2 Radiation Safety R. Torrance Andrews and Todd L. Kooy The increased availability and acceptance of fluoroscopically guided minimally invasive procedures has significantly altered the management of such disparate women’s health concerns as osteoporotic vertebral fractures, breast masses, uterine fibroids, fertility, and chronic pelvic pain. Conditions that once required major surgery and prolonged recovery for their treatment can now be managed on an outpatient basis with little or no morbidity. However, even as these techniques have reduced the risks associated with traditional surgical alternatives, they have introduced the new and often underappreciated risk of radiation injury. Exposure to ionizing radiation can cause local injury ranging from erythema to skin necrosis, can increase the long-term risk of malignancy, and can both reduce fertility by damaging ovarian function and increase the risk of genetic damage to a patient’s future children. Although the likelihood of such outcomes is low, the risk is nonetheless infinitely higher than would be the case if no radiation were utilized. Furthermore, there is neither a visible nor a sensory indication to the patient or the operator that an injury is being induced: radiation damage is painless in the acute phase. For these reasons, it is imperative that the operator be aware of, and observe, all available precautions for reducing radiation exposure. Radiation effects can be divided into two broad categories: stochastic and nonstochastic (also referred to as deterministic). Stochastic effects are those that can occur at any radiation dose, without a finite safety threshold.1 The likelihood of a stochastic effect rises with increasing exposure, but the effect itself is binary — it either occurs or it does not — and the amount of radiation has no impact upon its severity. Examples of these chance events include genetic damage leading to cancer and chromosomal defects in future children, either of which could theoretically occur at very low doses, but might not occur even at very high doses.1,2 Nonstochastic or deterministic radiation effects, in contrast, occur only once a specific threshold exposure value has been reached and increase in severity with increased dose. Skin injury at the beam entry site and radiation-mediated ovarian failure are examples of nonstochastic events: clinically significant skin injury at the beam entry site does not occur below a threshold of 200 cGy and ovarian failure does not occur below a threshold of 400 cGy.2–4 Radiation-induced skin damage is the most commonly observed injury following interventional procedures. As indicated, the severity of a skin injury is dependent upon the radiation dose (that is, it is a nonstochastic, or deterministic, event). Both the degree of skin injury and its progression over time follow a predictable course (Table 2.1). The first sign of injury is erythema, which can be seen within just a few hours of exposure to a dose of 200 cGy; temporary or permanent epilation follows within 3 to 4 weeks of doses of 300 to 700 cGy, respectively; a dose of 1000 cGy can cause desquamation after 4 to 5 weeks; and skin necrosis can occur within 10 weeks of an 1800 cGy dose. Unfortunately, once these injuries have occurred, there are few options for treatment other than palliative support and, in severe cases, skin grafting. Avoiding injurious doses is thus the most important factor in patient outcome. Stochastic effects are also of great concern in interventional procedures that utilize ionizing radiation. Uterine fibroid embolization (UFE) and ovarian vein embolization, for instance, are procedures that specifically seek to conserve the organs of reproduction. Beyond simply retaining these organs, many women are hoping to preserve or even improve their fertility, with the goal of becoming pregnant. An injury that induced malignant degeneration in the reproductive tract or caused chromosomal damage in as-yet unfertilized oocytes would be devastating. However, by definition, stochastic events are a matter of chance and are therefore impossible to predict with certainty. Furthermore, the likelihood of such events is known to vary by tissue type, with some organs, like the ovaries, being more “radiation-sensitive” than others (Table 2.2). Because the likelihood of these events increases with dose and because, again, there is no outward indication of injury, the only mechanism for protecting patients is to minimize their radiation exposure.
Radiation Effects
Organ or Tissue | Relative Sensitivity |
Gonads | 20 |
Red bone marrow | 12 |
Colon | 12 |
Lung | 12 |
Stomach | 5 |
Bladder | 5 |
Breast | 5 |
Liver | 5 |
Esophagus | 5 |
Thyroid | 5 |
Skin | 1 |
Bone | 1 |
Source: Adapted from Brateman L. Radiation safety considerations for diagnostic radiology personnel. Radiographics 1999;19(4):1037–1055. Adapted by permission.
Dose Monitoring
Continuous, real-time monitoring of radiation dose during interventional procedures is critical and serves several purposes. An unexpectedly high dose rate may be the operator’s best indication that otherwise occult technical or procedural factors are negatively impacting a given case. Having this information immediately available may allow for immediate corrective action. It also allows the operator to avoid a nonstochastic injury: as the dose approaches the 200 cGy threshold for a skin burn, for example, he or she can consider terminating the procedure or taking other steps (as discussed below) to stay below this threshold. If the threshold is exceeded, this fact must be recognized so that the patient can be followed appropriately for the development of clinical manifestations. Knowing a specific dose is less useful for predicting stochastic injuries, but a relative risk can be estimated.
Unfortunately, reliable dose monitoring is difficult, and all of the techniques in current use have significant limitations.
Fluoroscopic Time
Recording the number of minutes during which the imaging beam is active is quite simple. By law, all fluoroscopic equipment sold in the United States must be equipped with a mechanism for recording the fluoroscopic time, along with an alarm that sounds as each 5-minute increment passes. As a result, fluoroscopic time has become the most widely used tool for tracking patient dose. Unfortunately, this measure does not account for the dose delivered during image acquisitions, which is significantly higher than that delivered during fluoroscopy. A single recorded image can generate a dose equivalent to over 40 seconds of fluoroscopy,5 and the dose delivered during a typical angiographic sequence of 20 to 40 images can therefore far exceed the amount of radiation being “measured” by fluoroscopic time. In addition, fluoroscopic time fails to reflect such factors as beam collimation, filtration, magnification, and other aspects of imaging technique that can critically impact the dose rate. These factors are discussed in detail later in this chapter.
Dose Area Product
Dose area product (DAP) is a calculated value derived by multiplying the actual dose delivered to the patient by the area over which the radiation is experienced. This value, which is calculated by the fluoroscopic equipment in units of Gy · cm2, includes both the dose from fluoroscopic observation and that from image acquisition; it also reflects the use of collimation, filtration, magnification, and other techniques used to reduce patient exposure. DAP is therefore a much better tool than fluoroscopic time for monitoring overall patient exposure, and thus the risk for stochastic injury. Unfortunately, it provides only a general estimate of dose at the entry site: a small region exposed to a large amount of radiation might have the same DAP as a large region exposed to a small dose, but would have a much greater risk for local injury.
In Situ Dosimeters
A direct measurement (rather than a calculation) of dose can be made at a specific point in or on a patient’s body with a personal dosimeter placed at that point. This device can be a thermoluminescent dosimeter, a radiolucent probe, or, over a larger treatment area, radiation verification film. The primary advantage of such an approach is its high degree of accuracy. It is completely independent of all techniques utilized during a procedure and gives a very reliable indication of the actual dose being absorbed at the point where it is placed. Unfortunately, this information is not available until the dosimeter has been read, a process that can take several days; therefore, no real-time feedback is provided to the operator. In addition, placement of the device into a body cavity is, by definition, invasive, and may create complications independent of the procedure being performed.
Risk-Reduction Techniques
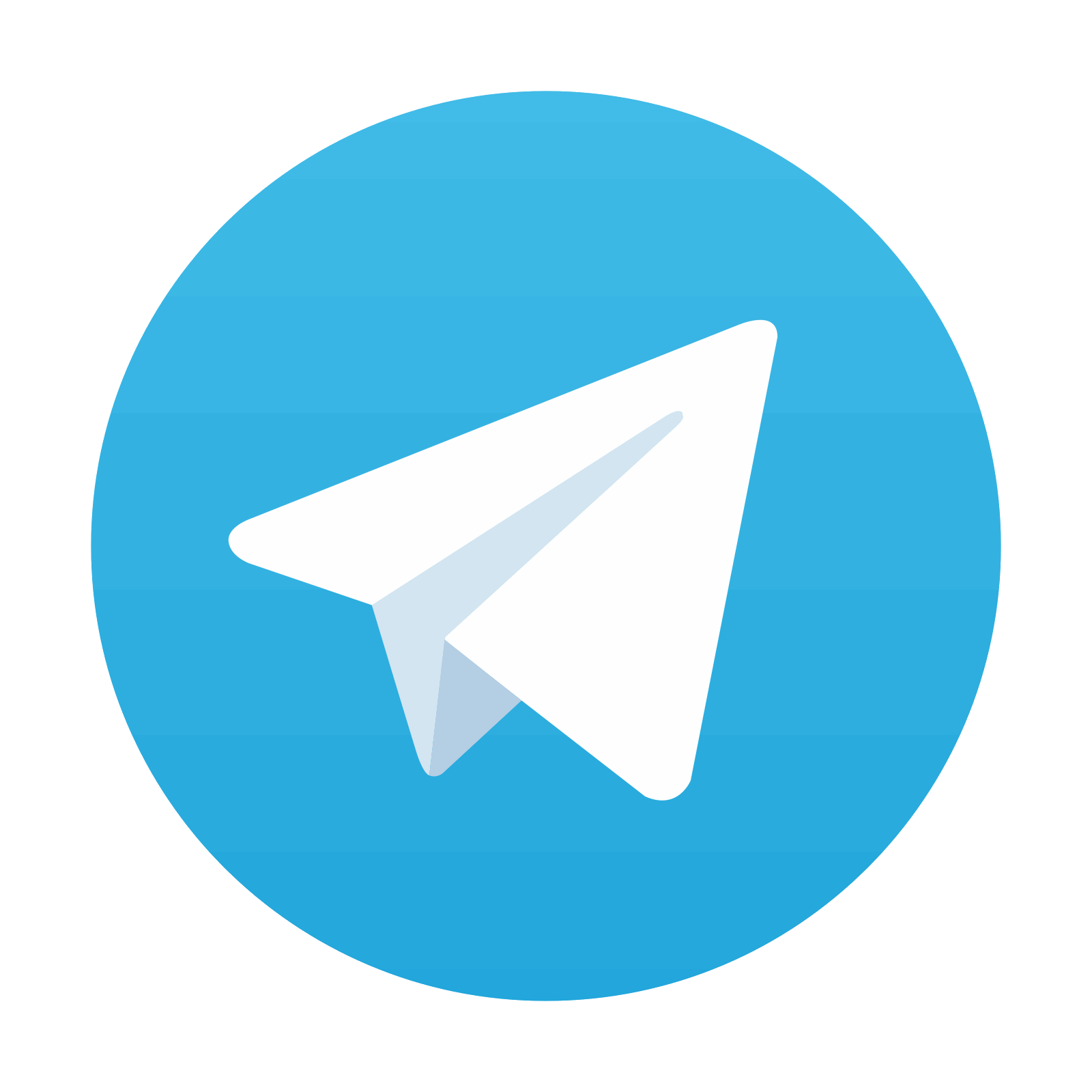
Stay updated, free articles. Join our Telegram channel

Full access? Get Clinical Tree
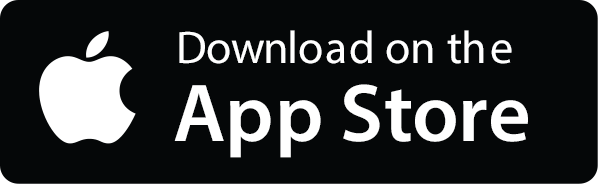
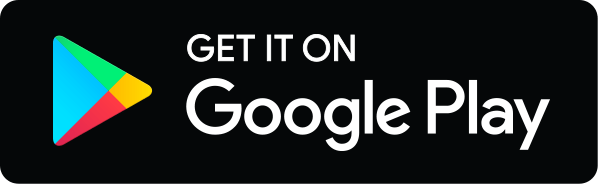