Prostate
Anthony L. Zietman
William U. Shipley
Hiroshi Tsuji
Prostate cancer, along with breast and lung cancer, constitute a significant proportion of the cases seen by the practicing radiation oncologist. Up to 40% of the referrals in some US practices are patients with this disease. In part, this is because of the explosion in early case detection through the widespread use of the prostate-specific antigen (PSA) blood test and in part because of the success of therapy. The incidence peaked in the early 1990s, a few years after the introduction of PSA as the prevalence of the disease in the previously unscreened population was detected. It then began to fall, as would have been predicted, but more recently has begun to rise again.1 This time it probably reflects the fact that sporadic early case detection has, in time, become an unofficial screening program with >80% of American men having had their PSA tested. It also reflects both the aging of the population, as well as the increasing life expectancy of modern man. Detection rates far exceed the death rates and it is clear that many more men are being detected with prostate cancer than require treatment.2 As we cannot sort out the “tigers” from the “pussycats,” radiation oncologists and urologists err on the side of caution and still treat most of those diagnosed. This poses a great stress on the limited health care resources available. It is likely that in the near future molecular markers will tell us who does not require treatment. At that time our case load will decline, and we can concentrate our energy and effective therapy on those who need it.
But which is the most effective therapy remains a burning question. External radiation has progressed substantially over the last two decades. The last Patterns of Care Survey told us that almost all radiation oncologists in the United States use three-dimensional (3-D) conformal techniques3 and new data suggest that perhaps most US centers have now switched to intensity-modulated radiation therapy (IMRT). Further, the survey documents the flight from external beam toward brachytherapy in the United States. The randomized trials tell us that conformal therapy reduces morbidity and that the increased radiation dose this allows gives higher cure rates. This is a superb example of practice changing around strong evidence. The introduction of intensity-modulated radiation and now, in an increasing number of centers, charged particle beam will likely produce dosimetric gains but whether these will yield detectable clinical gains for the patient remains uncertain.
These substantial technical improvements have, however, made external radiation much more lengthy and expensive. As a result, alternative approaches have been proposed in an attempt to reduce the social and economic burden. One is the use of hypofractionation, a previously heretical concept that has reappeared because of new evidence suggesting that prostate cancer may have a low α/β ratio.4 This concept is now being tested in randomized trials in the United States, Canada, and the United Kingdom. The other approach is the use of brachytherapy, both low- and high-dose rate. Each approach has its proponents and the early data looks favorable with both. It is the responsibility of radiation oncologists over the next decade to obtain and then sift the data to determine which offer real advantages and to which specific patients. If charged particle beam is to remain competitive with lower cost brachytherapy, it will have to prove either greater clinical efficacy or a lower morbidity to justify its use. It is possible that, with its high level of conformity, charged particle beam could also lend itself to hypofractionation to reduce cost and increase convenience; this approach has been explored with carbon ion beam treatment for prostate cancer (see subsequent text).
Patients with early prostate cancer have a disease that is so likely to be cured that quality of life issues become very important for the survivors. These are now being reported using appropriate instruments at an increasing rate and it is evidence from these that will likely become one of
the important ways that patients and physicians choose therapy in the years ahead.
the important ways that patients and physicians choose therapy in the years ahead.
In locally advanced disease, there have been tremendous efforts by the large US and European cooperative groups to perform trials, answer questions, and lay down standards of practice. The role of androgen therapy combined with external radiation has become better defined. We can now state that low-risk disease probably does not need it, intermediate-risk probably does but only as a short neoadjuvant course, and high-risk prostate cancer certainly needs it for years in a manner akin to breast cancer.5,6 The role of pelvic lymph node irradiation in locally advanced disease is one that will not go away.7 Radiation Therapy Oncology Group (RTOG) 9413 has suggested that there may be a small disease specific survival advantage when it is given and now new technology is being turned to treating the lymph nodes in a manner less morbid than in the past.
Prostate cancer now joins breast, testis, and lymphoma as an exemplary field of clinical research in which one trial builds on the shoulders of the next and piece by piece knowledge is built up to create a clearer picture of management. If charged particle irradiation is to become an established method of delivering highly conformal external beam, it will have to justify its use and its expense in terms of improved patient through randomized controlled trials.
CURRENT PROTON TREATMENT TECHNIQUES
For the first two decades of prostate cancer treatment at the Massachusetts General Hospital (MGH), the approach was limited by the range of protons available (160 MV, with range of ˜ 18 cm in water) and the fixed horizontal nature of the beam. The most direct and shortest route to the prostate was through the perineum and this was the route chosen for the beam (see Fig. 17.1). Patients were treated in lithotomy position with their legs supported in stirrups.8 During treatments, a balloon was inserted 12 to 15 cm into the rectum around a Lucite probe. It was inflated with 25 to 50 mL of saline along its length and served to immobilize the prostate and displaced the posterior rectal wall out from the path of the beam. Daily portal images were performed throughout the first phase of treatment, imaging the bones and also the metal markers within the Lucite probe that lay against the anterior rectal wall to minimize setup error. As proton beam provides limited skin sparing, the full dose could not be given through the perineum and therefore protons were just used for the boost. A four-field photon technique was used for the prostate and regional lymph nodes for 50 Gy. The proton boost phase preceded the photon phase as it was felt the rectal probe may prove intolerable after 50 Gy.
When the patient-dedicated facility with a rotating gantry and 230-MeV beam opened at the MGH, the technique was changed to that which had been developed by the group at Loma Linda University Medical Center (LLUMC) using opposed lateral beams. This is an effective way to deliver no dose to the posterior rectum. Both body cast immobilization and leg braces have been employed for patient immobilization and appear equally effective. For daily image-guidance, the MGH group now uses transabdominal ultrasound but have also used gold fiducial
markers in the past. The group at LLUMC still chooses to use a daily rectal balloon, which not only displaces the posterior rectum but also appears to help fix an otherwise mobile prostate. Other centers are following this lead.
markers in the past. The group at LLUMC still chooses to use a daily rectal balloon, which not only displaces the posterior rectum but also appears to help fix an otherwise mobile prostate. Other centers are following this lead.
Currently, low-risk patients at MGH receive treatment exclusively with lateral proton beams receiving 45 Gy (RBE) at 1.8 Gy (RBE) per fraction to a clinical target volume (CTV) that includes the prostate, proximal seminal vesicles, and a 1.0-cm margin. The posterior margin is only 0.5 cm to reduce the risk of rectal morbidity. The boost delivers 30 to 34 Gy (RBE) at 1.8 to 2.0 Gy (RBE) per fraction to a CTV that consists of the prostate alone and a 0.5-cm margin all around (see Fig. 17.2).
Higher-risk patients who may have micrometastases within the pelvic lymph nodes receive combined photon-proton treatment as well as androgen deprivation of duration appropriate to their risk group (4 months for intermediate risk, 2 years for high risk). The pelvic node treatment consists of 45 Gy delivered using IMRT techniques to spare some of the central structures such as small bowel and bladder. The CTV consists of the prostate and seminal vesicles as described for low-risk patients but also the internal and external iliac vessels with a 2-cm margin according to the recommendations of Shih et al.9 When image guidance is used and daily shifts in the field are made to account for daily motion of the prostate, it must be remembered that the nodes are fixed structures and moving the field to accommodate the prostate may lead to a geographic miss of the nodes; hence the generous 2-cm margin. Protons could also be used to effectively cover this larger nodal volume and this will be a future area of study.
Although the sharp dose falloff beyond the proton Bragg peak presents a valuable tool for tissue sparing distally to the tumor, precise positioning of the distal dose gradient is complicated by the uncertainties in the proton penetration depth. In a fractionated treatment course, radiologic path length for the incoming proton beam may vary day to day due to misalignment of tissue inhomogeneities, especially bony structures, and the compensator, because of variation in the patient positioning.10 To ensure the target coverage, a technique called compensator smearing is employed at the MGH proton center. An idealized range compensator is first designed by the treatment planning system based on the planning computed tomography (CT) data set. The compensator map consists of hexagonal elements, 3 mm in diameter, which are assigned the thickness of material necessary to stop the protons passing through the given element at the distal surface of the tumor: typically the 98% isodose is matched to the planning target outline.11 The “smear” algorithm is then applied, in which each of the hexagonal elements is reassigned the thickness to the value, that is the lowest among its nearest neighbors within a given “smear radius.” Typically, the smear radius of 3 to 5 mm is employed (and was used in treatment plans in these studies), which means that each hexagon is assigned the thickness that is the smallest among the original values for the given hexagon and its six immediate neighbors. This procedure insures that misalignment of up to 5 mm will not result in reduced dose to the target volume. However, as a result, the prescription isodose surface is pushed beyond the target volume and, inevitably, the dose to healthy tissue is increased. Compensator smearing has
been shown to reduce the effect of interfractional motion in lung tumors,12 and is expected to be similarly effective in dealing with intrafractional motion of prostate.
been shown to reduce the effect of interfractional motion in lung tumors,12 and is expected to be similarly effective in dealing with intrafractional motion of prostate.
PROTON PROSTATE CLINICAL TRIALS
The First Clinical Prostate Trial
The earliest clinical trial of proton beam in prostate cancer was the phase I/II study reported by Shipley et al. in 1979.8 Seventeen patients with locally advanced tumors were treated at the Harvard Cyclotron/MGH with pelvic photons to a dose of 50.4 Gy and a perineal proton boost to a total of 75.6 Gy (RBE) (Cobalt Gray equivalent). The perineal approach was necessitated by the range limitation imposed by the Harvard Cyclotron’s 160 MeV proton beam. The morbidity profile was favorable with only two urethral strictures, both in patients who had had prior transurethral resection of prostates (TURPs), and no severe late rectal complications.
The First Randomized Prostate Trial
In the pre-PSA era, most cases of apparently localized prostate cancer presenting to physicians were what we would now regard as locally advanced disease. This was therefore the focus of much research effort at that time. From 1982 to 1992, a total of 202 men with T3-4 tumors were randomized in a phase III trial at the MGH.13 All patients received 50.4 Gy using conventional photons and a four-field box beam arrangement to a CTV that included the prostate, seminal vesicles, and pelvic nodes. Half were randomized to receive a boost to 67.2 Gy using conventional photons through lateral portals and the other half were boosted to a total dose of 77.2 Gy (RBE) with perineal protons. Ninety-three of 103 patients randomized to the high-dose arm and 96 of 99 in the conventional dose arm received their assigned dose. The endpoints were clinical local failure based on a digital rectal exam, a positive posttreatment TURP specimen, or a positive rebiopsy (performed on a minority of patients with negative digital rectal examinations [DREs]). After 1989, PSA was used in follow-up, thereby allowing for a biochemical evaluation of outcome. There was a trend toward improved local control at 8 years in the high-dose proton group but this did not reach statistical significance (73% high dose vs. 59% low dose, p = 0.09). There was, however, a statistically significant improvement in local disease control at 5 and 8 years for the subset with Gleason 7-10 tumors treated in the high-dose arm (94% and 84% vs. 64% and 19%). The percentage of positive rebiopsies was also lower for this subset in the high-dose arm, though biases will have been introduced here by the fact that not all men with negative DREs consented to rebiopsy. This gain in local control was not seen for men with lower Gleason grade tumors.
The improved local outcome for men did not translate into a gain in terms of disease-free survival (biochemical or clinical) or overall survival. In retrospect this is not surprising. All men had large tumors and most were randomized before the use of PSA screening. Many would have PSA levels surely indicative of metastatic disease and, in a comparable contemporary trial, would not have been included as they could not stand to gain from an improved local measure. Other explanations are that the trial was underpowered or that the dose escalation was simply insufficient for that stage of disease.
Perhaps the most useful information to emerge from this trial came in from the subsequent analysis of treatment-related morbidity.14,15 It must be remembered that all patients received full pelvic photon treatment and so no difference would be expected, nor was it seen, in terms of small bowel toxicity. The sites of greatest interest were the bladder, urethra, and the rectum. The actuarial incidence of urethral stricture was seen in 19% of those in the high-dose arm and only 8% in the lose dose arm (p = 0.07). This was usually relieved by urologic intervention such that it was only a persistent problem in 2% of patients in each arm. Corresponding figures for late hematuria were 14% and 8% respectively. Among the 78 patients fully potent before irradiation who had not had endocrine therapy for a relapse, the risk of subsequent impotence was equal at 60% and 62%.
The actuarial rates of rectal bleeding were higher in the high-dose arm (32% vs. 12%) but in only one patient did this represent a grade 4 problem. To gain greater insight into the relationship between dose and rectal bleeding, a dose-volume histogram (DVH) analysis was performed on the patients receiving a proton boost, 41 of whom had bled.14 Patients receiving at least 76.5 Gy (RBE) to at least 40% of their anterior rectal wall had an 81% actuarial risk of rectal bleeding as compared with 25% who received this dose to <40%. It was also of note that the bleeding risk is not uniquely correlated with the volume receiving the highest dose of radiation; hence, there is also synergy with other parts of the DVH. Therefore, for a given, fixed high dose and volume, the risk increases according to the larger volume receiving a lower dose. A particularly potent synergy comes when >60 Gy is given to more than 70% of the anterior rectal wall (see Fig. 17.3). No subportion of the DVH dominates and Hartford et al. have made tables of statistically significant and equivalent risk combinations.15
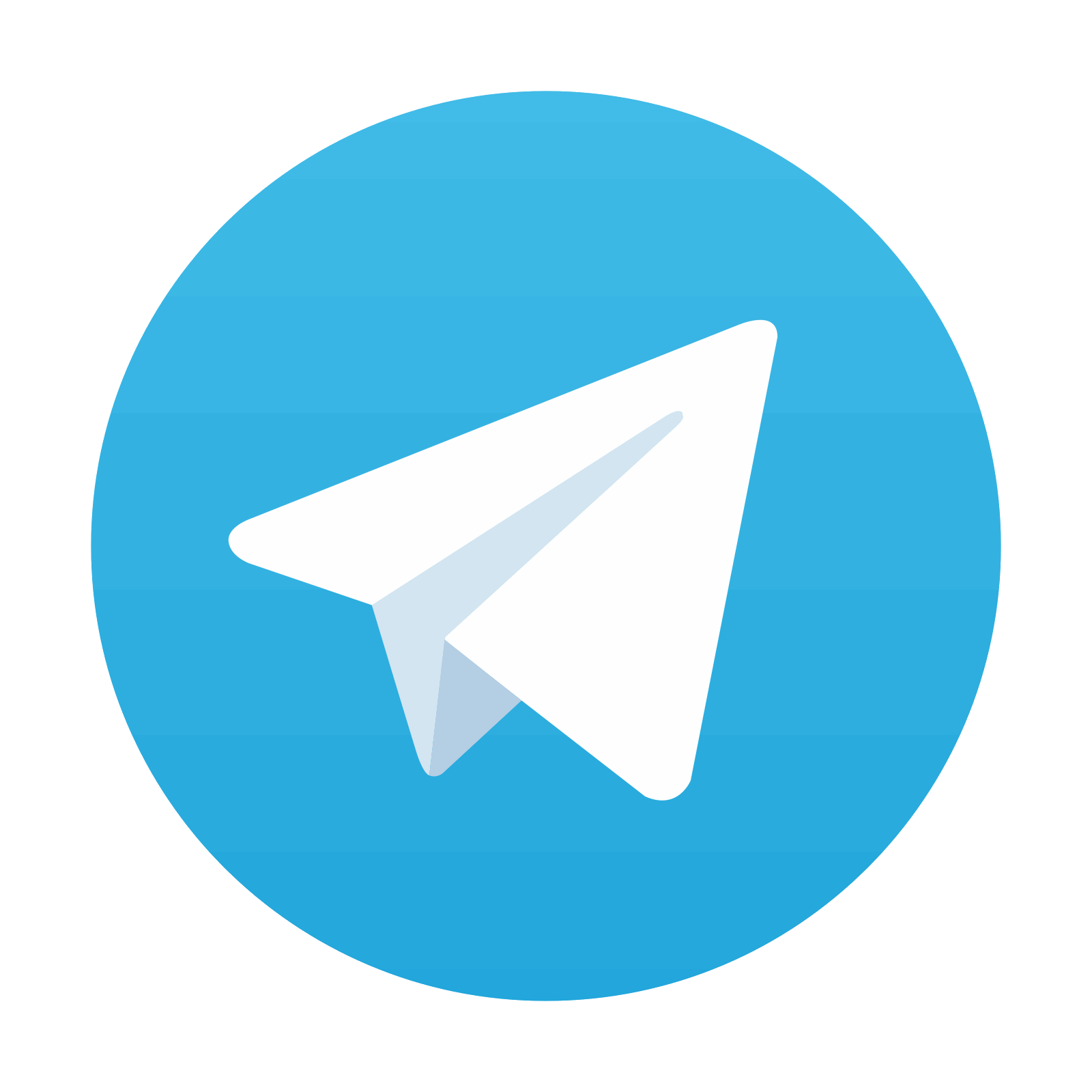
Stay updated, free articles. Join our Telegram channel

Full access? Get Clinical Tree
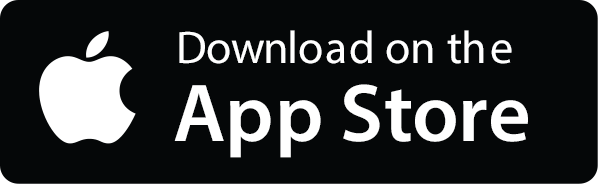
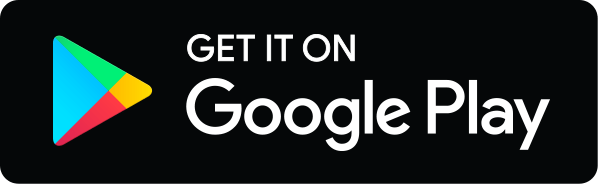