Radiation Sources for Emission Scanning: Positron and Gamma Emission
Cyrill Burger
Alfred Pfeiffer
Nuclear medicine is based on pharmaceuticals labeled with a suitable radioactive isotope. After such a radiopharmaceutical has been applied to a patient, it takes part in physiologic processes. A radioactive isotope has an unstable nucleus that will undergo one or several transitions (called decays) to finally reach a stable configuration. As a result of these decays, photons can be generated. Depending on their energy, they may penetrate the body tissue and be recorded by external detectors. From the detected photons, the emission images depict the distribution of the radiopharmaceutical in tissues at the acquisition time.
Different types of nuclear decays give rise to photons usable in nuclear medicine. For proton-rich nuclei, a beta-plus or an electron-capture decay may occur. The beta-plus decay ejects a positron. As a secondary event, the positron annihilates with an electron, producing two 511-keV photons that are emitted in opposite directions. In the electron-capture decay, a shell electron is absorbed by the nucleus. The resulting vacancy in the electron shell is filled by an electron from an outer shell, and the energy difference is transformed into a characteristic x-ray photon. Neutron-rich nuclei may decay by a beta-minus transition, by which an electron is ejected. Often the resulting daughter nucleus is in an excited state, from which it will return to the ground state by the emission of characteristic gamma-ray photons.
Radionuclides for medical purposes originate from cyclotrons, nuclear reactors, or secondary decays in generators. In cyclotrons, a suitable target material is bombarded with particles such a protons or deuterons to produce proton-rich radionuclides. From nuclear reactors, radionuclides can be obtained in two ways: one is to put a stable target into the high-neutron flux in the reactor, and neutron-rich radionuclides are then produced in nuclear transformations. The other is to extract fission products of the uranium 235 (235U) fission reaction. The ease and efficiency of radionuclide separation depends on the chemical properties of the radionuclide. The best specific activity is obtained if the atomic number differs from that of the unwanted material, as is the case with proton-enriched cyclotron products. A generator contains a longer-lived parent nuclide that decays into an unstable daughter nuclide suitable for emission imaging. The advantage of generators is that the daughter nuclide can be extracted in regular intervals directly at a clinical site, solving the logistic problem for routine medical investigations (Table 2.1).
Table 2.1 Medically Important Radionuclides | ||||||||||||||||||||||||||||||||||||||||||||||||||||||||||||||||||||||||||||||||
---|---|---|---|---|---|---|---|---|---|---|---|---|---|---|---|---|---|---|---|---|---|---|---|---|---|---|---|---|---|---|---|---|---|---|---|---|---|---|---|---|---|---|---|---|---|---|---|---|---|---|---|---|---|---|---|---|---|---|---|---|---|---|---|---|---|---|---|---|---|---|---|---|---|---|---|---|---|---|---|---|
|
Introduction
Nuclear medicine is based on labeling a pharmaceutical with a suitable radioactive isotope. After application to a patient, the radiopharmaceutical (see Chapters 14, 15, 16, 17 and 18) takes part in physiologic processes. Radioactive decays are continuously taking place and can be detected from the outside by the emitted gamma rays, allowing monitoring of the distribution of the tracer concentration. Several properties are required for a radioactive isotope to be suitable for nuclear medicine. The half-life of the radiopharmaceutical must be long enough to be delivered to the site of use, if it is not locally produced, and to permit acquisition of patient data; yet it should be short for radiation-protection reasons. The energy of the emitted photons must be high enough to penetrate the body tissues but low enough to be stopped and detected in the detectors of the gamma and positron emission tomography (PET) cameras. Finally, the chemical properties of the isotope should be such that it can be easily bound into stable molecules of interest.
Radioactive Decay Modes
A simplified atomic model is used for outlining the most relevant processes that eventually result in gamma rays usable for emission imaging in nuclear medicine. An atom consists of a nucleus surrounded by electrons (Fig. 2.1). The nucleus is formed by a number Z of positively charged protons and a number N of neutrons. Z is called the atomic number and determines the chemical properties of the atom; therefore, the atomic number has a one-to-one relation to a chemical symbol and a corresponding name. For instance, all atoms with Z = 6 are carbon C atoms. The term “nuclide” is used when referring to an atomic species with a defined number of protons and neutrons. The same number of protons may be combined with different numbers of neutrons. For carbon, configurations with five, six, seven, or eight neutrons are denoted as 116C, 126C, 136C, and 146C and are called C isotopes. Whether a nucleus is stable depends on the relative number of protons and neutrons: the N/Z ratio. When plotting the stable N/Z configurations as a function of Z, a “line of stability” starts with 1 and increases with the atomic number, reaching 1.54 for lead 208 (Pb) (1). In nature, about 270 stable configurations occur in elements and hence lie along this line. Unstable nuclides are positioned above the line of stability (neutron rich) or below (proton rich). They undergo transitions also called “radioactive decay processes” to reach a more stable configuration and by means of which particles or gamma rays are emitted. Hence they are called “radionuclides.” Of the 2,700 known radionuclides, about
60 occur naturally; the others are produced using different techniques, mostly in reactors or cyclotrons.
60 occur naturally; the others are produced using different techniques, mostly in reactors or cyclotrons.
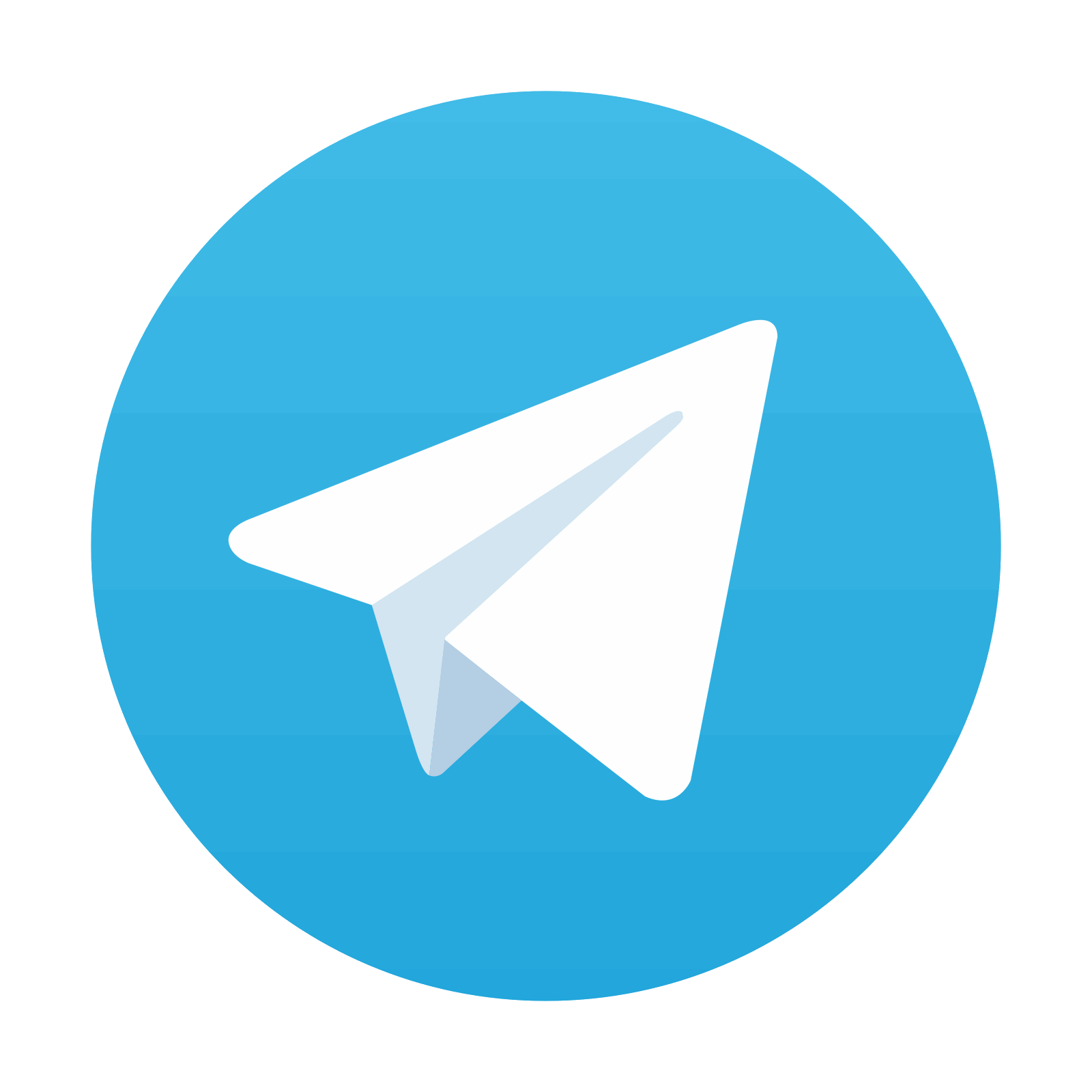
Stay updated, free articles. Join our Telegram channel

Full access? Get Clinical Tree
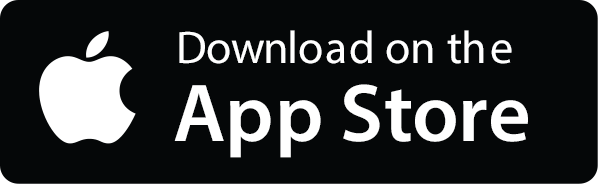
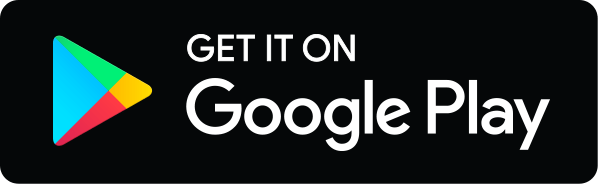