The pulmonary veins and atria are affected by a variety of congenital and acquired abnormalities. Echocardiography is often the initial modality used in the evaluation of atria and atrial appendages. However, echocardiography is operator dependent, has limited field of view, and does not have tissue characterization capabilities. Computed tomography (CT) and magnetic resonance imaging (MRI) are increasingly used in the evaluation of atria and the atrial appendages. In addition, CT and MRI are the ideal noninvasive imaging modalities in the evaluation of pulmonary veins. Imaging plays an important role in the detection of abnormality and characterization of the lesion.
Cross-Sectional Imaging Techniques
Pulmonary veins and the atria can be evaluated both by CT and MRI. Both CT and MRI have good spatial and temporal resolution, large field-of-view capabilities, and multiplanar reconstruction capabilities. CT is faster than MRI but requires the use of intravenous contrast media, which is potentially nephrotoxic in patients with predisposing conditions and is associated with a small but not insignificant risk for cancer because of ionizing radiation. MRI is not associated with radiation. MRI cannot be performed in patients with claustrophobia or those with contraindications such as metallic implants or pacemakers. Gadolinium-based contrast agents used in MRI are not nephrotoxic but can induce debilitating nephrogenic systemic fibrosis in patients with severe renal dysfunction. However, even in these patients angiography can be performed using the three-dimensional (3-D) whole heart steady-state free precession (SSFP) sequence.
Pulmonary veins and left atria are commonly imaged before and following radiofrequency ablation of the pulmonary venous ostia for atrial fibrillation. CT images are acquired at end expiration to enable mapping in the electrophysiology laboratory. Electrocardiogram (ECG) gating is preferred to avoid motion artifacts. In patients with low and stable heart rhythm, prospective ECG triggering can be performed to reduce the radiation dose. In patients with high heart rates and in large patients, retrospective ECG gating may be required. Non–ECG-gated helical scans are used in patients with atrial fibrillation and high heart rates. Non–ECG-gated technique has the least radiation dose of all the techniques available. Motion artifacts with this technique are trivial and do not compromise the ability to make a diagnosis. Pulmonary veins are typically imaged in the late systolic phase, when they have the largest caliber. Injection of 75 to 90 mL of nonionic iodinated contrast through an antecubital vein at the rate of 3.5 to 4.5 mL/second using a power injector is followed by 50 mL of normal saline to flush out the superior vena cava (SVC) to avoid obscuring the right superior pulmonary vein. Multiplanar reconstruction, volume rendering, shaded surface display, and endocardial (intraatrial) reconstructions are the postprocessing techniques used for evaluation of the left atrium and pulmonary veins.
MRI evaluation of the atria and pulmonary veins requires a combination of various sequences in different planes. Cine images are acquired through SSFP sequence. Characterization of a mass is performed using T1- and T2-weighted black-blood fast spin echo sequences. Early postcontrast images are acquired using T1-weighted spin echo sequence, and delayed postcontrast images are acquired using inversion recovery sequences, both following intravenous administration of 0.1 to 0.2 mmol/kg of gadolinium chelates. Evaluation of pulmonary veins can be done either using postcontrast MR angiography with T1-weighted spoiled gradient sequence or using a noncontrast whole heart 3-D SSFP sequence.
Pulmonary Veins
Pulmonary Venous Anatomy and Drainage
In 80% to 85% of the normal population, four pulmonary veins are seen draining into the left atrium, namely, the right superior, right inferior, left superior, and left inferior pulmonary veins. The right middle vein drains into the right superior pulmonary vein ( Fig. 40-1 , A ). Variations in pulmonary venous drainage are seen in 15% to 20% of the population. This includes variations in the number of ostia, the length of pulmonary veins, and branching patterns. In the conjoined pattern of drainage, the superior and inferior pulmonary veins of one side join before they drain into the left atrium, resulting in an ostium that is larger than normal ( Fig. 40-1 , B ). This is more common on the left than the right. In accessory pattern of drainage, there are accessory ostia in addition to the normal four ostia. Although any of the pulmonary segmental branches can open directly into the left atrium, the right middle lobe and right lower lobe superior segmental branches are the most common accessory veins ( Fig. 40-1 , C ). The accessory ostia are smaller than normal. Different patterns of drainage may be seen in the same individual. Occasionally a pulmonary vein drains into the pulmonary vein of the contralateral side ( Fig. 40-1 , D ).
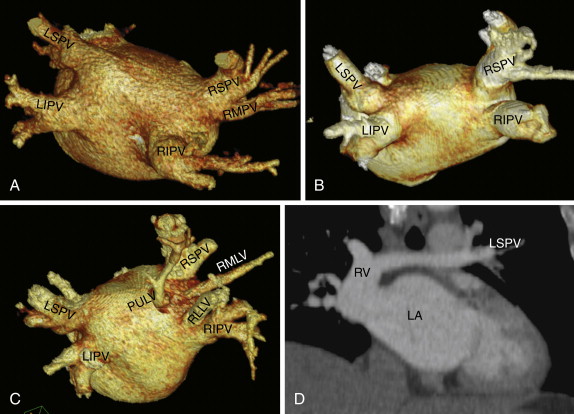
Anomalous pulmonary venous return is defined as drainage of pulmonary veins into a structure other than the left atrium. Anomalous drainage can either be partial or total. In partial anomalous pulmonary venous return (PAPVR), one to three of the pulmonary veins drain into the systemic venous circulation, either directly into the right atrium or into systemic veins, resulting in a partial left-to-right shunt. This is seen in 0.7% of the population and is usually asymptomatic. The left superior pulmonary vein is the most common vein to have anomalous drainage (47% of PAPVR cases), most commonly into the left brachiocephalic vein ( Fig. 40-2 , A ) , followed by right superior pulmonary vein draining into the SVC ( Fig. 40-2 , B ). Anomalous drainage of the right inferior and the left inferior pulmonary veins is less common. The anomalous pulmonary vein may also drain into the coronary sinus, azygos vein, inferior vena cava (IVC), and very rarely into the right atrium. Sinus venosus defect is seen in 42% of patients with right upper lobe PAPVR. In the scimitar syndrome, there is anomalous pulmonary venous drainage of part of or the entire lung into the IVC, coronary sinus, right atrium, azygos vein, portal vein, or hepatic vein. This is almost always seen on the right side and associated with hypoplasia of the right lung and right pulmonary artery. The anomalous pulmonary vein is seen in the shape of a scimitar, curving medially toward the diaphragm and the IVC in posteroanterior chest radiographs and coronal CT or MRI.
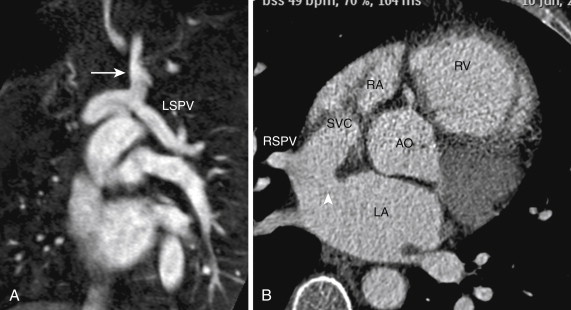
In total anomalous pulmonary venous return (TAPVR), all of the pulmonary veins drain directly into the right atrium or into a systemic venous channel that eventually drains into the right atrium. TAPVR is classified as supracardiac (typically a vertical vein draining into the brachiocephalic vein), cardiac (drainage directly into the right atrium or coronary sinus), or infradiaphragmatic (usually via the portal vein). Occasionally connections may exist at two or more levels. Obstruction to the pulmonary veins is seen most commonly in the infradiaphragmatic type, as the anomalous vein enters the portal vein, which has higher venous pressure than the right or left atrium and their tributaries.
Knowledge of variations in pulmonary venous drainage becomes crucial in patients being evaluated for radiofrequency ablation of pulmonary venous ostia for atrial fibrillation. Variations can be seen in the number of ostia, branching pattern, and length of branches. Because of the small size, accessory ostia may be easily missed during ablation procedures, resulting in recurrent atrial fibrillation caused by incomplete ablation of arrhythmogenic foci. Another variation to be recognized is the presence of an early or ostial branch that occurs within 5 mm of the pulmonary venous ostia. This is most common in the right middle vein and has a high risk for the development of pulmonary stenosis following ablation. In the electrophysiology laboratory, 3-D reconstructions from CT or MRI angiographic images are typically integrated with electrophysiology data acquired from left atrium mapping catheters to establish a road map for catheter ablation procedures, including anatomic variations. Endocardial views also help in determining the presence of small accessory ostia.
Pulmonary venous stenosis is a well-recognized complication of ablation procedures for atrial fibrillation, seen in 1% to 3% of these procedures. Stenosis is caused by intimal thickening, thrombus, endocardial contraction, and elastic laminae proliferation. Symptoms are usually seen 2 to 5 months after the procedure, with the intensity related to the degree of obstruction. CT or MRI is used in the evaluation of pulmonary stenosis. With MRI, postcontrast MR angiography or noncontrast MR angiography using whole heart 3-D SSFP sequence can be used. Based on the severity of the luminal narrowing, pulmonary venous stenosis can be graded as mild (<50% narrowing), moderate (50% to 69% narrowing), or severe (>70% narrowing) ( Fig. 40-3 , A ). The veins are measured in short-axis images obtained using double axial reconstruction of two orthogonal images. Severe pulmonary vein stenosis is treated with balloon angioplasty with stent placement. CT is useful in the evaluation of patency of the stent ( Fig. 40-3 , B ) and further development of complications.
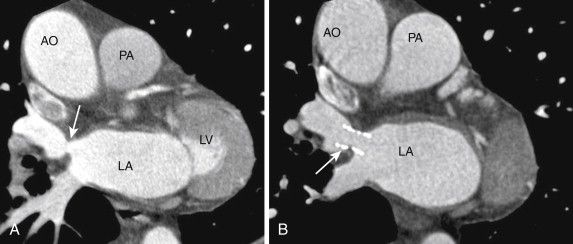
Atria and the Appendages
In normal individuals, the morphologic right and left atrium are located on the right and left sides, respectively. The right atrium receives the coronary sinus, the SVC, and the IVC, whereas the left atrium receives the pulmonary veins. The right atrium opens into the right ventricle through the tricuspid valve, and the left atrium opens into the left ventricle through the mitral valve. The atria are smooth-walled chambers and are derived from outgrowth of pulmonary veins and sinus venosus. The morphologic atria can be distinguished based on the appearance of the atrial appendages.
Atrial appendages are trabeculated, muscular outpouchings from the atrial chambers, which are remnants of the original embryonic atria. The right atrial appendage has a broad and triangular shape and has a wide junction with the right atrium. The left atrial appendage has a long, tubular, hook shape with crenellations and has a narrow junction with the left atrium. The atrial appendages are trabeculated, with muscle fibers oriented parallel to each other in the shape of a comb, called pectinate muscles, which are more pronounced in the right than the left. The atrial appendages are located within the confines of the pericardium and have only minimal useful function. The left atrial appendage is believed to act as a decompression chamber during left ventricular systole and when the left atrial pressure is elevated.
Accessory appendages or diverticula may be seen in the left atrium. An accessory appendage has ostium, body, and neck with an irregular contour because of pectinate muscle, more common in the left posterolateral wall. The accessory appendage may fibrillate independent of the rest of the heart and should be included in radiofrequency ablation. It can be a source of thromboemboli. A diverticulum has a saclike appearance with broad-based ostium and smooth contour. Diverticulum is either a remnant of an accessory pulmonary vein or a varix at the distal end of a small accessory pulmonary vein. Diverticulum may cause mitral regurgitation, arrhythmias, thromboembolism, or pericarditis. In juxtaposition with atrial appendages, both atrial appendages lie on one side of the great arteries. Diverticulum is associated with D-transposition of great arteries.
Atrial Size
Atrial measurements can be made in either CT or MRI. Atrial diameter and area are measured in the four-chamber images, usually in the end-systolic phase. Atrial volume can be measured using the area-length biplane or the prolate ellipsoid methods, both of which are less accurate than the modified Simpson method. Normal reference values have been established for atrial diameter, area, and volume. The normal atrial diameter is less than 4.1 cm in males and 3.9 cm in females. The normal atrial area is 20 cm², and the normal volume is 59 mL in males and 53 mL in females. Volumes measured by CT have been noted to be higher than those measured by MRI. The atrial size varies with body surface area and less with age and sex. Based on area, 21 to 30 cm² is considered mild atrial enlargement, 31 to 40 cm² is considered moderate atrial enlargement, and greater than 40 cm² is considered severe atrial enlargement.
Left atrial enlargement is most commonly seen in mitral stenosis. Other causes include volume overload (mitral regurgitation, atrial septal defect with either shunt reversal or tricuspid atresia, ventricular septal defect, patent ductus arteriosus, aortopulmonary window); pressure overload (noncompliant left ventricle [hypertrophic cardiomyopathy, hypertension, aortic stenosis], left ventricular dysfunction/failure, tumors); and miscellaneous (atrial fibrillation, idiopathic) ( Fig. 40-4 , A ). Left atrial enlargement has been shown to be an indicator of the duration and severity of diastolic dysfunction and is a predictor of adverse cardiovascular outcomes such as atrial fibrillation, congestive cardiac failure, stroke, and cardiovascular death. It is a risk factor for atrial fibrillation, stroke, and atrial fibrillation following therapy. Aneurysm of the left atrium is rare and can be congenital or result from rheumatic heart disease, tuberculosis, or syphilis. It most commonly involves the left atrial appendage. It is associated with pericardial defect and leads to mitral regurgitation, heart failure caused by pulmonary venous obstruction, respiratory distress, cardiac tamponade, thromboembolization, and arrhythmias. Small left atrium is seen in conditions with decreased pulmonary venous return such as hypoplastic left heart syndrome, TAPVR, and severe pulmonary venous stenosis.
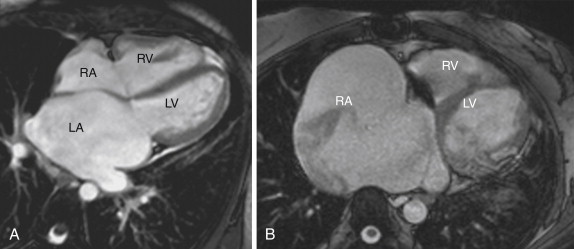
Right atrial enlargement is seen because of either volume overload (tricuspid regurgitation, atrial septal defect, atrioventricular canal defect, anomalous pulmonary venous return, pulmonary hypertension, sinus of Valsalva fistula) or pressure overload (right ventricular failure, tricuspid stenosis, tricuspid atresia, restrictive cardiomyopathy, myxoma) ( Fig. 40-4 , B ). Cardiac failure, Ebstein anomaly, arrhythmogenic right ventricular dysplasia, and endocardial fibroelastosis are some of the causes of tricuspid regurgitation. Right atrial enlargement is usually accompanied by right ventricular enlargement, except in tricuspid atresia and Ebstein anomaly. Giant right atrium without underlying cardiac cause is rarely seen. It is usually asymptomatic but may be associated with arrhythmias or thromboembolism. Fontan operation is another cause of a giant right atrium.
Left atrial calcification may be seen in the endocardial and subendocardial layers as a sequel to chronic mitral disease, atrial fibrillation, or congestive cardiac failure. It may involve the left atrial appendage in mitral stenosis (type A), free wall and mitral valve in advanced mitral stenosis (type B), or the posterior wall in mitral regurgitation (type C).
Congenital Abnormalities
Congenital abnormalities of the atria include hypoplasia, dextrocardia, transposition of great arteries, cor triatriatum, and atrial septal defects.
In dextrocardia with situs solitus the cardiac apex is located on the right, the morphologic left atrium is located to the left of the morphologic right atrium, with preservation of the atrioventricular concordance (i.e., right atrium opens into right ventricle, left atrium opens into left ventricle). In dextrocardia with situs inversus the morphologic left atrium is located to the right of the morphologic right atrium, and there is atrioventricular concordance ( Fig. 40-5 ). In situs ambiguus with left isomerism, bilateral morphologic left atrium is seen. In situs ambiguus with right isomerism, bilateral morphologic right atrium is seen. In D-transposition of great arteries there is atrioventricular concordance (right atrium opening into right ventricle, left atrium opening into left atrium), but there is ventriculoarterial discordance (left ventricle opening into pulmonary artery, right ventricle opening into aorta), as a result of which a parallel circulation is seen. In L-transposition (congenitally corrected transposition), there is both ventriculoarterial and atrioventricular discordance (right atrium opening into left ventricle, left atrium opening into right ventricle).
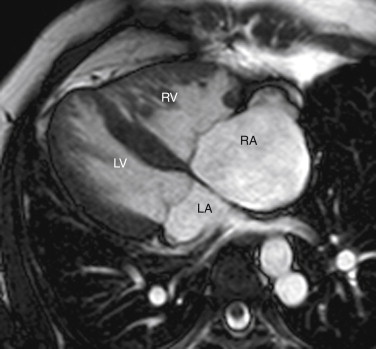
Hypoplasia of the left atrium is seen in hypoplastic left heart syndrome, which is caused by diminished flow through the left heart structures during development. The left ventricle is small and nonfunctional, along with atresia of the aortic and mitral valves. The left atrium is either small or normal. The aortic arch is hypoplastic or has coarctation. Atrial septal defect is present, but the ventricular septum is usually intact. Hypoplasia of the right atrium is seen in hypoplastic right heart syndrome, in which there is pulmonary valve atresia, small right ventricle, small tricuspid valve, and small hypoplastic pulmonary artery. The right atrium is either small or normal in size.
Cor triatriatum sinister is a congenital anomaly in which a fibromuscular membrane divides the left atrium into a proximal posterosuperior chamber and a distal anteroinferior chamber ( Fig. 40-6 ). The membrane has variable size and shape, and is either imperforate or fenestrated. The pulmonary veins typically open into the proximal chamber, and the distal chamber communicates with the mitral valve and the left atrial appendage. Very rarely, pulmonary veins may open in the distal chamber. In a subtotal cor triatriatum either the right or left pulmonary veins drain into the proximal chamber. Clinical symptoms depend on the size of the ostium and associated cardiac anomalies, such as anomalous pulmonary venous drainage. Cor triatriatum should be distinguished from a supravalvar membrane because in this condition the left atrial appendage is located proximal to the dividing membrane that is attached to the atrial surface of the mitral valve or superior to mitral annulus. Cor triatriatum dexter is a similar anomaly involving the right atrium.
