Quality Assurance for Proton Therapy
Richard L. Maughan
Jonathan B. Farr
INTRODUCTION
The Role of Quality Assurance in Radiation Oncology
The aim of quality assurance (QA) within a hospital environment is to maximize the probability of cure of a patient’s disease, while minimizing the side effects of the treatment. QA within the discipline of radiation oncology is an involved process, which is continually increasing in complexity as advances in technology offer new opportunities for innovative treatment modalities, especially related to tumor targeting. The high technology nature of radiation oncology brings together an array of highly qualified and skilled personnel who work as a team to provide safe and optimal patient care. This staff includes radiation oncologists, medical physicists, radiation therapists (radiation therapy technologists), dosimetrists, nurses, administrators, and other support staff (accelerator engineers, secretaries, billing clerks), all of whom must perform their specific roles diligently, accurately, and in accordance with well-defined procedures in order to assure the quality of the patient’s treatment.
Basic QA criteria may be mandated and set by regulatory agencies at the national, state, or provincial levels. Generally, the hospital QA committee takes responsibility for implementing these regulatory requirements, but practical oversight of radiation oncology QA occurs at the departmental level. An effective departmental QA committee must include representation from all the interested parties: radiation oncologists, medical physicists, therapists, dosimetrists, nursing, engineers, and administrators. All aspects of the patient’s treatment should be monitored and written policies and procedures should be developed to define the whole process that the patient undergoes, through consultation, diagnosis, simulation, treatment planning, treatment and follow-up. Not only is it the QA committee’s responsibility to create a comprehensive QA program with written policies and procedures, but it must also develop audit methods to monitor the effectiveness of these policies and procedures and continually strive for quality improvement. Quality improvement should be actively pursued through this audit process and through the annual selection of specific QA indicators, which are identified for a detailed monitoring during a particular year.
Physical and Technical Quality Assurance for Radiation Therapy
As in any hospital department, the medical aspects of the patient’s treatment must be carefully monitored such as the decision to treat, the prescription of dose, nursing care and follow-up. In radiation oncology, the complexity of QA is increased by the highly sophisticated equipment used in the treatment of the patient. This equipment includes accelerators, treatment planning systems, record and verify systems, and radiographic imaging equipment. Hence the emphasis of this chapter is on these physical and technical aspects of the QA process particularly as they relate to proton therapy, one of the most technically advanced and complex forms of treatment available in radiation oncology.
There are many works in the literature describing the physical aspects of QA for conventional photon and electron external beam therapy in considerable detail.
The report of the American Association of Physicists in Medicine’s (AAPM) Task Group no. 40 entitled “Comprehensive QA for Radiation Oncology”1 is a major resource. The AAPM has also published a report on treatment planning system QA2 and more recently the International Atomic Energy Agency (IAEA) has also published a technical report on this topic.3 These reports on conventional therapy techniques are, however, in need of revision because of the rapid introduction and widespread usage of more complex treatment techniques, especially intensity-modulated radiation therapy (IMRT) and, more recently, image-guided radiation therapy (IGRT). With the advent of IMRT, the need for patient-specific QA procedures to assure accurate delivery of the complex segment fields has been a major challenge. The International Commission on Radiological Units and Measurements (ICRU) is currently preparing a report on IMRT, which will address some QA issues and, in the United States, the AAPM also has task groups actively involved in revising report no. 40 and preparing reports on IMRT and imaging for radiation oncology.
The report of the American Association of Physicists in Medicine’s (AAPM) Task Group no. 40 entitled “Comprehensive QA for Radiation Oncology”1 is a major resource. The AAPM has also published a report on treatment planning system QA2 and more recently the International Atomic Energy Agency (IAEA) has also published a technical report on this topic.3 These reports on conventional therapy techniques are, however, in need of revision because of the rapid introduction and widespread usage of more complex treatment techniques, especially intensity-modulated radiation therapy (IMRT) and, more recently, image-guided radiation therapy (IGRT). With the advent of IMRT, the need for patient-specific QA procedures to assure accurate delivery of the complex segment fields has been a major challenge. The International Commission on Radiological Units and Measurements (ICRU) is currently preparing a report on IMRT, which will address some QA issues and, in the United States, the AAPM also has task groups actively involved in revising report no. 40 and preparing reports on IMRT and imaging for radiation oncology.
Physical and Technical Quality Assurance for Proton Radiation Therapy
There has been relatively little published on the QA of proton therapy beams. Moyers has written a brief description of QA needs in proton therapy related to acceptance testing, commissioning, and routine (daily, weekly, monthly, and annual) QA procedures.4 Additional short contributions on different aspects of proton therapy QA can be found in the works of others.5,6
Reports by the ICRU7 and IAEA8 have addressed in detail the issue of proton dosimetry protocols to ensure accurate and uniform calibration of the dose delivered by proton therapy beams. There are discrepancies between these two protocols. This is an important part of proton beam QA but is only part of the physical and technical QA demanded by modern precision- and image-guided techniques. The ICRU, in collaboration with the IAEA, is currently finalizing a report on prescribing, recording, and reporting the dose for proton radiation therapy. This publication will not only resolve the discrepancy between the two previously published dosimetry calibration protocols but will deal with many other aspects of proton therapy, including (in addition to prescription, recording, and reporting of dose) chapters on treatment planning, uncertainties, and physical QA procedures.
In the United States, there has been a further initiative by the AAPM, which has formed a work group on particle beams. The charge of this group is to “(a) continuously review developments in radiation therapy using particle beams (excluding electrons), including treatment planning, delivery, treatment protocols, QA techniques, dosimetry, and radiobiology and (b) encourage the formation and support of task groups that will produce documents assisting the AAPM membership in matters pertaining to particle beam therapy.” The first task group proposed by this working group has planned to address “Quality Assurance in Proton Radiation Therapy.”
The anticipated widespread introduction of scanning beams in proton therapy, together with advanced simulation and on-board imaging techniques, will considerably increase the complexity of the QA procedures. The purpose of this chapter is not to offer solutions to these problems but rather to highlight the increasing complexity of the dose delivery systems and proton beam treatment techniques with the associated need for a concentrated and coherent effort to provide QA solutions.
QUALITY ASSURANCE FOR PROTON RADIATION THERAPY
The reasons for QA in proton therapy to have remained largely undocumented in the literature are easy to comprehend. Proton beam therapy has, to date, been delivered at relatively few centers throughout the world and accounts for less than 1% of the total radiotherapy treatments. At the time of writing, there are 28 centers actively treating patients with proton or heavy charged particle beams. Of these, two treat with heavy ion beams only, six have lowenergy proton beams specializing in the treatment of ocular melanomas, and seven are hospital-based facilities with rotating treatment gantries. The majority of the facilities are still based on nuclear physics research accelerators, which have been adapted for use in radiation therapy. Only one of the active proton facilities uses pencil beam scanning. Therefore, there is a wide variety of beam delivery systems using passive scattering techniques to spread the proton beam. Although the double scattering techniques for beam spreading have many common basic features, there is a wide diversity among the systems and many aspects of the QA procedures are equipment specific, which create problems in defining QA procedures in anything but the broadest terms. In conventional therapy, linear accelerators are provided by a limited number of vendors and are constructed to similar specifications. In addition, over the years and with a large number of photon Linac users (many thousand) it has been possible to develop a range of specialized QA tools which are readily, commercially available. In proton therapy, the practice has been for individual centers to develop their own, often unique, QA tools and methods. The recent increased interest in proton therapy has led to the development of several commercial proton therapy systems and will hopefully lead proton therapy in a direction similar to photon therapy, with most centers using a limited number of well-characterized systems. At the same time, however, there is a parallel trend towards the introduction of more advanced treatment delivery techniques. In particular, many vendors are actively developing pencil beam scanning systems. It is anticipated that these
will replace double scattering for many treatments because they allow for intensity-modulated proton therapy (IMPT). This technique offers the important advantage of allowing conformation of the proton dose distribution to both the distal and proximal edges of the tumor, in contrast to double scattering which provides distal edge conformation only. Another motivation for IMPT development is to reduce the need for complicated forward treatment plans such as those including patching of beams around a critical structure. Complicated plans result in complicated deliveries increasing the potential for error. Therefore, QA for scanned proton beams will be a major focus for the future including the development of specialized treatment planning systems also requiring QA.
will replace double scattering for many treatments because they allow for intensity-modulated proton therapy (IMPT). This technique offers the important advantage of allowing conformation of the proton dose distribution to both the distal and proximal edges of the tumor, in contrast to double scattering which provides distal edge conformation only. Another motivation for IMPT development is to reduce the need for complicated forward treatment plans such as those including patching of beams around a critical structure. Complicated plans result in complicated deliveries increasing the potential for error. Therefore, QA for scanned proton beams will be a major focus for the future including the development of specialized treatment planning systems also requiring QA.
The advantages of proton therapy over conventional therapy are related to the unique dose distribution characteristics of charged particle beams in depth, which result in a potential for reduced integral dose to the patient. In passive scattering, these advantages are realized by selecting the appropriate beam energy (depending on the depth of the tumor) and appropriate energy modulation (to spread out the Bragg peak over the extent of the tumor in depth). This tailoring in depth involves beam-shaping methods which are unique to proton and heavy ion therapy and are dependent on the type of accelerator used to produce the beam. Beam shaping in depth is patient-specific, as it depends on the exact location and size of the tumor, and it requires a patient-specific QA procedure to ensure that it is correctly executed. The problem here is that beam penetration requires a good knowledge of proton range in tissue which is dependent on proton energy. The methods used to adjust beam energy (i.e., beam penetration) and the energy modulation (i.e., the width of the spread-out Bragg peak [SOBP]) depend on the combination of the acceleration system and the beam delivery system used (scattering or scanning).
The beam delivery system (or nozzle) produces the treatment beam but an equally important part of the treatment is the correct positioning of the patient in the beam and verifying that positioning. In modern proton therapy facilities, this involves a sophisticated patient positioner or robotic couch with six degrees of freedom. Patient position verification utilizes orthogonal imaging systems. In future, on-board cone beam computing tomography (CBCT), similar to that now being used in conventional therapy, will be available on the gantry. Simulation involves pretreatment imaging that is critical to the treatment planning process. In a modern radiation oncology department, simulation is no longer confined to rotational x-ray units and CT simulators; magnetic resonance imaging (MRI) and positron emission tomography (PET) images are increasingly used for tumor volume definition and normal structure delineation and the use of fused images is widespread. MRI and PET/CT units are now installed in radiation oncology departments for direct use in simulation. These practices introduce further QA challenges.
Quality Assurance and Beam Delivery Systems
Most proton therapy facilities use either a cyclotron or synchrotron to accelerate the proton beam. A cyclotron produces a continuous (or steady) beam output at a fixed energy. By contrast, the synchrotron produces a pulsed output with the ability to change the beam energy from pulse to pulse.
In cyclotrons, proton beam energy changes to adjust beam penetration must be achieved by inserting a calculated tissue-equivalent thickness of material into the beam before it enters the patient, in order to shift the position of the distal edge of the Bragg peak to the appropriate position. Typically, this may be achieved in one of two ways, either by (i) introducing a patient-specific range shifter into the beam path immediately before it enters the patient or (ii) by using an energy selection system (ESS) immediately after beam extraction and before the beam is transported to the treatment nozzle. In both cases, there must be automated equipment validation or procedures for ensuring the correct energy or appropriate range shifter is applied to the beam. Beam modulation can be achieved using a rotating stepped wheel to shift the position of the Bragg peak in depth and deliver the intensity required to produce a flat topped SOBP. In some systems, a series of wheels is supplied to give a predefined SOBP width for a given beam energy and the most appropriate wheel for a particular patient’s treatment is selected and manually installed in the treatment nozzle. In another system, a limited set of automatically selectable stepped wheels is combined with a beam intensity modulation system to provide a wide range of SOBP options, with no manual selection necessary. The QA requirements for these two arrangements are clearly very different. In pencil beam scanning using a cyclotron, the required energy is set using an ESS and the dose is delivered as a series of lateral scanned fields at different depths in the tumor using a series of calculated pristine beams. The lateral scanning system may be a raster or discrete spot scanning system. The scanning pattern is determined in the treatment planning system and a well-defined QA procedure is required to monitor this.
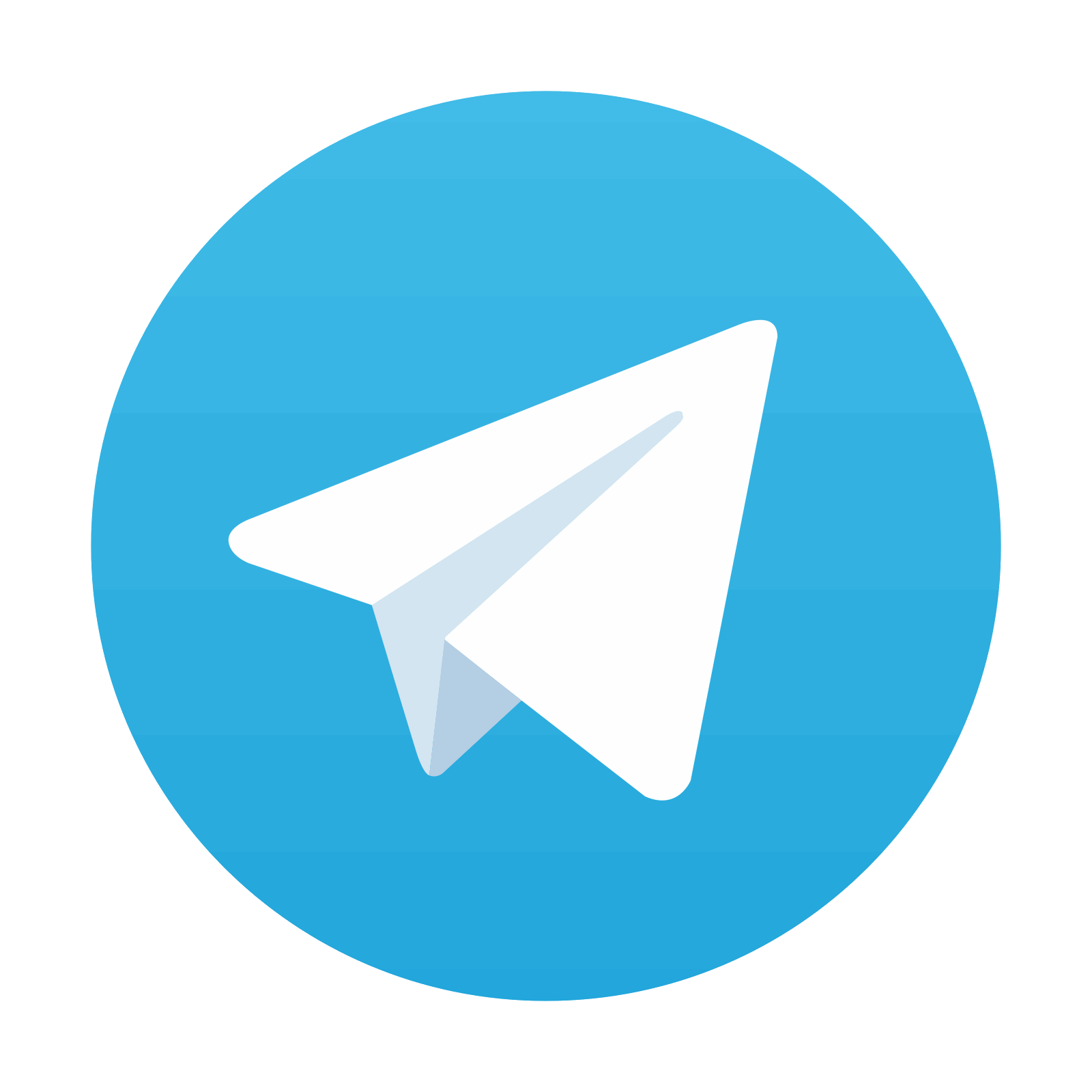
Stay updated, free articles. Join our Telegram channel

Full access? Get Clinical Tree
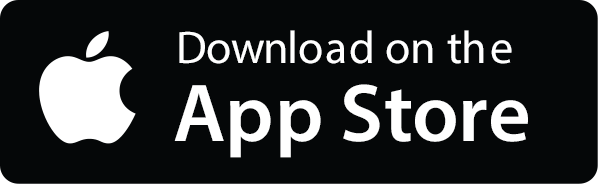
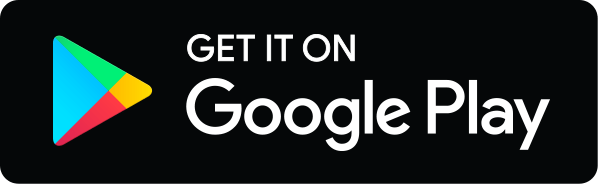