, Ahmad Ameri1 and Mona Malekzadeh2
(1)
Department of Clinical Oncology, Imam Hossein Educational Hospital, Shahid Beheshti University of Medical Sciences (SBMU), Shahid Madani Street, Tehran, Iran
(2)
Department of Radiotherapy and Oncology, Shohadaye Tajrish Educational Hospital Shahid Beheshti University of Medical Sciences (SBMU), Tehran, Iran
Radiation-induced liver disease (RILD), historically called radiation hepatitis, has been reported in 6–66% of patients whose livers are irradiated based on radiation dose, exposed liver volume, and baseline liver function [1, 2]. Abnormalities in laboratory liver function tests may be found on routine evaluation of patients when RILD is mild, but in severe cases, liver dysfunction may affect the treatment course and eventually threaten patient’s lives.
14.1 Mechanism
The histologic hallmark associated with RILD is veno-occlusive disease (VOD). It is characterized by severe congestion of the sinusoids in the central portion of the lobules with sparing of the larger veins [3].
The pathogenesis of RILD is unknown. Some possible mechanisms have been proposed. It has been postulated that radiation damages the sinusoidal and vascular endothelial cells, which initiates the coagulation cascade leading to fibrin accumulation and clot formation. The fibrin network is replaced by collagen deposition and resulted in progressive fibrous obliteration of small hepatic veins [3, 4]. Irradiation induces the dysregulated activation of myofibroblastic hepatic stellate cells, increasing some cytokine expression like TGF-β1 (transforming growth factor) and hedgehog (Hh) pathway activation (an essential pathway for tissue remodeling), [5] which are important in the development of fibrosis following exposure to radiation. GDC-0449 targets the Hh signaling pathway, and this suppressed Hh signaling may lead to reduced proliferation of progenitor cells and fibrosis in irradiated livers [5].
Finally, hepatic vessels occlude, resulting in vascular leakage, distorted dilated sinusoids, and congestion with erythrocyte trapping, causing central zone hypoxia and hepatocyte death [6].
A cluster of NF-κB-regulated cytokines, including TNF-α, are induced by radiation and increase the sensitivity of hepatocytes to radiation and induce cell apoptosis [7].
After 4 months, an effective circulatory system within the liver has apparently developed leading to the disappearance of congestion and restoration of hepatic cells [8].
14.2 Risk Factors
Radiation treatment factors have a strong correlation with RILD. Mean radiation dose to the liver and volume of liver exposed to more than 30–35 Gy of radiation are directly related to RILD occurrence [9, 10]. Emami et al. found a <5% rate of RILD when the mean whole liver dose is ≤30 Gy in patients without preexisting liver disease or primary liver cancer. The mean liver dose should be ≤28 Gy in those patients with preexisting liver disease [11]. However, part of the liver could be safely treated with higher doses with acceptable complications. Dose per fraction is an important factor in the development of RILD. Altered fractionated radiation therapy with large fraction sizes decreases liver tolerance [12].
The combination of chemotherapy agents with whole liver radiation seems to decrease liver tolerance [13, 14], although studies with use of fluoropyrimidines [15–18] or partial liver radiation have not been reported to significantly increase hepatic complications of combination therapy.
Baseline liver function is an important factor in predicting the occurrence of RILD. Cirrhotic livers have a lower tolerance for radiation. It has been reported that patients with worse Child-Pugh Class (B vs. A) and chronic hepatitis B carriers are at higher risk for developing RILD [9].
Patients with elevated liver enzymes during radiation therapy are at risk for developing RILD [12]. Such liver abnormalities are presumably related to self-limited liver inflammation [19].
Primary hepatobiliary carcinoma is associated with a significantly increased risk of RILD compared with a diagnosis of liver metastases; this could be related to preexisting cirrhosis or hepatitis in patients with hepatobiliary carcinoma [20].
14.3 Timing
RILD typically occurs 4–8 weeks after the completion of treatment, although it has been described as early as 2 weeks and as late as 7 months afterward [3].
14.4 Symptoms
In severe cases, patients develop rapid weight gain, increase in abdominal girth, liver enlargement, right upper quadrant discomfort, ascites, and jaundice [3]. Physical examination reveals ascites and hepatomegaly in moderate to severe cases, although in mild cases these signs are detectable only by ultrasound or abdominal CT scan [3]. Serum alkaline phosphatase is predominantly elevated (more than twice the upper limit of normal or baseline value), with minimal increase or relatively normal levels of aspartate transaminase (AST) and alanine (ALT) (in the range of twofold above normal) and bilirubin [3, 19].
In patients with intrahepatic cancer, decreases in alkaline phosphatase because of tumor response may mask alkaline phosphatase elevation resulting from RILD [23].
In patients with hepatitis and cirrhosis, nonclassic-type RILD with markedly elevated serum transaminases (>5 times the upper limit of normal) rather than elevated alkaline phosphatase or a decline in liver function (measured by a worsening of Child-Pugh score by 2 or more) and jaundice can occur, indicating severe radiation-induced injury to hepatocytes [19].
Reactivation of viral hepatitis has been reported in patient who underwent radiation therapy; however, its role in RILD pathogenesis is unclear. Elevation of transaminases rather than commonly reported increase in alkaline phosphatase is seen in hepatitis B carrier patients and implies radiation injury of hepatocytes rather than bile ducts [9]. It has been suggested that the risk of hepatitis B reactivation is decreased with prophylactic antiretroviral therapy [19].
Thrombocytopenia has been reported in children studies related to congestion of the portal bed and spleen and secondary hypersplenism [24].
14.5 Scoring
Most research uses the Cancer Therapy Evaluation Program and Common Terminology Criteria for Adverse Events (CTCAE) to evaluate liver toxicity after radiation therapy [25]. The Cancer Therapy Evaluation Program, Common Terminology Criteria for Adverse Events (CTCAE), Version 3.0, defines grades 2, 3, 4, and 5 liver dysfunction as jaundice, asterixis, encephalopathy or coma, and death, respectively. No grade 1 has been defined (Table 14.1) [19].
Table 14.1
CTCAE.V3 liver dysfunction criteria
Definition | |
---|---|
Grade 1 | – |
Grade 2 | Jaundice |
Grade 3 | Asterixis |
Grade 4 | Encephalopathy |
Grade 5 | Death |
14.6 Diagnosis
Patients with typical clinical picture and history of recent radiation therapy to the liver included in treatment field have a high likelihood of having RILD.
In patients with intrahepatic cancer, progression of cancer should be ruled out before making the diagnosis of RILD. An abdominal CT scan and paracentesis of the ascites are typically performed as part of the differential diagnosis [3].
Ascitic fluid obtained by paracentesis is consistent with a transudate feature (the serum to ascites albumin gradient > 1.1) with cytological negativity for malignant cells [26].
Irradiated liver in RILD appears hypodense on non-contrast CT scans. This CT finding is limited to radiation fields with well-defined linear margins in partial liver irradiation with more than 45 Gy [27, 28]. The sharp margins of demarcation are not seen in patients that received radiation through several non-axial and non-coplanar portals. The hypodensity of the irradiated area most likely correlates with changes of increased water content resulting from either edema or vascular congestion [29]. On contrast CT scans, irradiated areas are hypodense on the portal venous phase due to hypoperfusion and decreased contrast inflow and become hyperdense on the delayed phase obtained 4 min after contrast injection due to decreased venous drainage and stasis [30]. Maximal effect of radiation on liver appearance in CT images is seen 2–3 months after completion of therapy and is reversible with return to normal appearance [29].
The area of low density on CT has high signal intensity on the T2-weighted and low intensity signal on the T1-weighted sequence of MR images [27, 31]. Contrast enhancement of the irradiated area could be seen in MRI imaging after administration of gadopentetate dimeglumine (Gd-DTPA) due to the increased capillary permeability and chondroitin sulfate iron colloid (CSIC) due to hypofunction of the reticuloendothelial system [32].
The irradiation area has diminished function that could be detected with radioisotope scan as the area with decreased radioisotope uptake. These functional scans have the potential to quantify hepatocyte function to distinguish functional regions of hepatocytes from nonfunctional zones. Technetium (99mTc) sulfur colloid (SC) single-photon emission computed tomography is used as the imaging modality to image liver function, and there is a correlation between differential SC uptake and varying doses of radiation delivered [33].
14.7 Prevention
Prevention of RILD is paramount due to its fatal potential.
Three-dimensional treatment planning offers the potential to determine the liver radiation dose and volume, RILD risk could be estimated using dose-volume histogram (DVH) parameters, and modification of treatment planning should be considered if the RILD risk is high enough.
With the availability of intensity-modulated radiotherapy (IMRT), stereotactic body radiotherapy (SBRT), and image guidance in radiation treatment planning, radiation can be delivered with better liver sparing.
Coa et al. recently have proposed a risk assessment strategy for radiation treatment planning and re-optimization of the plan during therapy for intrahepatic radiation treatment. The assessment of the patient’s individual portal vein perfusion dose-response function during therapy and prediction of residual liver function after radiation could allow for detection of patients at high risk for RILD and adjusting the treatment plan [34, 35].
Amifostine is an organic thiophosphate that was developed to selectively protect normal tissues against the toxicities of chemotherapy and radiation. Systemically or regionally, administration of amifostine effectively protects hepatocytes from ionizing radiation damage without compromising the antitumor effect of radiation. Feng et al. observed that the use of amifostine allows for a higher dose of whole liver radiation to be safely administered and suggested the possibility of using amifostine in combination with radiation for patients with focal liver disease undergoing intensity-modulated radiotherapy or stereotactic body radiotherapy for focal liver tumors [36, 37].
Theoretically, anticoagulation may exert a protective effect against acute radiation injury by precluding fibrin clot formation and decrease the hepatic congestion. Lightdale et al. observed a possible protective effect for warfarin in a small group of Hodgkin’s disease patients [23]. Further evaluation of anticoagulation effect on radiation hepatic injury appears warranted.
Exposure to ionizing radiation enhances production of reactive oxygen species and decreases the levels of antioxidant in liver, whereas selenium and vitamin E supplementation can modulate the changes in liver. Gençel et al. observed significant decrease in oxidative stress in rat liver with selenium and vitamin E administration prior to a 7 Gy exposure relative to control animals [38].
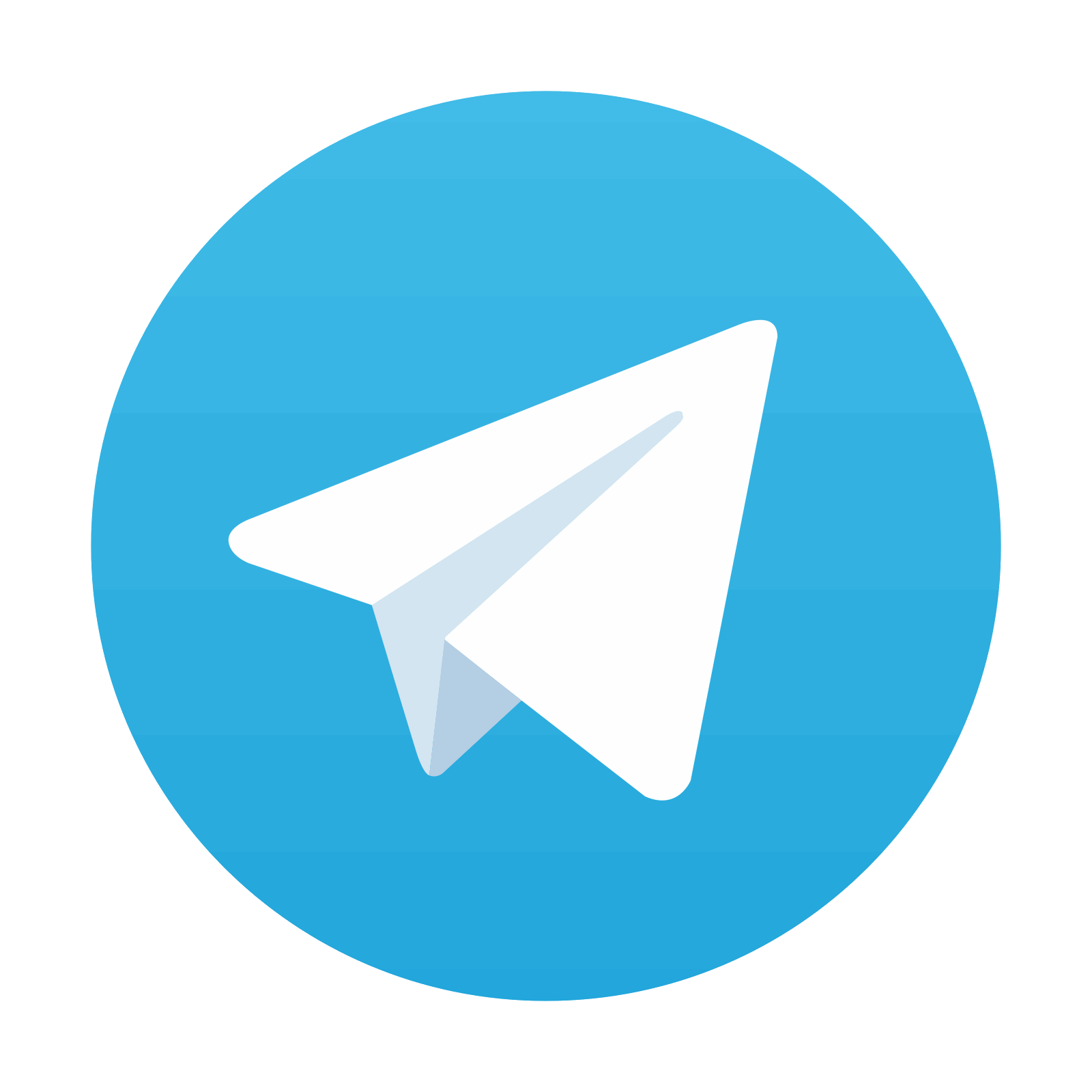
Stay updated, free articles. Join our Telegram channel

Full access? Get Clinical Tree
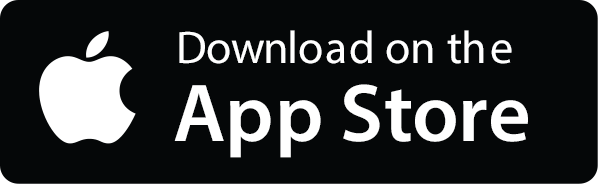
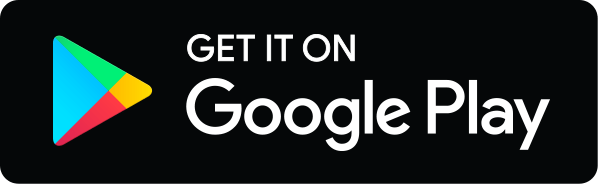