249CHAPTER 16
Prostate SBRT
Prostate cancer remains the second most common malignancy in men worldwide, with rising incidence particularly in developed countries (1). Rates of overall death have, however, been declining on average 3.5% per year since 2004 in the United States, which may reflect the introduction of prostate-specific antigen (PSA) screening and therapy advancements (2). Radiation therapy (RT) provides a critical curative means to treat localized prostate cancer. Rapid technological advancements during the past two decades including three-dimensional planning and dose escalation via conformal techniques with daily image guidance can also be attributed to these mortality reductions by way of improved progression-free survival (3–6). Similarly, with the advent of more conformal intensity-modulated radiotherapy (IMRT), dose escalation can provide higher rates of disease cure while reducing the risk of long-term toxicity (7).
In an increasingly cost-conscious environment and with techniques now enabling better sparing of nearby critical organs, focus has shifted to provide more convenient and potentially cost-effective therapeutic measures. Included in these RT advancements is the concept of moderate hypofractionation, traditionally using doses ranging from 2.5 to 3 Gy per day as opposed to 1.8 to 2 Gy used in conventional RT regimens. This was initially explored with the hypothesis of improved disease outcomes on the basis of radiobiological modeling. Within the linear-quadratic model, the alpha–beta (α/β) ratio represents the dose threshold where cell kill is equally provided by the linear and quadratic components. Slowly proliferating cells, most commonly late-responding normal tissue, would be expected to have a low α/β ratio, whereas rapidly proliferating cells such as most cancer cell lines would have the opposite. Modeling from the application of clinical data in fact suggested the contrary for prostate adenocarcinoma, where the α/β is closer to 1.2 to 1.5 Gy (8–10). In an attempt to capitalize on this ratio relative to rectal tissue, trials assessing moderate hypofractionation have been conducted, with results suggesting similar curative rates in exchange for equal or slightly increased late toxicity (11–14).
Supporters of hypofractionation argue that establishing superiority may require even higher doses per fraction (≥5 Gy), which would also capitalize on the ability to reduce treatment costs and enhance patient convenience with fewer treatment visits. Stereotactic body radiation therapy (SBRT) allows for this unique opportunity by way of further refined conformity, introducing the concept of extreme hypofractionation. Considered a more novel treatment approach, mounting data suggest that with meticulous planning and implementation, SBRT as a means for extreme hypofractionation is more than an experimental option, providing excellent outcomes in appropriately selected patients and opening the door to broader application in the treatment of prostate cancer.
INDICATIONS FOR PROSTATE SBRT
Primary Treatment
Prostate cancer management is driven by patient life expectancy, patient preference, and disease risk stratification, the latter related to initial PSA at the 250time of diagnosis, tumor staging, and Gleason score. Monotherapy, mainly by way of prostatectomy or RT, has been well established for low-risk prostate cancer (T1a–T2a, Gleason score ≤6, and initial PSA <10) with curative rates exceeding 90% (15, 16). With high survival rates and the costs, both financial and in the form of toxicities, active surveillance using a strict protocol for intervention has been explored with favorable rates in prospective, observational studies for low-risk patients (17, 18). In the landmark ProtecT (Prostate Testing for Cancer and Treatment) trial in which patients with mostly low-risk disease were randomized to active surveillance to active treatment, no difference in overall survival or cancer-specific survival was seen at 10 years between the two groups, arguing for active surveillance to be a first-line recommendation for low-risk prostate cancer (16). Yet on these trials assessing active surveillance, 45% to 59% of men either developed disease progression or opted to proceed with treatment, the latter attributable to psychological stress of diagnosis without active management (16–18).
For patients who opt to proceed with active treatment, external beam radiation therapy (EBRT) is a proven, minimally invasive option delivered to doses of 75.6 to 79.2 Gy over 42 to 44 daily fractions (19). In addition to the socioeconomic demands of daily treatment for 2 months, financial concerns have been raised with direct Medicare costs in the modern era of $18,000 to $31,574 (20, 21). Capitalizing on the presumed radiobiological benefit of extreme hypofractionation, SBRT provides an appealing method to improve patient convenience through fewer visits and reduce direct medical costs. Medicare reimbursement rates for a course of SBRT are notably lower, estimated at $14,315 to $20,899 (22, 23). These estimates exclude resource demand reduction and indirect patient costs such as required housing, travel, and reduced work hours associated with daily conventional RT. Even so, two studies have now shown cost-effectiveness of SBRT over IMRT, maintaining superiority even if slight decrements are seen in efficacy and/or toxicity (22, 23).
Technical feasibility of SBRT for prostate cancer was shown in the early 2000s using a CyberKnife® treatment platform (Accuray Inc., Sunnyvale, CA) (24). In this dosimetric feasibility study, plans generated with CyberKnife, compared with step-and-shoot IMRT, had improved sparing of bladder and rectum while providing comparable prostate coverage. Propelled by technical feasibility data and the wave of interest in hypofractionation as a whole, multiple retrospective and phases I and II single-arm prospective studies have been reported with variable platforms, showing favorable early outcomes and acceptable rates of toxicity (Table 16.1) (25–41). A vast majority of patients in these reported studies have low- or favorable intermediate-risk disease.
First prospectively reported data from the phases I and II SHARP (Stereotactic Hypofractionated Accelerated Radiation to the Prostate) studies evaluated 40 patients treated to 33.5 Gy in 5 fractions to the prostate via non-coplanar beams and a linear accelerator-based SBRT technique (37). Four-year biochemical progression-free survival (bPFS) was 90% by modern definition with no grade 3 or greater toxicities reported in the acute nor late setting. In more recent years, a number of other prospective studies have corroborated these findings. King et al. provided a pooled analysis of patients prospectively treated in multiple institutional phase II studies, including a landmark 1,100 patients (25). With almost all receiving 35 to 36.25 Gy in five fractions (89%) using a CyberKnife treatment platform, the 5-year bPFS rates were 95.2%, 84.1%, and 81.2% for low-, intermediate-, and high-risk patients, respectively. Recent release of a multi-institutional prospective study using CyberKnife confirmed a similar rate of 5-year bPFS of 97.1%, with high rates or both low-risk (97.3%) and favorable intermediate-risk (97.1%) patients and 1.6% rate of grade 3 toxicities (40). For comparison, the 5-year biochemical recurrence rates on Radiation Therapy Oncology Group (RTOG) 0415 were 6.3% to 8.1% between conventional and moderate hypofractionation (14). Although 21% of patients were treated with 3D conformal therapy as opposed to IMRT, late severe (grade ≥3) gastrointestinal (GI) and genitourinary (GU) toxicities rates on RTOG 0415 were 2.6% to 4.1% and 2.3% to 3.5%, respectively. Report of the Registry for Prostate Cancer Radiosurgery (RPCR), including a robust 1,743 patients, identified only 1 case of grade 3 urinary toxicity (42).
The overwhelming preponderance of data now for SBRT has led to a revision in national guidelines and organizational recommendations. In 2013, the American Society for Radiation Oncology (ASTRO) provided a Model Policy supporting the use of SBRT for “select patients with low- to intermediate-risk disease” (43). National Comprehensive Cancer Network guidelines have been more cautious to adopt this option routinely with current guidelines expressing interest in long-term follow-up but allowing SBRT as “an alternative to conventionally fractionated regimens at clinics with appropriate technology, physics, and clinical expertise” (44). Growing support has nevertheless led to the ongoing phase II NRG/RTOG 0938, which randomizes low-risk patients (Gleason ≤6, clinical stage T1–2a, and PSA <10) to extreme hypofractionation (36.25 Gy in 5 fractions) or moderate hypofractionation (51.6 Gy in 12 fractions), stratified by treatment platform. In all, this would suggest that SBRT is a reasonable option in experienced centers and particularly on a clinical protocol or registry until long-term (10-year) data are finally reached.
251
Primary treatment of intermediate- and high-risk disease with prostate SBRT remains more controversial as existing data largely contain low-risk patients. The failure thus far to prove a survival benefit to prophylactic whole pelvic RT has buoyed support for localized SBRT, often encompassing the seminal vesicles and prostate with or without androgen deprivation therapy (ADT) (45, 46). An evolving definition of “intermediate-risk disease” has also established a favorable intermediate-risk cohort, defined as having Gleason 3 + 4 disease with less than 50% cores positive and only one adverse risk factor (T2b–c, Gleason 3 + 4 disease, or PSA 10–20) (44). Among intermediate-risk patients, favorable intermediate-risk patients have low rates of biochemical relapse, distant metastases, and prostate cancer–specific mortality (47). Such patients may be best suited being treated with monotherapy akin to low-risk prostate cancer and thus may be excellent candidates for primary SBRT.
252In their analysis, King et al. reported on 334 patients (30%) with intermediate-risk disease treated with primary CyberKnife SBRT, of whom 15% received ADT (25). The 5-year bPFS rate was 84.1% in the entire cohort. No difference was seen in outcomes with or without ADT, although there was a nonsignificant numerical benefit (5-year bPFS, 79.7% vs. 97.2%, p = .17). Meier et al. included more favorable intermediate-risk patients (T1c–2b with either Gleason 7 and PSA <10 or Gleason 6 and PSA 10–20), revealing a high 5-year bPFS rate of 97.1% in this group (40). Katz et al. published on 153 intermediate-risk patients, most (69%) with favorable intermediate-risk disease (48). The 8-year bPFS rate was 84.3% in all intermediate-risk patients. As suspected, favorable intermediate-risk patients had a noticeably higher rate of bPFS (93.2% vs. 68.2%, p < .0001). Again, no significant benefit was seen with the addition of ADT. These findings would support primary SBRT for favorable intermediate-risk patients with outcomes similar to modern results for low-risk disease treated with conventionally fractionated RT. The persistent lack of benefit for ADT seen in these studies suggests that further dose escalation via extreme hypofractionation may diminish any advantage.
In contrast, high-risk patients have been shown to benefit from RT dose escalation, conventionally up to doses of 79.2 to 86.4 Gy, at the cost of increased bowel toxicities (3–5, 49). Coupled with no proven benefit to pelvic field RT, primary SBRT with or without ADT may be a reasonable consideration for organ-confined disease, although there are limited supporting data at present. As a benchmark, modern rates of bPFS for high-risk patients treated with ADT and dose-escalated EBRT range from 67.9 to 88.0 Gy (49–51). High-risk patients treated with or without ADT represented 125 patients (11%) of those reported by King et al. (25). The 5-year bPFS for these patients was 81.2%, despite only 38% receiving ADT. Katz et al. reviewed 38 patients with high-risk disease, showing similar 8-year bPFS rates with primary SBRT for organ-confined disease (8-year rate, 65.0%) (48). A larger proportion received ADT (55%) compared with the series by King et al., despite an overall lower bPFS rate. With emerging benefits of docetaxel and long-term ADT, both of these may play a role with primary SBRT, although they have largely not yet been examined in prospective fashion with mature results (50, 52, 53). As such, until complete maturation of SBRT results and final determination of the role of pelvic RT and/or ADT, treatment of unfavorable intermediate-risk or high-risk disease should be judiciously considered for organ-confined disease with vigilant follow-up.
SBRT Boost
Dose escalation through conventionally fractionated RT has been established as a method to improve bPFS in intermediate- and high-risk patients (3–6). Further dose escalation was initially explored via brachytherapy, providing a highly conformal method of boost. The first trial comparing a brachytherapy boost with EBRT alone in mostly (53%) high-risk patients, while showing a relapse-free survival benefit, was criticized for applicability because of the low cumulative dose on the external beam only arm (54). In contrast, the ASCENDE-RT (Androgen Suppression Combined with Elective Nodal and Dose Escalated Radiation Therapy) trial published this year provides a more appropriate randomized comparison of dose-escalated EBRT (78 Gy at 2 Gy per fraction) to 46 Gy EBRT with a low-dose-rate (LDR) brachytherapy boost, all receiving 12 months of ADT. Among these intermediate- and high-risk groups, addition of a brachytherapy boost led to further improvement in 7-year bPFS (75% vs. 86%, p = .004), albeit at the cost of increased late grade 3 urinary toxicities (5% vs. 19%, p < .0001) (55, 56). These data have reinvigorated the role of further dose escalation for unfavorable intermediate- and high-risk prostate cancer patients.
Few studies have examined a similar approach as done with brachytherapy, introducing a SBRT boost following EBRT for intermediate- and/or high-risk patients (Table 16.2) (34, 57–60). The predominant benefit to SBRT boost over brachytherapy would be avoidance of an invasive surgical procedure, particularly in an aging population. In addition, the increase in late GU toxicities with brachytherapy boost, mainly representing urethral stricture, challenges the potential bPFS benefit. Lower localized hot spots and lesser dose heterogeneity using SBRT may provide a suitable balance between dose escalation and toxicities (61).
Existing literature supports a boost dose of 19 to 21 Gy in two to three fractions following EBRT, providing favorable early rates of bPFS (34, 57–60). Among 97 patients treated with either primary SBRT or SBRT boost after EBRT for unfavorable intermediate- or high-risk disease, Katz et al. showed no difference in bPFS between the two treatment groups nor with the addition of ADT (34). With the limitation of this being in a relatively small group of patients, these results would add to skepticism about the role of pelvic RT or ADT in the setting of extreme dose escalation. Interestingly, the published retrospective results for SBRT boost suggest lower rates of severe late toxicity (grade ≥3, 0%–7%) compared with those seen in the ASCENDE-RT trial (54). Nonetheless, with a paucity of data, patients managed with this approach should be followed prospectively on a clinical protocol.
253
Local Salvage Following Radiation Therapy
Despite improvements in treatment delivery and feasibility of dose escalation with or without ADT, a proportion of patients treated with conventional RT will still experience biochemical failure, most commonly those with intermediate- and high-risk disease. Roughly two-thirds have been shown to have biopsy-proven local recurrence in the setting of biochemical failure (62). In the smaller subset with isolated local recurrence, salvage therapy can be particularly challenging. Local therapy options ranging from radical prostatectomy, cryotherapy, brachytherapy, and SBRT have been explored with variable rates of success and toxicities.
Salvage radical prostatectomy has long been reported with the strongest data arising from a multi-institutional retrospective cohort (63). A majority (68%) were treated earlier with EBRT with or without brachytherapy. Following salvage prostatectomy, completed at a median of 41 months from RT, the 5-year bPFS was a modest 48%. Preoperative PSA and biopsy Gleason score were independent predictors of bPFS with the most favorable outcomes among those with Gleason ≤7 prostate cancer at the time of recurrence and PSA ≤4. Appropriate identification of this cohort is critical as 30-day complication rates can reach 51% with long-term risks of rectal injury and anastomotic stricture (64). Alternatively, relatively small, single-institution series have reported on the use of salvage LDR or high dose rate (HDR) brachytherapy (65–68). Similar to prostatectomy, rates of bPFS range from 25% to 75%, driven by presalvage characteristics, and cumulative crude grades 3 to 4 GU and GI toxicity rates were 13% and 5%, respectively (68). More favorable outcomes have been seen using HDR brachytherapy (65, 66). Such findings have led to the accrued RTOG 0526, which is using salvage LDR brachytherapy in select patients.
In light of dosimetric similarities between intact prostate HDR brachytherapy and prostate SBRT, salvage SBRT has also been explored as a means to limit reirradiation to surrounding critical organs (Table 16.3). Vavassori et al. initially reported on feasibility in six patients treated using a CyberKnife platform to deliver 30 Gy in five fractions after a median EBRT dose of 80 Gy (69). In this limited subset with only 1-year outcomes, four of six patients developed biochemical failure, although none within the prostate bed and no severe toxicities were reported. Notably, these patients had a very short median interval to reirradiation (13.5 months), which reflected in the high rate of biochemical and distant failure despite local control. Other published series, all likewise suffering from the same limitation of short follow-up, appear to have more favorable biochemical control rates among more selective patients and no reported severe toxicities as of yet (70–73). Application of salvage SBRT in this setting is, however, in its infancy with requirement of more data and follow-up in light of the high risks associated with reirradiation.
EXCLUSION CRITERIA FOR PROSTATE SBRT
As existing literature is largely confined to short-term follow-up and retrospective studies, prostate SBRT should be conducted at a high-volume facility with appropriate technical support and expertise. Ideally, patients should be treated on a clinical protocol with meticulous follow-up. Nonetheless, a plethora of data now exists for low- and favorable intermediate-risk patients, such that prostate SBRT can be considered an efficacious and safe definitive treatment option in an equipped and experienced center. Maturation of existing phase II data and future completion of RTOG 0938 may ultimately support widespread adoption of prostate SBRT.
254Aside from those for any form of RT (i.e., scleroderma, active inflammatory bowel disease), SBRT otherwise has few data-driven contraindications. With the high doses delivered per fraction, one could reasonably extrapolate relative contraindications from brachytherapy and moderate hypofractionation literature. Compared with conventionally fractionated RT, Pollack et al. demonstrated increased late grade ≥2 urinary toxicities with modest hypo-fractionation (hazard ratio [HR]: 4.41, p < .001) for patients with higher baseline urinary symptoms, defined as having an International Prostate Symptom Score (IPSS) greater than 12 (11). The American Brachytherapy Society (ABS) provides IPSS greater than 20 as a relative contraindication to LDR brachytherapy (74). However, the ABS guidelines for HDR brachytherapy still provide this as an option even in patients with significant baseline symptoms, albeit with appropriate counseling (75). Although LDR literature does indeed suggest an increase in urinary toxicities for patients with considerable baseline symptoms, the data for HDR brachytherapy are less distinct (76, 77). This experience is similar for prostate SBRT, where existing data have not shown an association with baseline urinary symptoms and the risk of acute or chronic urinary toxicities. Transient increases in IPSS and Expanded Prostate Cancer Index Composite (EPIC)-26 urinary scores are still expected after prostate SBRT with 13% experiencing late urinary flare symptoms as well (78, 79). Thus, appropriate counseling with patients about this potential risk may still be advised for those with exceptionally significant baseline lower urinary tract symptoms (IPSS >20).
Although larger gland size (>60 cm3), prominent median lobe, and substantial transurethral resection of the prostate (TURP) defect are all relative contraindications for LDR brachytherapy, these are driven largely by technical challenges of implantation that should not solely preclude a patient from SBRT (74). One study evaluating patients with larger prostate gland size (≥50 cm3) treated with SBRT found higher rates of grade 2 acute GU toxicities (50% vs. 35%) compared with their earlier published experience with varying gland size (28, 80). Seymour et al. revealed a roughly fivefold increase in the risk of late grade 2 or greater GU toxicities if gland size is 50 cm3 or higher (81). Prostate volume as a continuous variable was also found to be predictive for the risk of late toxicity with a 4% relative increase per cubic centimeter. Katz et al. revealed a trend toward significantly high rates of grade 2 (11.6% vs. 5.3%) and grade 3 (3.1% vs. 0.6%, p = .051) with prostate volumes greater than 60 cm3 (82). These findings, however, may be confounded by differences in dose homogeneity, which was 255independently related to the risk of late GU toxicity (81). In all, these patients should be appropriately counseled that similar to brachytherapy literature, there may be a correlation with larger gland size being associated with a higher risk of GU toxicities, albeit not conclusively.
As with prostate brachytherapy, patients with limited life expectancy (<10 years), particularly with low- or favorable intermediate-risk disease, and non–organ-confined (T3–4 or node-positive) disease are not suitable candidates for prostate SBRT. Select T3 patients have been treated earlier with prostate SBRT, as either monotherapy or a boost after EBRT, but should not be routinely considered (33, 57, 59). Lastly, patients with inability to undergo MRI for treatment planning or with extensive hip prosthesis resulting in imaging artifact should ideally preclude prostate SBRT on the basis of inability to accurately delineate the prostate and nearby critical structures. Data regarding the limitations of non–MRI-based planning are discussed later.
TREATMENT PROCESS
Because of the high level of precision required, prostate SBRT should be conducted in a setting with adequate resources and an established workflow. Much like SBRT in general, this entails careful consideration of immobilization at the time of simulation, treatment planning processes, physics and dosimetry support with a sufficient level of training, motion management, daily image guidance, and processes to ensure follow-up. Resources specific for prostate SBRT can be ascertained from manuscripts with large published experiences, the Radiosurgery Society (RSS), and ongoing clinical protocols that are publically available (Table 16.4) (28, 29, 35, 36, 82–84).
Patient Selection Recommendations
On the basis of existing data and exclusion criteria reviewed earlier, ideal patients for primary prostate SBRT include low- and favorable intermediate-risk patients with organ confined disease (T1–2N0M0), life expectancy exceeding 10 years, no general contraindications to pelvic RT, and no specific imaging restrictions (hip prostheses artifact, inability to complete MRI). Preference for prostate SBRT over alternative RT treatment options may be driven by patient preference and prioritization of convenience and cost. Although a consistent association has not been shown, patients with considerable lower urinary tract symptoms (IPSS >20) and/or larger prostate volumes (>50–60 cm3) should be counseled about potential increases in urinary toxicities. High-risk patients with organ-confined disease may also be suitable for SBRT, pending further data defining its role amid pelvic EBRT and/or ADT.
Simulation
With required precision of prostate SBRT, the first consideration before proceeding with simulation is developing an adequate plan for immobilization, 256target localization, and reduction of intrafraction motion. At least three radiopaque fiducials should be placed transrectally or transperineally in a noncoplanar fashion with a 1- to 2-week delay from simulation to account for migration. Custom immobilization is preferred to avoid rotational errors. Patients should be positioned supine for comfort as prone positioning has not been proven to reduce prostate motion (85, 86). Accounting for bladder and rectal filling variation is critical to reduce anatomical distortion of the prostate and subsequent systematic and dosimetric errors (87–90). Variation approaches including dietary modifications (low-gas, low-residue diet), fleet enema prior to each treatment, and strict bladder filling protocols (16–24 ounces of water 45–60 minutes prior to treatment) (91–96). The goal of these approaches should be to maintain a comfortably full bladder and empty rectum. Although data are conflicting regarding the impact of dietary changes or fleet enemas, regardless of which approach is undertaken, this should be maintained consistently and assessed daily with image guidance prior to each treatment. Use of a biodegradable rectal spacer is an emerging option to reduce rectal dose and avoid changes to the prostate–rectum interface during treatment (97, 98).
TABLE 16.4 Selective Resources With Treatment Process Details
Resource | Details |
RTOG 0938 | Randomized phase II protocol Simulation, treatment planning, dosimetry constraints, plan assessment, and image guidance details on various treatment platforms |
Georgetown/Beth Israel Deaconess (83) | Review article Treatment planning and image guidance details for CyberKnife platform |
Sunnybrook (36) | Phase I/II trial Treatment planning, dosimetry constraints, and image guidance details for linac-based SBRT |
Georgetown (28) | Retrospective Treatment planning, dosimetry constraints, plan assessment for CyberKnife platform |
Stanford (35) | Phase II trial Treatment planning and dosimetry constraints for CyberKnife platform |
Stanford/Naples (29) | Retrospective pooled analysis Simulation, treatment planning, dosimetry constraints for CyberKnife platform |
Flushing (145) | Prospective/retrospective Simulation, treatment planning, dosimetry constraints for CyberKnife platform |
SBRT, stereotactic body radiation therapy.
Non–contrast-enhanced CT simulation should be completed with 2.5-mm slice thickness or less. Prostate MRI should be ideally completed on the same day to avoid anatomical variability from bladder and rectal changes. MRI should be done without an endorectal coil and with preference toward thin-cut (≤2.5-mm slice thickness) T2-weighted images for sufficient delineation of the prostate gland. CT imaging alone is inferior to MRI by way of overestimation of prostate contours, difficulty in differentiating the apex of the gland, and inability to identify the neurovascular bundles and prostatic urethra (99, 100). For patients unable to undergo MRI, retrograde urethrogram via injection of 8 to 10 mL of contrast into a urinary catheter or the penile urethra may overcome the latter two limitations (101). Still, inability to assess the adjacent soft tissues and overestimation of prostate volume can lead to an increased risk of toxicities following SBRT; thus, simulation without ability to perform an MRI should be carefully reconsidered (102).
Target Delineation
T2-weighted MRI images should be fused to the simulation CT with respect to the prostate contour along the bladder and rectum, not bony anatomy. Image fusion should be meticulously scrutinized to avoid systematic error. The clinical target volume (CTV) should consist of the entire prostate defined by MRI for organ-confined disease (Figure 16.1). The prostatic base and apex are best identified by MRI. Inclusion of the proximal seminal vesicles should be considered for intermediate- and high-risk patients on the basis of the risk of microscopic involvement. The extent of inclusion is typically within the first 2 cm on the basis of surgical series, although this should be in the context of rectal constraints achieved (103). The planning target volume (PTV) should involve a margin of 3 to 5 mm on the basis of institutional setup error. A 3-mm margin is favored posteriorly to reduce overlap with the rectum. Organs at risk that should be identified and contoured include the rectum, bladder, penile bulb, femoral heads, prostatic urethra, neurovascular bundles, sigmoid and small bowel (if applicable), and skin (Figure 16.1).
257
FIGURE 16.1 Depictions of prostate and organ-at-risk contours. (A and C) Corresponding CT and MRI images identifying the prostate (red), prostatic urethra (brown), neurovascular bundles (cyan), rectum (brown), and femoral heads (dark blue and dark green). (B and D) Corresponding CT and MRI images, with the latter enabling better delineation of the prostatic apex. (E) The prostate (red) and 3-mm expansion to create the planning target volume (PTV, orange) with implanted fiducials denoted by imaging artifact. (F) Three-dimensional representation of contours: prostate (red), bladder (yellow), proximal seminal vesicles (blue), neurovascular bundles (cyan), rectum (brown), and penile bulb (purple).
Treatment Planning
Platforms
Various treatment platforms exist for delivery of prostate SBRT, including linear accelerator (linac)-based, CyberKnife, TomoTherapy™ (Accuray Inc.), and proton therapy. CyberKnife robotic radiosurgery allows for use of numerous noncoplanar beams and intrafraction motion assessment that was not traditionally feasible through alternative platforms. Addition of motion management systems such as Calypso (Varian Medical Systems, Inc., Palo Alto, CA) and ExacTrac (BrainLAB, Munich, Germany) and rotational arc therapy have narrowed these differences (104, 105). Conversely, the use of IMRT in lieu of SBRT techniques has been shown to be dosimetrically inferior with reduced dose conformity, lower PTV coverage, and higher doses to adjacent bladder and rectum (106–108). Passive scatter proton therapy has lower conformality indices compared with CyberKnife or TomoTherapy (109). In regards to patient convenience, flattening filter-free mode available on linac-based SBRT has enabled a considerable reduction in overall treatment time, although there is less awareness of the radiobiological implications (110). In all, no outcome data tout superiority of one platform over another and, thus, all are allowed on RTOG 0938. Stratification of patients by platform on this study will hopefully provide the opportunity for subset analysis.
Treatment Planning
Fractionation Schemes
Most published series support the use of 35 to 36.25 Gy in five fractions delivered either daily or every other day. This fractionation scheme consisted of the most common regimens (89%) in the 1,100-patient, prospective, multi-institutional analysis (25). The question of further dose escalation arose from the nonsignificant improvement in 5-year bPFS in this series: 92.5% with 35 Gy, 90.7% with 36.25 Gy (p = .08), and 95.8% with 38 to 40 Gy (p = .83). This trend was more pronounced among intermediate-risk patients: 72.3% with 35 Gy, 87.2% with 36.25 Gy (p = .73), and 96.7% with 38 to 40 Gy (p = .58). Assuming an α/β value of 1.5, the respective equivalent 2 Gy doses (EQD2) are 113, 121, and 132 to 145 Gy. The lack of a dose–response relationship may reflect the exceedingly high EQD2, which are likely beyond the steep gradient of the dose–response curve for prostate cancer. As shown in a phase I/II trial, further dose escalation up to 50 Gy in five fractions led to five patients (8.2%) in the high-dose arm having grades 3 to 4 rectal toxicity requiring a diverting colostomy (31, 111). Katz et al. have also shown an increase in grades 2 to 3 late urinary (13% vs. 1%) and rectal (2.9% vs. 0%) toxicities with 36.25 Gy as opposed to 35 Gy delivered in equal daily fractions (112). No difference in bPFS was seen between these two fractionation regimens. On the contrary, Quon et al. evaluated two separate fractionation schemes (35 or 40 Gy in five weekly fractions) delivered on prospective trials, showing no difference in quality of life survey scores between the two regimens (113). When using 36.25 Gy in five fractions, King et al. identified lower rates of late grade 1 to 2 GU (17% vs. 56%, p = .007) and GI (5% vs. 44%, p = .001) toxicities with treatment every other day rather than daily fractionation (35). These findings compiled together should bring pause to escalation of SBRT doses beyond 40 Gy, with preference toward regimens of 35 to 40 Gy in five weekly fractions, 36.25 Gy in five fractions delivered every other day, or 35 Gy in five fractions daily.
258Dose Constraints
Prospective trial constraints and those supported by toxicity data are listed (Table 16.5). In general, prescription dose coverage of the PTV should be 95% or greater. Published series have also used a simultaneous integrated boost to the prostate or dominant nodule seen on MRI (26, 30). At minimum, 99% or more of the prostate should receive the prescription dose, excluding the urethra if constrained. Correlations have been made with late grade 2 and greater urinary toxicities with dose heterogeneity within the prostate (114). For this reason, the prescription isodose line should be greater than 80% on CyberKnife treatment plans if not higher on alternative platforms. Priority should otherwise be placed on the high-dose volume of the rectum, rectal wall circumference dose, and mean and maximum point urethral doses on the basis of published data (30, 81, 111, 115). Although urethral dose reduction was initially avoided to prevent local failures, emerging data regarding urethral dose and known toxicity data from brachytherapy should trump concerns about prostatic disease control, particularly without supporting data to this claim.
259Plan Evaluation
A sample plan is provided using volumetric arc therapy and CyberKnife to deliver 36.25 Gy in five fractions to the prostate with a 3-mm margin (Figure 16.2). Limiting the urethral hot spot can be completed as shown in Figure 16.3. When using the CyberKnife stereotactic treatment platform, 120 to 160 beams should be used to achieve adequate PTV and prostate coverage as outlined earlier. The prescription isodose line selected should be no lower than 80% to reduce intraprostatic hot spots. Treatment time should be below 60 minutes per fraction to prioritize patient comfort. Newer models may be more readily capable of achieving this with the Iris collimator or higher MU (monitor unit) output. If linac-based SBRT is pursued, noncoplanar beam arrangements are preferred. Rotational arc therapy may also be used with no suggested dosimetric differences between the use of a single or two arcs (116). Once a suitable treatment plan, beam arrangement, and isodose line are selected, target organs should be more closely assessed with prioritization toward the rectum, urethra, bladder, penile bulb, and remaining critical structures in this respective order (Figure 16.4).
Treatment Delivery
Patients arriving for treatment should undergo similar bowel and bladder preparation as done for simulation. Linac-based SBRT should be coupled with daily cone beam CT imaging to assess bladder filling, rectal distension, and adequate alignment of the prostate target. Fiducials placed earlier can be used for guidance (117). Patients treated with CyberKnife platforms should have orthogonal films completed first for verification of fiducial placement.
Motion Management
Intrafraction prostatic motion is a well-documented phenomenon, a combination of respiratory motion and variations in rectal and bladder filling (118–121). Combining the longer typical treatment times for prostate SBRT, accounting for prostatic motion can facilitate in reducing margins to reduce toxicities and avoid prostate target miss. Patients treated with volumetric modulated arc therapy, particularly with a flattening filter-free mode, can receive prostate SBRT in minutes, reducing the impact of intrafraction motion (116, 122, 123). For other SBRT platforms, prolonged treatment times have been clearly correlated with a higher risk of significant intrafraction motion (124, 125). Although abdominal compression is often used for thoracic SBRT, this device has not been shown to have as significant an impact for prostate SBRT and is thus not recommended to be solely used (126).
FIGURE 16.2 Isodose lines for treatment plans using volumetric arc therapy (left) and the CyberKnife platform (right).
FIGURE 16.3 Example of urethral dose-sparing technique via volumetric arc therapy, limiting the prostatic urethra to a dose below the prescription isodose line.
260FIGURE 16.4 Example of an acceptable dose–volume histogram for delivery of 36.25 Gy in five fractions with a simultaneous integrated boost of 40 Gy to the prostate using volumetric arc therapy.
Established methods to assess and correct for intrafraction motion include the Calypso tracking device and stereoscopic kV x-ray imaging (121, 127). The Calypso 4D Localization System uses beacon electromagnetic transponders implanted into the prostate to be detected and tracked in three dimensions through the course of RT. Kupelian et al. provided a large experience using Calypso for fractionated RT with 41% of fractions having motion exceeding the 3-mm threshold (127). Alternatively, stereoscopic kV x-ray imaging can similarly account for prostate motion and avoid target miss (107, 121, 128). Xie et al. examined the use of orthogonal films using a CyberKnife platform for prostate SBRT. In this study, prostate movement thres-holds were set at 5 mm, with a 30-second sampling interval enabling a vast majority (94.6%) of movement to be within 2 mm (121). Motion beyond 2 mm was marginally increased (7.5% of datasets) with a longer 60-second sampling interval. Through stereoscopic kV imaging, extreme variations in intrafraction motion have been shown to reduce prostate prescription coverage, such that not accounting for these differences may compromise outcomes (107). In all, intrafraction and interfraction prostate motion should be accounted for by daily image guidance and motion tracking via electromagnetic transponders or orthogonal kV imaging at a minimum of 30- or 60-second intervals to limit shifts to 2 mm or less.
Patient Assessment and Follow-Up
Patients should be seen weekly throughout the course of prostate SBRT and at completion of RT. Those at higher risk for developing urinary or bowel toxicities should be appropriately counseled with all patients being seen at minimum 1 month after completion of prostate SBRT. Supportive measures for symptoms include phenazopyridine or cranberry tablets for dysuria, selective alpha receptor antagonists for obstructive symptoms, and hydrocortisone and/or antidiarrheal agents for proctitis or diarrhea. Patients should anticipate a transient worsening in bowel and urinary symptoms with resultant return to baseline approaching 3 months (129). As emphasized earlier, patients treated with prostate SBRT should be diligently followed, ideally on a clinical protocol, with stringent assessment.
261PSA Kinetics
As with EBRT, the success of prostate SBRT is defined by a combination of clinical examination and PSA response. Following definitive management, patients should be surveilled with PSA every 6 to 12 months up to 5 years and then annually as per national guidelines (19). More frequent PSA may be indicated for higher risk patients or if there is any change in trajectory. Biochemical failure should be defined as per ASTRO recommendations as nadir plus two at the time of failure (129). This being said, the expected kinetics of PSA after prostate SBRT appears to be noticeably different than that seen with brachytherapy or EBRT.
The PSA nadir following RT has been shown to predict for bPFS and overall survival (130–133). For example, Ray et al. revealed that nadir PSA less than 0.5 resulted in significantly lower rates of bPFS (8-year, 75% vs. 17%–52%) and distant metastasis-free survival (8-year, 97% vs. 73%–96%) (130). Furthermore, the rate of PSA decline may also predict for outcomes, where a shorter time to nadir and PSA at 1 year (<0.5–2) led to lower bPFS and distant metastasis rates (130, 134, 135). Although the use of conventionally fractionated IMRT and prostate SBRT have not been directly compared, assessment of PSA kinetics suggests superiority of prostate SBRT in this regard (136–138). In a small comparative study of 69 patients, Lee et al. demonstrated lower PSA nadir values with prostate SBRT (0.37 vs. 0.23, p = .011) and greater slope of decline over time (136). Kishan et al. compared 439 patients treated with various RT techniques, showing that more patients achieved a nadir PSA less than 0.5 with prostate SBRT or HDR brachytherapy as opposed to with IMRT (75.9%–76.2% vs. 44.9%, p < .001) (137). Similar to that seen by Kim et al. and Anwar et al. in a separate study, the decay rate of PSA was markedly greater with SBRT or HDR brachytherapy with a continued decline beyond 1,000 days after treatment (138). In conjunction with data correlating PSA response to outcomes, these findings would infer equivalent if not superior efficacy of prostate SBRT.
A phenomenon well known following RT is PSA bounce, defined as a rise in PSA greater or equal to 0.2 ng/mL with a resultant decline (139). This biochemical occurrence is poorly understood, theorized to represent delayed prostatic inflammation or transition to cell kill following sublethal damage (140–142). Coincidentally, studies evaluating PSA bounce have correlated this occurrence as a predictor for improved bPFS (142–144). Contrary to temporal and nadir trends in PSA response between modalities, prostate SBRT appears to have comparable rates of PSA bounce (12%–36.2%) to IMRT and HDR (25, 137, 138, 145–147). The median time to PSA bounce and median PSA rise following prostate SBRT is generally 9 to 36 months and 0.50 to 0.55 ng/mL (25, 137, 145, 146). These findings should help to ease patient anxiety that may occur with such transient rises in PSA following RT, even after achieving a PSA nadir.
TOXICITIES
Comparison of Modalities
Although no randomized comparison exists between RT modalities, several quality of life and retrospective analyses have been published in an attempt to define differences specific to conventional IMRT and prostate SBRT (148, 149). Yu et al. evaluated Medicare claims between prostate SBRT and IMRT, identifying higher rates of GU toxicities at 6 months (12.6% vs. 15.6%, p = .009) and 2 years (36.3% vs. 43.9%, p = .001) with prostate SBRT (148). These results were, however, largely hypothesis-generating, limited by inability to account for baseline function, treatment plan quality, fractionation schemes, prostate volume, or concurrent medications including anticoagulants. The authors could not account for differences in grade of toxicities. In response, the Prostate Cancer Outcomes and Satisfaction With Treatment Quality Assessment (PROSTQA) Study Consortium published a large retrospective analysis of 803 patients treated with IMRT, brachytherapy, or prostate SBRT to assess trends in patient-reported quality of life (EPIC-26) surveys (149). Prostate SBRT had lower rates of minimal clinically detectable differences in sexual and bowel symptoms. Compared with brachytherapy, prostate SBRT had lower domain score changes from baseline with urinary irritation or obstruction compared with brachytherapy. In the absence of randomized, comparative data and in contrast to the claims data analysis by Yu et al., these data allude to lower bowel symptoms following prostate SBRT over alternative RT modalities and less urinary symptoms compared with brachytherapy.
Urinary Toxicities
Acute Toxicity
Acute grades 1 to 2 urinary symptoms are expected following prostate SBRT, typically returning to baseline at approximately 3 months following treatment (128). With exceedingly low rates of acute grades 3 to 4 urinary toxicities after prostate SBRT, patient-reported measures such as the EPIC-26 survey may 262be more relevant measures of treatment toxicity. The clinically meaningful impact of a decline in quality of life survey scores is defined by the minimally important difference (MID), which is one-half of the standard deviation from baseline (150). In a pooled quality of life analysis, Evans et al. demonstrated that domain scores in urinary irritation and incontinence were significantly lower 1 month after prostate SBRT with mean differences of –10.43 ± 14.64 (MID in 69% of patients) and –5.19 ± 12.36 (MID in 32% of patients), respectively (149). At 3 months, although mean scores in these categories were lower and a proportion still had a MID (32%–36%), these were not significantly different from baseline. Use of an alpha-blocker urinary medication appeared similar between baseline (39%) and at 6 months (44%), such that improvement in urinary domain scores was not confounded by an increase in medication use. King et al. have shown similar findings, with patients having a mean EPIC-26 urinary domain score decline of 8.7 at 1 to 3 months compared with baseline with recovery at 6 months and suggestion of improvement beyond 3 years (128). This trend was similar in patients regardless of baseline urinary symptoms.
Patients developing acute obstructive urinary symptoms including urgency, frequency, nocturia, weakened stream, and intermittency can be managed with alpha-adrenergic antagonists (tamsulosin, terazosin), 5-alpha-reductase inhibitors (dutasteride, finasteride), and/or phosphodiesterase-5 inhibitors (tadalafil). Urinary frequency and retention symptoms are the most commonly reported (151). Repka et al. evaluated the prophylactic use of an alpha-adrenergic antagonist along with urethral dose reduction (maximum point dose <110%), showing smaller increases in urinary domain scores compared with prior literature (151). In a Canadian randomized phase II study, patients treated once a week (over 29 days) compared with those treated every other day (11 days) had lower rates of acute grade 1 (41% vs. 64%) and grade 2 (11% vs. 18%) urinary toxicities (p < .01) (152). Both of these approaches may be reasonable considerations to reduce the risk of acute, mainly grades 1 to 2, urinary toxicities with prostate SBRT.
Late Toxicity
Long-term grades 3 to 4 urinary toxicities are exceptionally rare (0%–6%) in existing literature. Prospective quality of life studies have shown return to baseline of urinary symptoms within 6 to 12 months with prostate SBRT (35, 149). Continued use of urinary medications such as alpha-adrenergic blockers appears to be similar to prior to treatment (149). The incidence of late grade 2 GU symptoms after conventionally fractionated RT and brachytherapy ranges from 27% to 44% and 16% to 24%, respectively, with variations on the basis of the grading system used (11, 12, 14, 153). Likewise, in a large series following prostate SBRT with median follow-up of 5 years or more, rates of late grade 2 GU toxicities appear similar (7%–21%) if not lower (29, 31, 38, 81).
Dose heterogeneity, bladder dose, prostatic urethral dose, and prostate gland size have all been correlated with a higher risk of late GU toxicities, many of these able to be corrected with careful treatment planning (30, 79, 80, 113, 146). Two studies have correlated homogeneity index, particularly the volume of prostate receiving 120%, with higher rates of grade ≥2 late GU toxicities (80, 113). Previously outlined in this chapter are the data regarding prostate size, with a suggestion of 50 to 60 mL as a sufficient cutoff to predict the risk of GU toxicities, mainly obstructive symptoms (79, 80, 145). Sparing of the prostatic urethra using various parameters with ultimate intent to reduce the maximum point dose has been shown to correlate to late GU toxicities and urinary quality of life survey scores (30, 79, 80). Fractionation schemes using longer treatment times (every other day fractions) have further reduced the risk of late grades 1 to 2 GU toxicities (35). The appropriate dose fractionation scheme has been reviewed earlier (35, 111, 112).
Although overall studies have shown return to baseline of urinary quality of life domains in the long term after prostate SBRT, a proportion (13.4%) of patients may still have a delayed flare of urinary symptoms (77, 147, 149). This consists of an increase in obstructive voiding symptoms such as frequency, urgency, dysuria, and weakened stream approximately 9 to 18 months after SBRT. As shown in brachytherapy literature, patients with this late flare in symptoms typically were younger and did not have any correlation with higher PSA bounce rates (77, 154). These patients are generally managed conservatively with alpha-adrenergic antagonists and, if not responsive, a short course of corticosteroids with the theory being this represents delayed inflammation.
Bowel/Rectal Toxicities
Acute Toxicity
Similar to that seen with urinary toxicities, severe (grade ≥3) GI toxicities are exceedingly uncommon with the largest incidence occurring in patients 263treated with a high-dose scheme (31, 110). Overall rates of acute grades 2 or greater GI toxicities such as bowel frequency, urgency, and proctitis range from 2% to 23% (27, 30, 32, 36, 81, 155). As seen with urinary patient-reported outcomes, an initial decline in EPIC bowel scores occurs between 1 and 3 months (mean bowel domain score –12 vs. baseline) with return to baseline around 6 months (128). Paydar et al. found this trend to be even sooner for acute proctitis, with a peak at 1 week and return to baseline at 3 months (155). Symptoms from acute rectal toxicities are best managed with supportive measures such as antidiarrheals and/or steroid suppositories.
Although acute rectal toxicity rates are low with prostate SBRT, methods that may further reduce rectal toxicities include use of a biodegradable rectal spacer and intrarectal amifostine (96, 97, 156, 157). From EBRT literature, intrarectal placement of amifostine suspension has been shown to reduce the rates of acute proctitis (156, 157). The largest downside to this approach is patient discomfort with daily insertion, which is less so an issue with the fewer treatments delivered with prostate SBRT. No direct comparisons have been made in prostate SBRT series, although some have routinely used amifostine for treatments with suggested low rates of GI toxicity (145).
Late Toxicity
Rates of severe late rectal toxicities are generally uncommon with exception of one series (grades 3–4, 6.6%) because of the use of a high-dose regimen (31, 110). In this series, all severe toxicities occurred in the highest dose arm (50 Gy in five fractions) with five of six patients requiring colostomies because of rectal ulceration, pain, and/or fistula formation. Dose correlations were found with the risk of late grades 3 to 4 rectal toxicity, most of which restricted to the high-dose volumes. These findings strongly indicate the need for careful rectal dosimetry and avoidance of extreme dose fractionation schemes. In all other reported series and in quality of life data, late rectal toxicity rates remain low with an expected return to baseline of bowel quality of life scores at 3 to 6 months (149, 155). Compared with IMRT and brachytherapy, prostate SBRT has been shown to have significantly higher bowel domain scores, suggesting lower rates of long-term patient-reported toxicities (149).
Rectal bleeding can occur following RT because of proximity of the anterior rectal wall to the prostate gland. Sood et al. evaluated endoscopic findings after prostate monotherapy or boost SBRT using the Vienna Rectoscopy Score (VRS) (158). Ten patients (20%) had telangiectasias on endoscopy, mostly nonconfluent defined as VRS grade 2. Nonetheless, the crude rate of symptomatic rectal bleeding requiring minor cauterization was 1.9%. Musunuru et al. reported on 19% with high-grade hematochezia after prostate SBRT at a median time of 11.7 months from the start of treatment (159). Patients receiving higher doses with larger PTV margins or treatment to the seminal vesicles had higher rates of hematochezia at 2 years: 4.9% (35 Gy, prostate with 4-mm margin), 27.2% (40 Gy, prostate with 5-mm margin), and 42.1% (40 Gy, prostate/seminal vesicles with 5-mm margin). As expected, this reflected the rectal volume receiving a high dose (V38 ≥2 cm3: odds ratio [OR]: 4.7, p = .0001). Other predictive factors included prior history of hemorrhoids (OR: 2.7, p = .0203) and use of anticoagulants (OR: 6.5, p = .0027). Methods of rectal dose sparing, protracted fractionation, and cytoprotective agents may be most critical in these patients.
Sexual Function Toxicity
Decline in patient-reported sexual function is commonly reported after RT, although it is confounded often by ADT and expected effects of aging. Unlike EPIC domain scores for urinary and bowel function, sexual domain scores can continue to decline after SBRT (149). Even after selecting only patients with good baseline sexual function, Evans et al. showed lower rates of twofold MID changes in sexual function at 2 years with prostate SBRT (20%) compared to those with IMRT (33%) and brachytherapy (35%). The mean sexual domain score decline was significantly less with prostate SBRT (–14 vs. –20.6 to –23.6, p < .01), despite brachytherapy patients being younger. However, on multivariable analysis, this difference was not statistically significant. Other series have confirmed continued decline in sexual potency with increasing need for medication support (160, 161). Use of MRI-based planning may enable sparing of the neurovascular bundle akin to nerve-sparing prostatectomy, although this has not been correlated with outcomes as of yet. Therefore, patients being treated with prostate SBRT should be counseled that much like alternative forms of RT and as expected with aging, erectile dysfunction can transpire with time after treatment.
264CONCLUSIONS
The greatest limitation of prostate SBRT presently is the lack of long-term prospective data. Maturation of existing retrospective and prospective data, as approaching the 10-year median follow-up mark, should provide further reassurance of the role of prostate SBRT as monotherapy for low- and favorable intermediate-risk patients. Several randomized trials are attempting to directly compare conventionally or moderately hypofractionated RT with prostate SBRT (Table 16.6). The Prostate Advances in Comparative Evidence (PACE) trial is a unique, ongoing randomized trial evaluating prostate SBRT to IMRT and also to radical prostatectomy for organ-confined, low- or intermediate-risk disease (NCT01584258). Sponsored by the Proton Cooperative Group, a randomized phase III trial (NCT01230866) is accruing patients to compare conventional fractionated proton RT with extreme hypofractionation (38 Gy in five fractions). A similar trial is being run at the University of Miami using photon IMRT (NCT01794403) and a Hong Kong group (NCT02339701). RTOG 0938, a French study, and the Scandinavian Hypofractionated Radiotherapy of Intermediate Risk Localized Prostate Cancer (HYPO-RT-PC) trial are comparing moderate and extreme hypofractionation (ISRCTN45905321, NCT01434290, NCT02361515). The Lyon group is also integrating injection of a hyaluronic acid as a rectal spacer to reduce rectal toxicity. Ultimate accrual and report from these trials will better define the appropriate fractionation and treatment approach for these patients.
An alternative approach for low-risk prostate cancer in the era of multiparametric MRI is the use of focal therapy. Focal brachytherapy results have been reported in early form using MRI-based planning to achieve similar efficacy to whole gland irradiation while improving urethral and rectal sparing (162, 163). Aluwini et al. reported early results of prostate SBRT using a simultaneous integrated boost (44 Gy in four fractions) to the gross disease if identified by MRI (26). Only 28% had an identifiable dominant lesion, with 3 of 14 patients having multiple lesions. Results from this series appeared comparable to other prostate SBRT series. The Hypo-FLAME (Hypofractionated Focal Lesion Ablative Microboost in prostatE Cancer) study (NCT02853110) is an ongoing phase II study aiming to assess the impact of a similar approach, delivering 50 Gy in five fractions to an identified nodule by MRI.
The role of prostate SBRT among high-risk and oligometastatic patients has yet to be well established. Traditionally, high-risk patients receive ADT with dose-escalated RT on the basis of an overall survival benefit (49, 50). However, the role of ADT with prostate SBRT is less clear. Early 265results of the FASTR (Fairly Brief Androgen Suppression and Stereotactic Radiotherapy for High Risk Prostate Cancer) trial have shown feasibility of an extreme hypofractionated approach along with ADT and simultaneous coverage of a lower dose to the pelvic nodes (164). FASTR-2 (NCT02229734), an accruing follow-up study, has prolonged ADT length to 18 months while excluding coverage of the pelvic nodes. With improvements in the management of metastatic disease, several studies have now suggested a benefit to cytoreductive definitive prostate management in select patients (165, 166). If definitive management indeed plays a future role in these patients, prostate SBRT provides the appeal of being delivered in a shorter, noninvasive, and cost-effective treatment course. Two trials (NCT02206724, NCT02563691) are currently evaluating the safety and efficacy of prostate SBRT in this setting.
REFERENCES
1. Torre LA, Siegel RL, Ward EM, et al. Global cancer incidence and mortality rates and trends: an update. Cancer Epidemiol Biomarkers Prev. 2016;25(1):16-27. doi: 10.1158/1055-9965.EPI-15-0578
2. Howlader N, Noone AM, Krapcho M, et al. (2016, April). SEER cancer statistics review, 1975-2013, National Cancer Institute. Bethesda, MD. Available at: http://seer.cancer.gov/csr/1975_2013/, based on November 2015 SEER data submission, posted to the SEER web site.
3. Michalski JM, Moughan J, Purdy JA, et al. Initial results of a phase 3 randomized study of high dose 3DCRT/IMRT versus standard dose 3D-CRT/IMRT in patients treated for localized prostate cancer (RTOG 0126). Int J Radiat Oncol Biol Phys. 2014;90(5):1263. doi: 10.1016/j.ijrobp.2014.10.035
4. Al-Mamgani A, Heemsbergen WD, Levendag PC, et al. Subgroup analysis of patients with localized prostate cancer treated within the Dutch-randomized dose escalation trial. Radiother Oncol. 2010;96(1):13-18. doi: 10.1016/j.radonc.2010.02.022
5. Zietman AL, Bae K, Slater JD, et al. Randomized trial comparing conventional-dose with high-dose conformal radiation therapy in early-stage adenocarcinoma of the prostate: long-term results from Proton Radiation Oncology Group/American College of Radiology 95-09. J Clin Oncol. 2010;28(7):1106-1111. doi: 10.1200/JCO.2009.25.8475
6. Kuban DA, Tucker SL, Dong L, et al. Long-term results of the M. D. Anderson randomized dose-escalation trial for prostate cancer. Int J Radiat Oncol Biol Phys. 2008;70(1):67-74. doi: 10.1016/j.ijrobp.2007.06.054
7. Michalski JM, Yan Y, Watkins-Bruner D, et al. Preliminary analysis of 3D-CRT vs. IMRT on the high dose arm of the RTOG 0126 prostate cancer trial: toxicity report. Int J Radiat Oncol Biol Phys. 2011;81(2):S1-S2. doi: 10.1016/j.ijrobp.2011.06.004
8. Fowler J, Chappell R, Ritter M. Is alpha/beta for prostate tumors really low? Int J Radiat Oncol Biol Phys. 2001;50(4):1021-1031. PubMed PMID: 11429230.
9. Brenner DJ, Martinez AA, Edmundson GK, et al. Direct evidence that prostate tumors show high sensitivity to fractionation (low alpha/beta ratio), similar to late-responding normal tissue. Int J Radiat Oncol Biol Phys. 2002;52(1):6-13. PubMed PMID: 11777617.
10. Miralbell R, Roberts SA, Zubizarreta E, et al. Dose-fractionation sensitivity of prostate cancer deduced from radiotherapy outcomes of 5,969 patients in seven international institutional datasets: α/β = 1.4 (0.9-2.2) Gy. Int J Radiat Oncol Biol Phys. 2012;82(1):e17-e24. doi: 10.1016/j.ijrobp.2010.10.075
11. Pollack A, Walker G, Horwitz EM, et al. Randomized trial of hypofractionated external-beam radiotherapy for prostate cancer. J Clin Oncol. 2013;31(31):3860-3868. doi: 10.1200/JCO.2013.51.1972
12. Incrocci L, Wortel RC, Alemayehu WG, et al. Hypofractionated versus conventionally fractionated radiotherapy for patients with localised prostate cancer (HYPRO): final efficacy results from a randomised, multicenter, open-label, phase 3 trial. Lancet Oncol. 2016;17(8):1061-1069. doi: 10.1016/S1470-2045(16)30070-5
13. Dearnaley D, Syndikus I, Mossop H, et al. Conventional versus hypofractionated high-dose intensity-modulated radiotherapy for prostate cancer: 5-year outcomes of the randomised, non-inferiority, phase 3 CHHiP trial. Lancet Oncol. 2016;17(8):1047-1060. doi: 10.1016/S1470-2045(16)30102-4
14. Lee WR, Dignam JJ, Amin MB, et al. Randomized phase III noninferiority study comparing two radiotherapy fractionation schedules in patients with low-risk prostate cancer. J Clin Oncol. 2016;34(20):2325-2332. doi: 10.1200/JCO.2016.67.0448
15. Kupelian PA, Potters L, Khuntia D, et al. Radical prostatectomy, external beam radiotherapy <72 Gy, external beam radiotherapy > or =72 Gy, permanent seed implantation, or combined seeds/external beam radiotherapy for stage T1-T2 prostate cancer. Int J Radiat Oncol Biol Phys. 2004;58(1): 25-33. PubMed PMID: 14697417.
16. Hamdy FC, Donovan JL, Lane JA, et al. 10-year outcomes after monitoring, surgery, or radiotherapy for localized prostate cancer. N Engl J Med. 2016;375(15):1415-1424. doi: 10.1056/NEJMoa1606220
17. Klotz L, Vesprini D, Sethukavalan P, et al. Long-term follow-up of a large active surveillance cohort of patients with prostate cancer. J Clin Oncol. 2015;33(3):272-277. doi: 10.1200/JCO.2014.55.1192
18. Tosoian JJ, Trock BJ, Landis P, et al. Active surveillance program for prostate cancer: an update of the Johns Hopkins experience. J Clin Oncol. 2011;29(16):2185-2190. doi: 10.1200/JCO.2010.32.8112
19. Mohler JL, Armstrong AJ, Bahnson RR, et al. Prostate cancer, version 1.2016. J Natl Compr Canc Netw. 2016;14(1):19-30. PubMed PMID: 26733552.
20. Nguyen PL, Gu X, Lipsitz SR, et al. Cost implications of the rapid adoption of newer technologies for treating prostate cancer. J Clin Oncol. 2011;29(12):1517-1524. doi: 10.1200/JCO.2010.31.1217
21. Paravati AJ, Boero IJ, Triplett DP, et al. Variation in the cost of radiation therapy among Medicare patients with cancer. J Oncol Pract. 2015;11(5):403-409. doi: 10.1200/JOP.2015.005694
22. Parthan A, Pruttivarasin N, Davies D, et al. Comparative cost-effectiveness of stereotactic body radiation therapy versus intensity-modulated and proton radiation therapy for localized prostate cancer. Front Oncol. 2012;2:81. doi: 10.3389/fonc.2012.00081
23. Hodges JC, Lotan Y, Boike TP, et al. Cost-effectiveness analysis of stereotactic body radiation therapy versus intensity-modulated radiation therapy: an emerging initial radiation treatment option for organ-confined prostate cancer. J Oncol Pract. 2012;8(suppl 3):e31s-e37s. doi: 10.1200/JOP.2012.000548
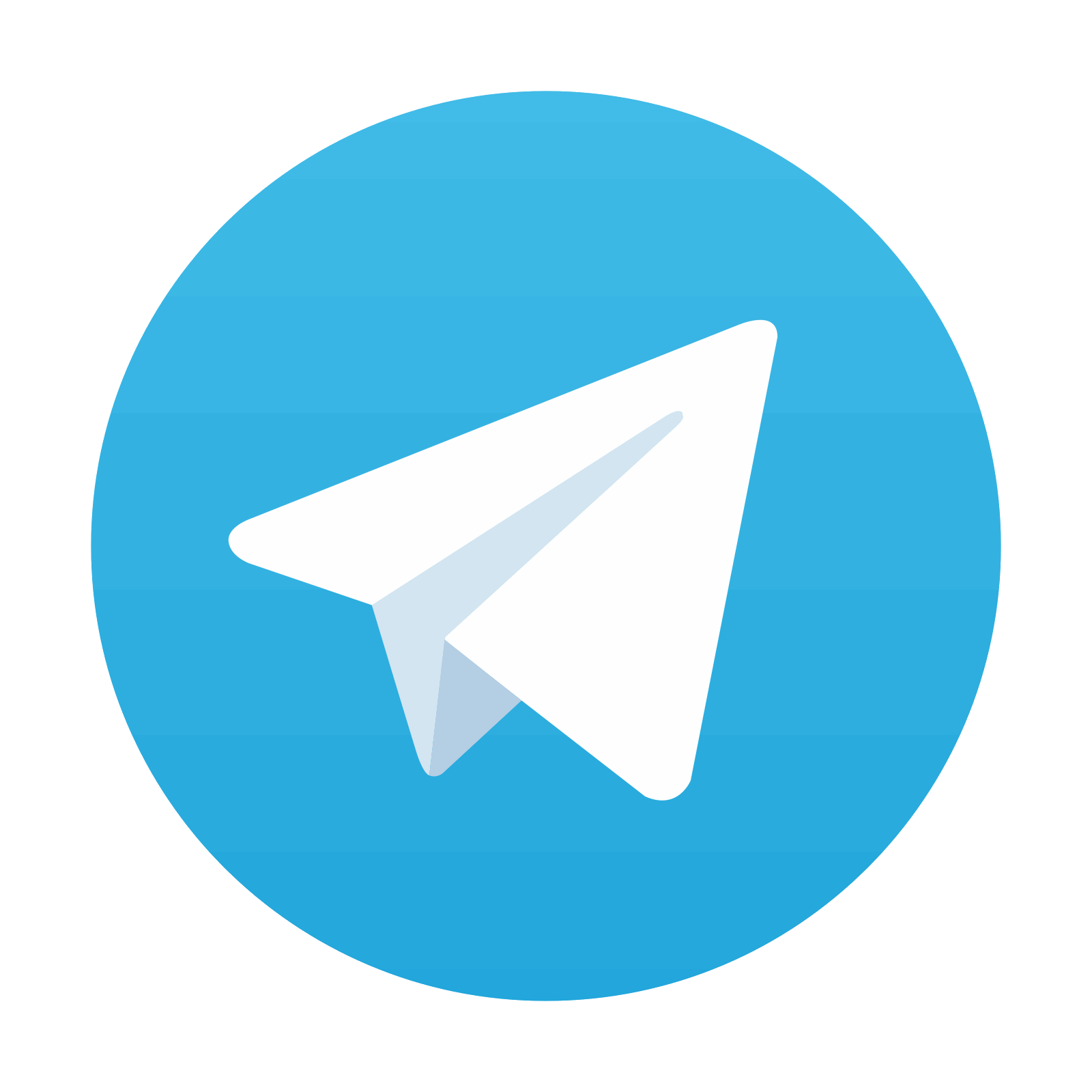
Stay updated, free articles. Join our Telegram channel

Full access? Get Clinical Tree
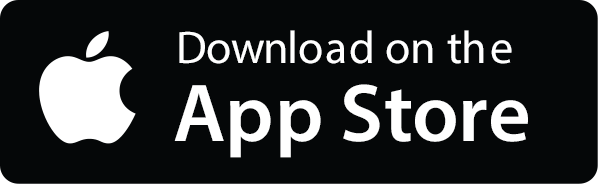
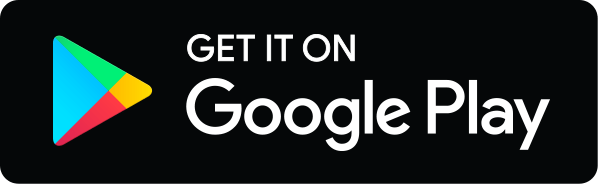