen deposition. The thickness of plaques can vary from 1 mm to more than 10 mm. Plaques are thicker over the ribs than over the intercostal spaces.
17.1.4.1 Radiological Features
On chest radiographs, these lesions appear as focal areas of pleural thickening, usually less than 1 cm thick, and predominantly localized posterolaterally along the inferior costal margins and at the diaphragmatic level. They are usually bilateral and tend to be asymmetrically distributed; when monolateral (25 % of cases), for reasons as yet unknown, they are prevalently left-sided. Pleural plaques have a tendency to calcify. Forty years after the initial exposure to asbestos, almost 40 % of these people exhibit radiologically obvious pleural calcifications. They may appear punctate, linear, or occasionally “cakelike,” especially when located along the diaphragm surfaces. Less commonly calcified plaques may be pedunculated, in which case they may be mistaken for intraparenchymal nodules (Muller 1993). CT facilitates the successful detection and location of pleural plaques because the cross-sectional images are free from overlapping (Fig. 17.7). It can also define subpleural fat deposits that mimic plaques on plain radiographs. Radiological findings that are suggestive of extrapleural fat rather than pleural plaques include a bilateral location among the midlateral chest wall and a symmetric distribution. Some authors have shown that the combination of bilateral abnormalities and posterolateral plaques at least 5 mm thick or bilateral calcified diaphragmatic plaques has a 100 % positive predictive value for the diagnosis of asbestos-related disease. Although pleural plaques per se are asymptomatic and not premalignant, detection of pleural plaques is important for three main reasons: (a) in patients with associated interstitial disease, the presence of these lesions, in the appropriate clinical setting, strongly suggests the diagnosis of asbestosis; (b) they are virtually pathognomonic of asbestos exposure and thus are important diagnostic clues suggesting an increased risk of developing malignant pleural mesothelioma; and (c) they may serve as encouragement to stop smoking because of the synergistic interaction between asbestos exposure and smoking in the development of lung cancer (Muller 1993).
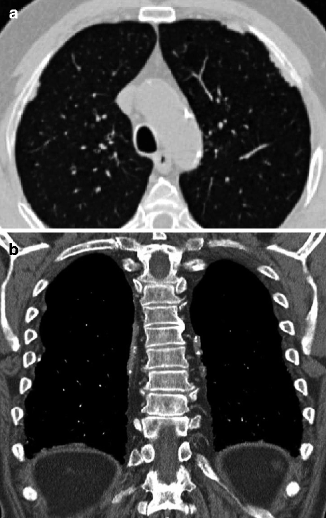
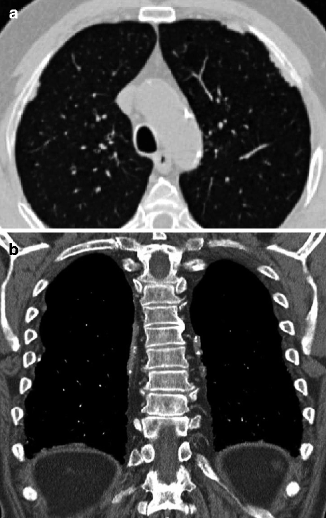
Fig. 17.7
Pleural plaques in a patient with history of asbestos exposure. Axial CT scan (a) shows focal areas of pleural thickening with bilateral and asymmetric distribution along the costal pleural surfaces. On coronal reformatted image, (b) the plaques appear partially calcified and are also seen along the diaphragmatic pleura
17.1.5 Fibrothorax
Fibrothorax, almost always due to previous empyema, is easily detected as a thick, calcified pleural rind on both plain radiographs. Calcified fibrothorax in older patients is particularly likely to be tuberculous in origin. The list of possibilities can be narrowed down given a history of therapy for tuberculosis, although such a history is frequently lacking (Hulnick et al. 1983). Rarely, tuberculous pleurisy progresses to become chronic tuberculous empyema, which may be defined as persistent, grossly purulent pleural fluid containing numerous tubercle bacilli. Since the advent of chemotherapy, this is usually seen secondary to incomplete medical treatment, which sometimes leads to the development of resistant organisms (Gallardo et al. 2000). Tuberculous empyema is often asymptomatic and sometimes does not become apparent until many years after the episode of acute pleurisy. During this period, tubercle bacilli remain dormant within the calcified pleural space and are later reactivated. CT, which differentiates fluids from soft tissues and calcifications, can identify collections of pleural fluid within a calcified fibrothorax. These findings are the most accurate indicator of active infection. In addition, CT can be of value in diagnosing complications that may arise from chronic tuberculous empyema, specifically bronchopleural fistula (BPF) and empyema necessitatis (EN). Although BPF is decreasing in frequency, it is still a serious complication of pulmonary resection and inflammatory diseases of the lungs. Tuberculous residual derangement in pulmonary or pleural architecture, spontaneous or iatrogenic, predisposes to the development of BPF. Fibrothorax appears to be the most common source of late BPF formation, while, during the active disease, rupture of a pulmonary cavity in the pleural space is more common. BPF may present clinically in various forms, ranging from incidental radiographic discovery to life-threatening acute pneumothorax. Radiographically, BPF usually presents as a new pleural air-fluid level, and sometimes with a tension pneumothorax. Diagnosis is often made by the correlation of sequential changes in chest radiographs. The presence or absence of an active infection is crucial in selecting appropriate medical treatment. The introduction of a drainage tube alone can lead to closure of the fistula. If the fistula does not close with tube drainage in a few weeks, a definitive operation is recommended (Hulnick et al. 1983). EN is an uncommon complication of an empyema in which the inflammatory mass spontaneously bores its way from the pleural cavity into the soft tissues of the thoracic wall, forming a subcutaneous abscess, which may or may not open to the skin. The most common etiology is tuberculosis. The most common clinical presentation is a mass in the thoracic wall that is sometimes accompanied by a cutaneous fistula. The chest radiograph usually shows signs of chronic pleural disease with pleural thickening, which may be calcified. A subcutaneous mass associated with pleural empyema should be considered suspicious for EN. CT is very useful and is diagnostic when it shows a pleural collection with thickened often calcified pleural wall, associated with a mass in the thoracic wall (Fig. 17.8).
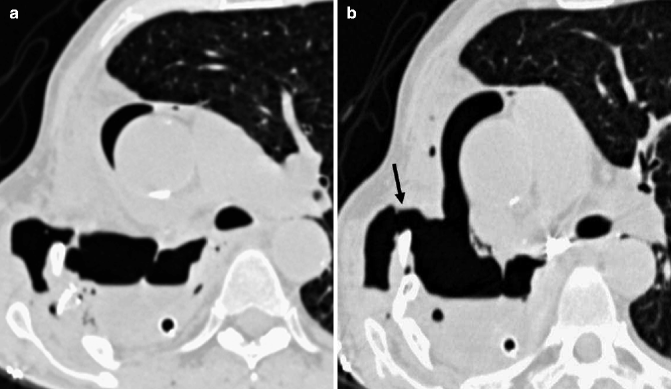
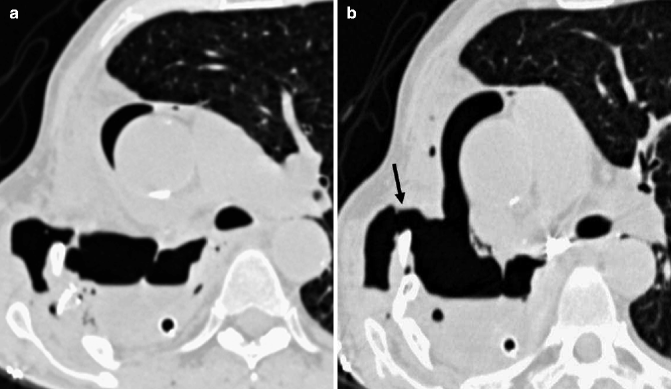
Fig. 17.8
Empyema necessitatis. Axial CT images (a, b) demonstrate a multiloculated collection in the right pleural cavity with a communication between the empyema and the chest wall and a subcutaneous air-fluid level (arrow in b)
17.2 Malignant Pleural Disease
Malignant pleural disease is a common clinical problem, as about 25 % of all pleural effusions in elderly patients have a neoplastic origin Feragalli B et al. 2003).
The pleura may be involved by one of several primary or secondary malignant tumors. Primary tumors account for about 10 % of pleural malignancies and are malignant pleural mesothelioma, localized fibrous tumor, and pleural sarcoma. Secondary tumors account for about 90 % of pleural neoplasms; the principal causes are adenocarcinoma or, less frequently, lymphoma and thymoma.
Depending on the location, size, and underlying histologic features, pleural tumors may produce a spectrum of findings; CT is the best method for characterizing location and composition of pleural masses and is particularly useful in distinguishing pleural from peripheral pulmonary lesions.
17.2.1 Primary Tumors
17.2.1.1 Malignant Pleural Mesothelioma
Malignant pleural mesothelioma (MPM) is the most common primary neoplasm of the pleura that typically affects individuals occupationally exposed to asbestos through a variety of industries.
Epidemiology and Etiology
MPM is an important health problem both because its incidence has increased over the past 30 years and because of the diagnostic, clinical, and therapeutic challenges it poses (Damhuis and Planteydt 1995; Peto et al. 1995; Aberle and Barnes 1991). Approximately 2,000–3,000 cases of MPM occur in the United States each year (Aberle and Barnes 1991), and the incidence is expected to rise till approximately 2020 (Peto et al. 1995). Men are more frequently affected, and the male to female ratio is 2–6:1. The peak of incidence occurs between the sixth and seventh decade of life (Pisani et al. 1988); MPM is extremely rare in childhood (Fraire et al. 1988; Kane et al. 1990).
The association between MPM and asbestos fibers was established in 1960 by Wagner et al. (1960) in a landmark study which identified a clear relationship between occupational exposure to crocidolite and the development of mesothelioma. Thereafter, this association was confirmed by numerous studies (Antman 1980; Churg 1988; Craighead et al. 1982; Mossman and Churg 1998; Roggli et al. 1992) that demonstrated a high incidence of MPM among patients with prolonged asbestos exposure and a beginning was made to abandon the production and processing of asbestos material. Approximately 80 % of MPM cases occur in individuals occupationally exposed to asbestos (McDonald and McDonald 1980; Hammar 1994a, b); the lifetime risk of developing mesothelioma for asbestos workers has been estimated at about 10 % (Selikoff et al. 1980). The incidence of MPM is higher in subjects with continuous exposure compared to occasional exposure; nonetheless, even brief (under 1 year) or indirect exposures (such as contact with the clothing of an exposed worker) may produce the tumor. The highest incidences are found in shipyard and construction workers, in asbestos miners and manufacturers, and in heating trade and insulation workers (McDonald and McDonald 1980; Huncharek 1992).
The latency period for development of MPM is about 35–40 years after initial exposure; the incidence peak occurs later than asbestos-related lung cancer (25–30 years) and earlier than asbestosis (40–45 years). Unlike asbestosis and lung cancer, the incidence of MPM does not decrease after reaching its peak but remains steady.
Asbestos minerals can be divided in the serpentines (chrysotile, the so-called “white asbestos,” and anthophyllite) and the amphiboles (crocidolite, the so-called “blue asbestos”; amosite, the so-called “brown asbestos”; and tremolite). The carcinogenicity appears to be related to the length-to-diameter ratio of asbestos fibers; fibers with the highest ratio being the most carcinogenic. In general, the amphiboles have the highest ratio, and epidemiologic data confirms their malignant potential (Mossman and Churg 1998; Rudd 1996). The amphiboles appear to be 15 times more potent than chrysotile in the development of mesothelioma (Miller et al. 1996). The microscopic marker of heavy prior asbestos exposure is the presence in the sputum of so-called “asbestos bodies,” a type of ferruginous bodies resulting from the deposition of iron–protein complexes on asbestos fiber cores. Macroscopic markers of asbestos exposure are pleural plaques (Greenberg 1992; Hillerdal 1994). As we described before, pleural plaques radiologically appear as focal areas of pleural thickening, typically less than 1 cm thick, that affect the parietal pleura; the visceral pleura is rarely affected. The plaques first appear 15–20 years after the initial asbestos exposure. There is no evidence that these lesions are premalignant or precursors of MPM; the presence of plaques, however, does suggest an increased probability of MPM occurring (Hillerdal 1994).
About 20 % of patients with mesothelioma have no history of asbestos exposure nor evidence of asbestos fibers in their lungs, and, consequently, other etiologic factors have been implicated including exposure to other mineral fibers (zeolite), chronic inflammation, genetic factors, radiation exposure, viruses (Simian virus 40), and exposure to other nonfibrous minerals and organic chemicals (polyurethane, ethylene oxide, polysilicone) (Hammar et al. 1989; Carbone et al. 1997; Peterson et al. 1984; Sahin et al. 1993; Hillerdal and Berg 1985; Baas et al. 1998).
Clinical Features
Most patients present relatively late with symptoms since the onset is usually insidious. The most frequently reported complaints are localized chest pain (70 %), as a result of growth in the chest wall, and dyspnea (68 %) due to the presence of a pleural effusion. Weight loss, coughing, and fever are also present in a large proportion of patients. Secondary hypertrophic osteoarthropathy occurs in less than 10 % of patients (Antman 1980). Laboratory analysis may reveal thrombocytosis (more than 400,000 platelets/mm3) which occurs in 34–41 % of patients (Ruffie 1992). Intermittent hypoglycemia is found in less than 10 % of patients.
Pathological Features
MPM manifests most commonly as multiple tumor masses that involve the parietal and visceral pleural surfaces with greater involvement of the parietal than visceral layer. The tumor may progress to thick sheet like or confluent masses, with resultant lung encasement. Greater involvement of the inferior hemithorax is probably due to gravitational factors. A pleural effusion, whether exudative, bloodstained, or frankly hemorrhagic, is present in about 60 % of patients at diagnosis. Chest wall and mediastinal involvement, including pericardial and cardiac invasion, may occur in advanced disease. Tumor growth along needle tracks and scars that penetrate the chest wall have been documented. The diaphragm is frequently infiltrated; tumor extension into the peritoneal cavity is identified in at least one-third of autopsies. Lymphatic and hematogenous metastases occur more frequently than previously supposed (Huncharek 1994; Huncharek and Smith 1988) and are found at autopsy in more than half of MPM cases (Huncharek 1994).
Microscopically, MPM is generally divided into three histologic categories: epithelioid, sarcomatoid, and biphasic. Epithelioid mesothelioma constitutes approximately 55–65 % of malignant mesotheliomas; it may be difficult to distinguish from peripheral adenocarcinoma of the lung with pleural invasion or adenocarcinoma metastatic to the pleura. Techniques other than hematoxylin–eosin staining are needed to make the correct diagnosis, including histochemical staining, immunohistochemical staining, and electron microscopy (Miller et al. 1996; Wick et al. 1990). The sarcomatoid variant constitutes approximately 10–15 % of MPM and must be differentiated from a true sarcoma such as osteosarcoma or chondrosarcoma. The remaining mesotheliomas (20–35 %) fall into the biphasic category, which evinces features of both epithelioid and sarcomatoid mesotheliomas.
Radiographic Features
The most indicative plain radiographic findings of MPM are irregular, nodular opacities around the periphery of the lung, associated with ipsilateral pleural effusion (30–95 %) (Wechsler et al. 1983). However, it should be remarked that pleural thickening, especially when focal and circumscribed, cannot be identified on chest radiograph and, consequently, a monolateral pleural effusion can often be the only radiographic sign of an MPM. Unlike pleural effusion unrelated to pleural malignancies, pleural effusion in MPM is characteristically not associated with contralateral shift of the mediastinum because of the restrictive action of the pleural tumor peel. Instead there may be evidence of volume loss on the affected side, with narrowing of the intercostal spaces, elevation of the hemidiaphragm, and ipsilateral shift of the mediastinum (Fig. 17.9). Alternatively the mediastinum may be fixed in the midline. Distinct pleural masses without effusion are identified in less than 15 % of patients on their initial chest radiograph. It should be recalled, however, that radiography does not always allow easy and precise definition of the pleural or parenchymal localization, just as pleural thickening may be erroneously diagnosed even in the absence of this disease. False positives may be due to thickening of the subpleural fat or to prominence of intercostal muscles; symmetric radiographic findings may sometimes suggest the correct diagnosis and exclude pleural disease. In conclusion, plain chest radiography has low specificity and sensitivity in diagnosing pleural diseases, particularly MPM; recidivant monolateral pleural effusion, especially if associated with ipsilateral volume loss, should raise the suspicion of MPM, thus indicating that CT is needed.
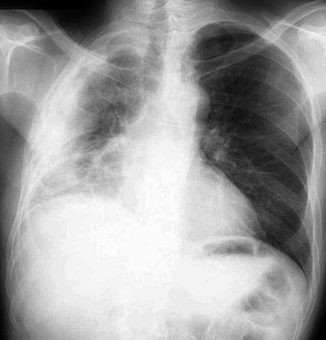
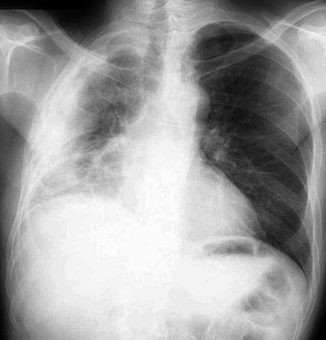
Fig. 17.9
Right MPM in a 55-year-old woman with no history of asbestos exposure. PA chest radiograph shows right pleural effusion and irregular pleural thickening with extension to the major fissure, encasing the lung and contracting the right hemithorax
CT Features
CT has been shown to be superior to chest radiography in identifying and characterizing pleural disease. The tumor often shows a widespread nodular thickening surrounding the lung, spreading into the fissures and extending into the mediastinum (Fig. 17.10). In a study of 50 patients, Kawashima and Libshitz (1990) reported the most frequent CT findings of MPM: diffuse or nodular pleural thickening (92 % of patients), thickening of the interlobular fissures (86 %), pleural effusion (74 %), loss of volume of the involved hemithorax (42 %), pleural calcifications (20 %), and chest wall invasion (18 %). In a more recent study, other authors (Seely et al. 2009) reviewed CT findings of 92 patients with biopsy-proved MPM. They found that all the patients had pleural thickening that was nodular in 79 patients (86 %) and mediastinal in 87 (95 %); ipsilateral volume loss was seen in 42 patients (46 %) and pleural effusion in 80 patients (87 %).
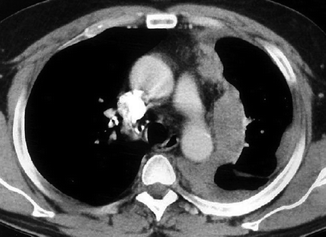
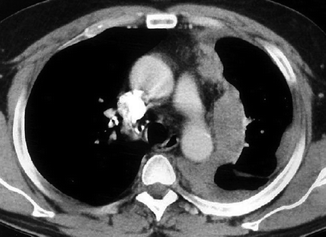
Fig. 17.10
Left MPM in a 59-year-old man with history of asbestos exposure. Enhanced CT scan through the upper chest shows circumferential, irregular, and left-sided pleural thickening with involvement of the fissure. Mediastinal pleura appears particularly thickened at this level where the neoplastic tissue infiltrates the mediastinal fat without any sign of blood vessel involvement
Detection of the above signs raises the problem of differentiating MPM from other benign or malignant pleural diseases also manifesting with pleural effusion and thickening, including fibrothorax, asbestos-related pleural fibrosis, empyema, infections (tuberculosis, fungal, actinomycosis), and pleural metastasis. Leung et al. (1990) have shown that, despite this overlap, CT can play a major role in distinguishing malignant from benign pleural disease in consideration of the following high specific signs: (a) circumferential pleural thickening (specificity 100 %, sensitivity 41 %), (b) nodular pleural thickening (specificity 94 %, sensitivity 51 %), (c) parietal pleura thickening of more than 1 cm (specificity 94 %, sensitivity 36 %), and (d) mediastinal pleural involvement (specificity 88 %, sensitivity 56 %). Twenty-eight of 39 malignant cases (sensitivity 72 % and specificity 83 %) were identified correctly on the basis of the presence of one or more of these criteria. These features may be seen in mesothelioma and in metastatic pleural disease but are unusual in benign pleural disease. There is a close correlation between these signs and pathology. Whereas MPM tends to involve the entire pleural surface, reactive pleurisy usually does not affect the mediastinal pleura (except in case of tuberculous empyema which, when extensive, may involve the mediastinal pleura); for this reason, it is important to remember that mediastinal pleural thickening and circumferential pleural thickening, also when minimal and focal, should raise suspicion of MPM especially when associated with pleural effusion and/or ipsilateral volume loss (Figs. 17.11 and 17.12). More recently, other authors (Hierholzer et al. 2000) described signs indicative of malignant pleural disease, finding similar results to those reported above, except for pleural thickening more than 1 cm that was seen in both malignant and benign pleural disease with no statistically significant differences.
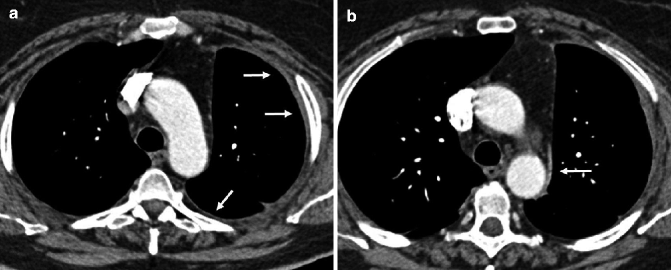
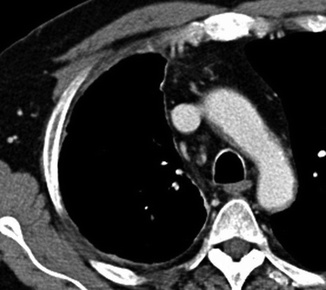
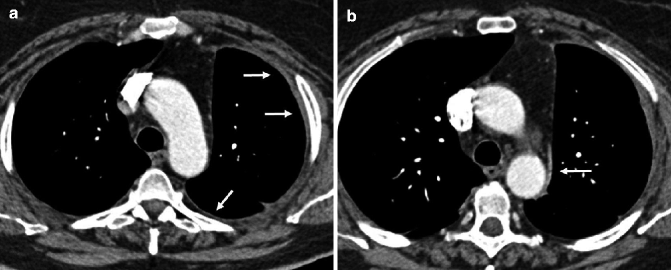
Fig. 17.11
Fifty-five-year-old woman with early MPM. CT scans show a minimal thickening of the left parietal pleura (arrows in a) associated with a minimal and focal thickening of the ipsilateral mediastinal pleura (arrow in b) and a mild volume loss of the hemithorax. Even when it is smooth and regular, as in this case, the involvement of mediastinal pleura is suspect for MPM
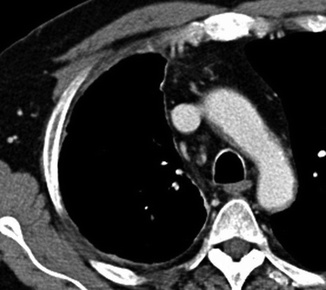
Fig. 17.12
Sixty-seven-year-old man with early MPM. Axial enhanced CT scan displays minimal pleural thickening with circumferential appearance, suggestive of MPM
The presence of pleural calcifications suggests a benign process. In the series by Lung, calcifications were seen in 16 of 35 patients with benign pleural thickening and in only 3 of 39 patients with malignant pleural disease. Rib destruction and frank chest wall invasion are good indicators of malignancy; however, some infectious process, such as actinomycosis, tuberculosis, and nocardiosis, very rarely can invade the chest wall, but usually at a single focus rather than at multiple sites, as seen with malignant disease (Miller et al. 1996).
To conclude, CT is highly sensitive in identifying pleural disease. Most of the time, it allows differentiation between benign and malignant pleural lesions, whereas it does not always enable differentiation between MPM and pleural metastasis when these involve only one hemithorax.
MRI Findings
MPM has intermediate signal intensity on T1-weighted images, similar to that of chest wall muscles. On T2-weighted images, MPM has increased signal intensity relative to that of the adjacent chest wall musculature with focal areas of very high signal intensity due to pleural fluid (Hierholzer et al. 2000; McLoud and Flower 1991b; Montalvo et al. 1991) (Fig. 17.13). Like CT, MRI scans allow identification of pleural thickening, involvement of the interlobar fissures, and the craniocaudal extension of the tumor. The signal intensity of pleural lesions has also been analyzed by Falaschi et al. (1996) who assessed the capability of MRI to differentiate malignant from benign pleural lesions. The authors found that high signal intensity on T2-weighted images was observed in all malignant lesions, whereas only two benign lesions in their series displayed this signal. The authors concluded that high signal intensity on T2-weighted images had a good diagnostic accuracy in differentiating malignant from benign pleural lesions, showing a sensitivity of 100 % and a specificity of 87 %. The negative predictive value was 100 % which implies that the presence of low signal intensity in sequences with long repetition time (TR) is a reliable sign of benignity and is related to the fibrosis characteristic of benign disease. In their more recent study, Hierholze and colleague (Hierholzer et al. 2000) confirmed that high signal intensity in relation to intercostal muscles on T2-weighted and/or contrast-enhanced T1-weighted images is significantly suggestive for malignant disease. Using morphologic features in combination with the signal intensity features, MRI had a sensitivity of 100 % and a specificity of 93 % in the detection of pleural malignancy. It can therefore be inferred that, in identifying and characterizing pleural lesions, MRI may be used as an adjunct to CT in the presence of doubtful findings.
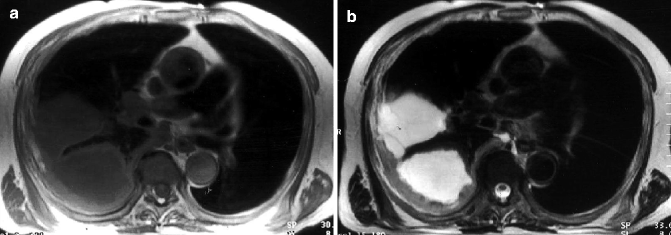
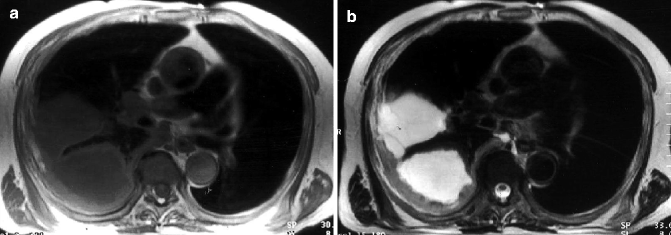
Fig. 17.13
MPM of the right lung in a 68-year-old man. Axial T1-weighted MRI (a) demonstrates irregular pleural thickening of moderate signal intensity and loculated pleural effusions of lower signal intensity. Axial T2-weighted image at a similar level (b) allows differentiation between the tumor, which has increased signal intensity relative to that of the chest wall musculature, and the pleural effusion, which has very high signal intensity
Diagnostic Thoracoscopy
Diagnostic thoracoscopy is indicated in any patients without a precise histopathological diagnosis in whom clinical and laboratory findings raise the suspicion of MPM. In a study of 188 patients with MPM, Boutin et al. (Boutin and Rey 1993; Boutin et al. 1993) compared the sensitivity of the diagnostic techniques currently used in the diagnosis of MPM. They reported the following sensitivity rates: (a) pleural fluid cytology, 26 %; (b) percutaneous needle biopsy, 20.7 %; (c) pleural fluid cytology and needle biopsy, 38.7 %; and (d) thoracoscopic biopsy, 98.4 %. Medical or surgical thoracoscopy has therefore become the gold standard for the cytological–histological diagnosis of MPM, and exploratory thoracotomies, burdened by higher mortality and morbidity rates, have been almost completely abandoned.
Prognosis and Staging
MPM is a usually fatal primary neoplasm. In fact once malignant mesothelioma is diagnosed, the typical course is rapid progression to death, usually of local disease: the mean survival time after diagnosis is approximately 11 months in patients treated with conventional therapies. Some studies have demonstrated that survival was improved in patients who had extrapleural pneumonectomy followed by radiation and chemotherapy (Sugarbaker et al. 1991, 1999; Pass 1994
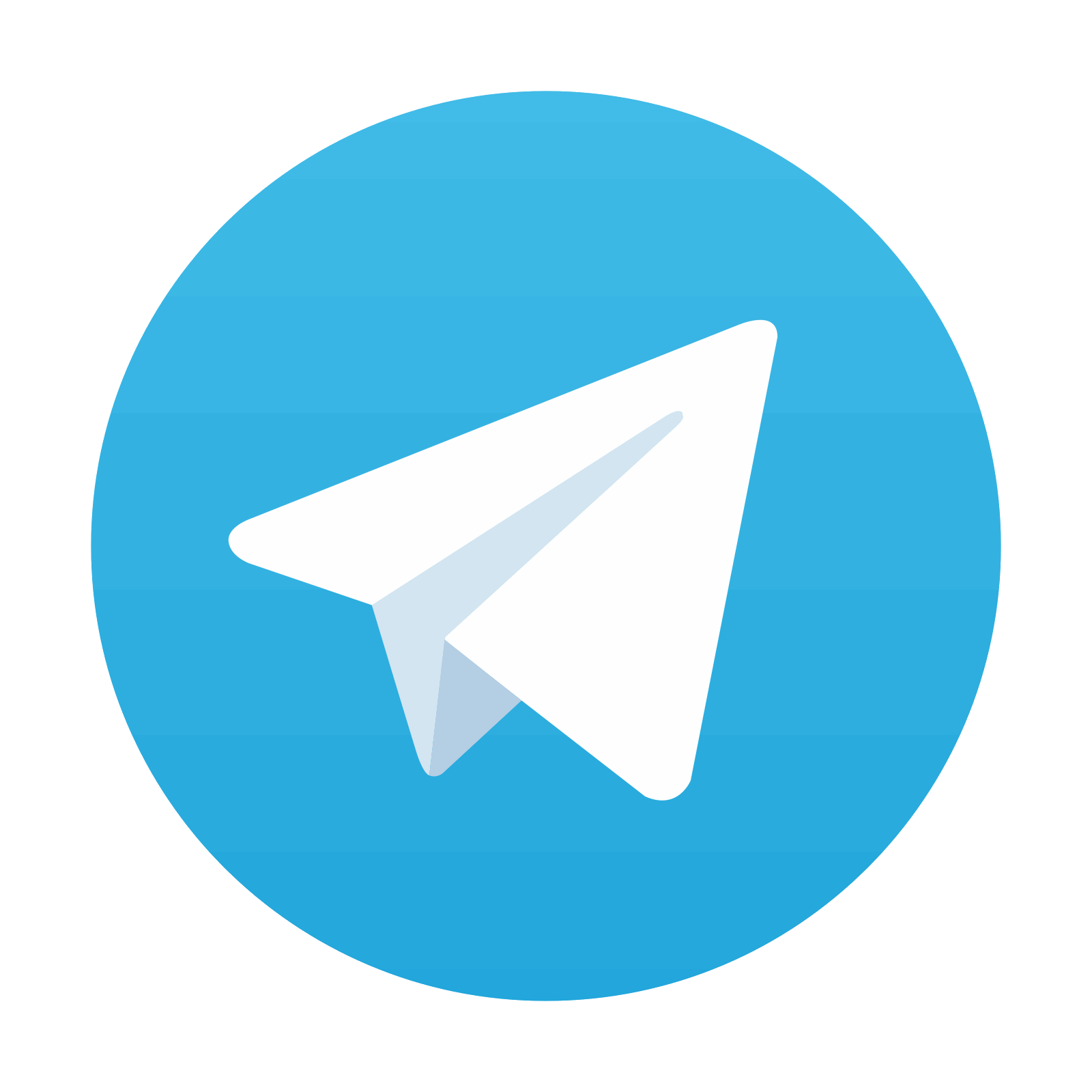
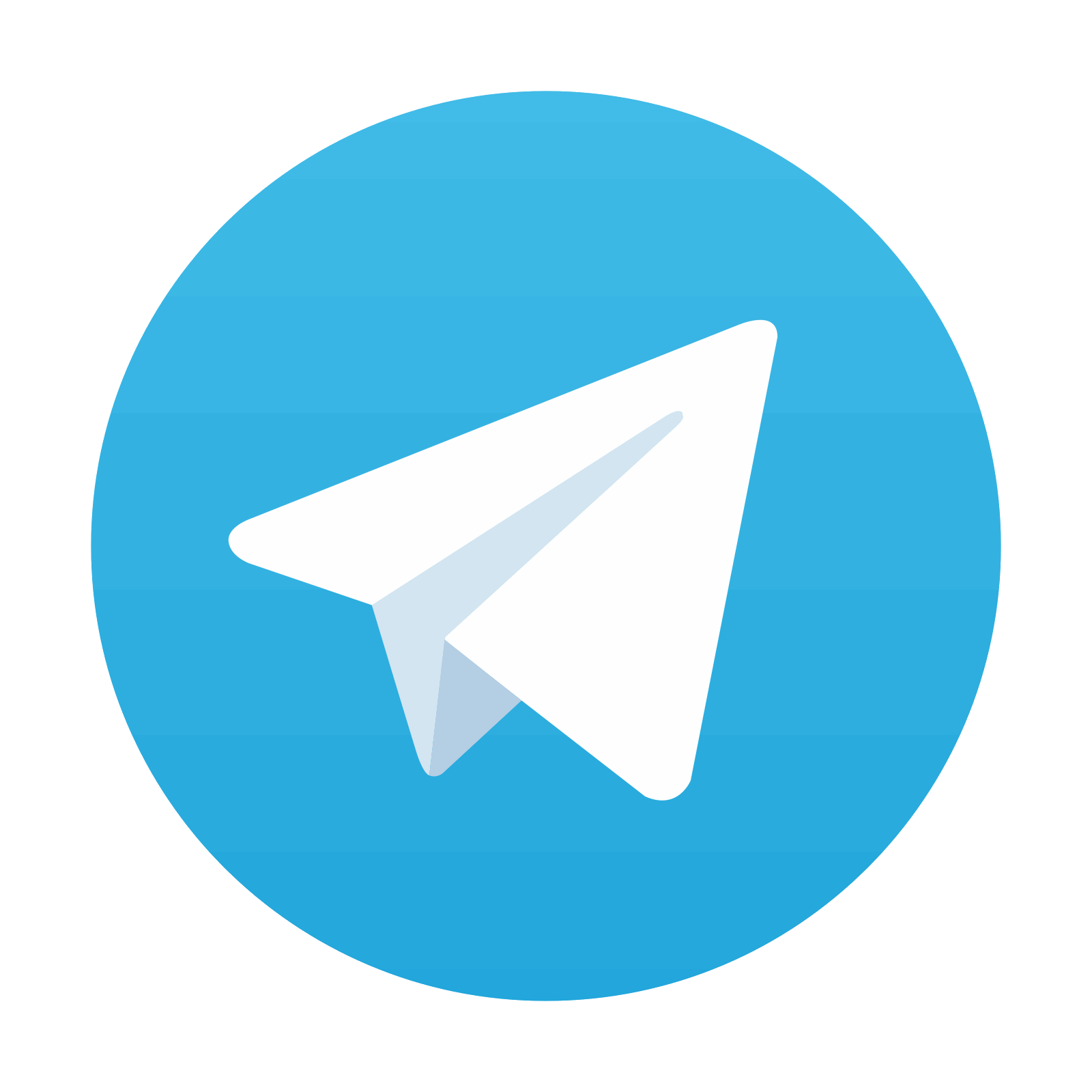
Stay updated, free articles. Join our Telegram channel

Full access? Get Clinical Tree
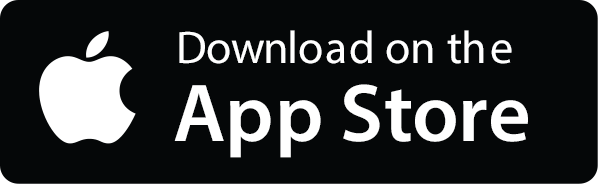
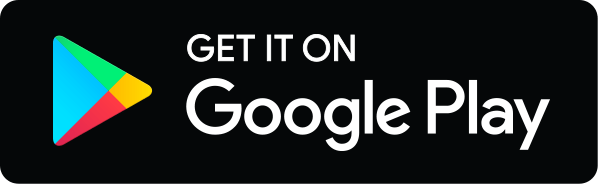
