The relationships between the kidneys and the lungs are clinically important ones in health and disease. This chapter first reviews the role of the kidney in fetal lung development and the interactions between respiratory and renal function under normal conditions. Then a brief overview is provided of the large group of diseases that affect the lungs and the kidneys. Most of these conditions are uncommon in pediatric patients, although three of them—Wegener granulomatosis, systemic lupus erythematosus, and Goodpasture syndrome—may be encountered frequently by clinicians. They are discussed in detail elsewhere in this book. This chapter describes how chronic renal failure may affect respiratory function and the intrathoracic structures, and provides a brief review of the corresponding manifestations of acute renal failure and the ways in which they affect respiratory care in children. The phenomenon of dialysis-related hypoxemia is described and explained. Finally, the ways in which critical illness and its management may adversely affect kidney function are summarized.
Role of the Kidney in Fetal Lung Growth
Lung development continues during the middle trimester with branching morphogenesis and is completed postnatally with the development of alveoli. Fetal urine is an important component of the amniotic fluid during late gestation and contributes to lung growth. During fetal development, the kidney also is a major source of proline. Proline aids in the formation of collagen and mesenchyme in the lung, explaining the severe pulmonary hypoplasia secondary to prolonged or severe oligohydramnios (amniotic fluid index <4 for >2 weeks) seen in fetal renal agenesis, urinary tract obstruction, bilateral renal dysplasia, and bilateral cystic kidneys.
Physiologic Connections Between the Lungs and the Kidneys
Lung and kidney function are intimately related in health and disease. Respiratory changes help modulate the systemic effects of renal acid-base disturbances, and the reverse is also true, although renal compensation occurs more slowly than its respiratory counterpart. Under normal circumstances, the lungs and kidneys work together to maintain acid-base balance in the body, according to the relationship described by the Henderson-Hasselbalch equation
pH = pK + log ( base concentration / acid concentration )
According to this equation, the overall acidity or alkalinity of the blood, which is quantified by the negative logarithm of the hydrogen ion concentration (or pH), is determined by the relationship between the amount of base and the amount of acid present, also expressed logarithmically, as modified by a mathematical constant (pK) for the particular acid involved. The bicarbonate-carbonic acid system is the major buffering system of the extracellular fluid. Bicarbonate (HCO 3 − ) dissociates into CO 2 and water in the presence of the enzyme carbonic anhydrase, so that the acid-base quotient in the previous equation can be thought of as the HCO 3 − concentration divided by the CO 2 concentration. The CO 2 concentration is related to the partial pressure of CO 2 (PaCO 2 ) in the arterial blood by the solubility constant 0.03, so the Henderson-Hasselbalch equation can be rewritten in terms of what clinicians typically measure:
pH = 6.1 + log [ HC O 3 − concentration / ( Pac o 2 × 0.03 ) ]
Because the kidneys normally determine the concentration of the HCO 3 − , and the alveolar ventilation regulates the PaCO 2 , the relationship also can be rewritten conceptually as:
pH = pK + ( kidneys / lungs )
A decrease in HCO 3 − concentration (metabolic acidosis), whether from an increase in acids in the blood or an overall loss of HCO 3 − , provokes an increase in alveolar ventilation (respiratory alkalosis), which tends to restore the balance between the two and bring the low arterial pH (acidemia) back toward normal. This process may be thought of as respiratory compensation for metabolic acidosis. The stimulus to increase the ventilation is chiefly the action of H + ions on the peripheral chemoreceptors.
An increase in HCO 3 − concentration (metabolic alkalosis) causes an increase in arterial pH (alkalemia), which tends to decrease alveolar ventilation (respiratory acidosis). In this instance, respiratory compensation is usually less vigorous, however, because the respiratory stimulant effect of hypercapnia is much stronger than the respiratory depressant effect of alkalemia. The familiar clinical presentation of diabetic ketoacidosis is an example of respiratory compensation for severe metabolic acidosis. Patients with this disorder may hyperventilate to PaCO 2 levels of 10 mm Hg or less, which diminishes (but does not completely correct) their severe acidemia. In both instances, the respiratory changes are immediate (within a few minutes) because of the rapidity of equilibration between alveolar gas and pulmonary capillary blood.
In the less frequent situation of primary metabolic alkalosis, as is seen with protracted vomiting or the ingestion of excess alkali, patients typically present with only modest hypercapnia (e.g., PaCO 2 48 to 50 mm Hg) despite pH greater than 7.60. An increase in PaCO 2 stimulates the kidneys to retain HCO 3 − , producing metabolic alkalosis, which tends to normalize arterial pH. Conversely, hypocapnia prompts an increased loss of HCO 3 − , causing a compensatory metabolic acidosis that decreases arterial pH. The renal responses to respiratory acid-base disturbances occur much more slowly, however—over a few days—than do respiratory adjustments to metabolic disturbances. As a result, because carbonic acid/HCO 3 − buffering acts immediately, but is relatively weak, sudden alterations in respiratory acid-base status cause more sudden and severe changes in arterial pH than do their primary metabolic counterparts. An example of the more gradual adjustment of metabolic status with changes in ventilatory status is respiratory acidosis in patients with cystic fibrosis. When such patients present with a severe exacerbation, they may be severely acidemic if hypercapnia has developed rapidly, whereas the same PaCO 2 in a clinically stable patient tends to be associated with a much more normal pH ( Table 6-1 ).
Normal | Acute Respiratory Acidosis * | Chronic Respiratory Acidosis † | |
pH | 7.40 | 7.24 | 7.38 |
PaCO 2 (mm Hg) | 40 | 56 | 56 |
HCO 3 − (mEq/L) | 24 | 25 | 33 |
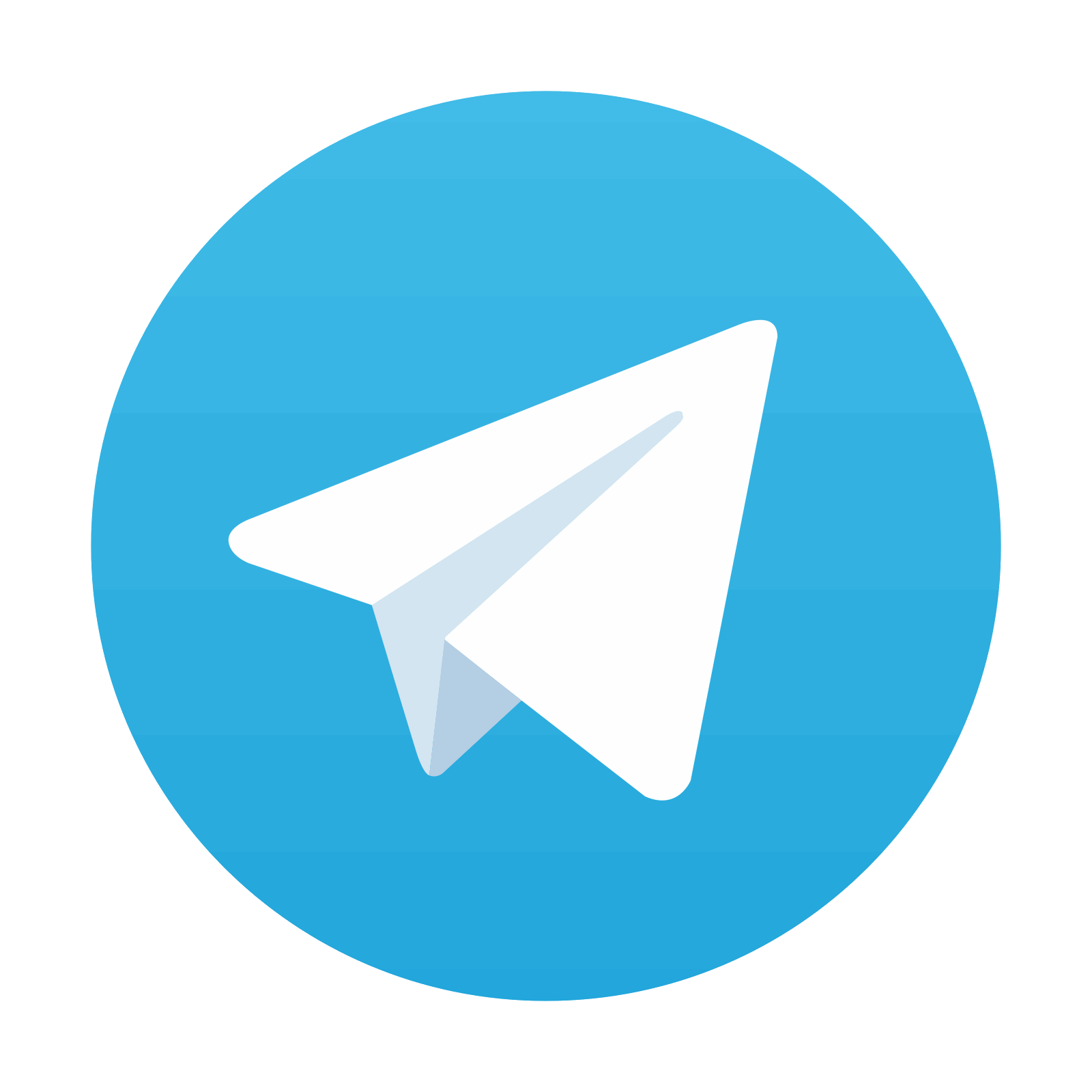
Stay updated, free articles. Join our Telegram channel

Full access? Get Clinical Tree
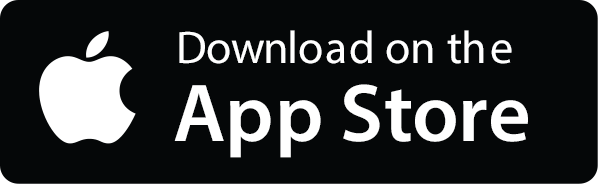
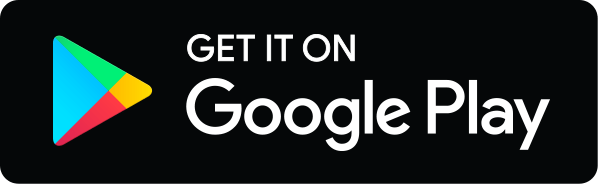
