Fig. 3.1
Induction of anti-tumor immunity by RFA combined with immunotherapy
3.5.2.1 Stimulation of Antigen Presentation
The instantaneous release and availability of tumor antigens for antigen-presenting cells founded interest in strategies to increase antigen presentation to T cells . Increasing the numbers of DCs by transfer of ex vivo-generated DCs is the most straightforward approach in this respect. Although DC vaccination indeed increased the efficacy of radiotherapy [94–96], intratumoral ethanol injections [97], cryoablation [98, 99], and photodynamic therapy [100, 101], the intratumoral injection of ex vivo-engineered bone marrow-derived DCs failed to improve the outcome of RFA in a murine model for urothelial carcinoma [65]. Unfortunately a trial by Engleman [102] to study the effects of DC injection after RFA was prematurely terminated.
In a model of cryoablation of melanoma tumors we found that the ablative treatment combined with peri-tumor injections of the TLR9 agonist CpG resulted in superior protection against tumor re-challenges [35]. The clinical efficacy of CpG was highly dependent on the timing of administration relative to the ablation [103] and was also directly correlated with the timing of injection and subsequent in vivo co-localization of antigens and CpG within DCs [36]. The immune adjuvant efficacy of CpG was founded specifically upon TLR9 function in plasmacytoid DCs that subsequently stimulated the CD8a +DCs and merocytic DCs to cross-prime tumor-specific CTLs [104]. The administration of CpG in close proximity to the ablated area is a simple procedure in RFA where the insertion of RF probes enables an immediate entry for the injection of immune stimulants. A clinical trial is currently in progress in patients with colorectal liver metastases evaluating the safety and efficacy of intrahepatic CpG injections in combination with RFA.
3.5.2.2 Stimulation of T Cell Reactivity
Saito et al. [105] established a murine orthotopic model of head and neck squamous cell carcinoma (HNSCC) and additionally treated the mice with IL-2 gene transfer. The combinational treatment was most successful in reducing tumor growth, which coincided with increased influx of macrophages and DCs, enhanced CTL activity and protection against tumor re-challenges. Habibi et al. [85] performed RFA on mammary carcinomas in FVBN202 transgenic or BALB/c mice and co-administered IL-7 and IL-15 intra-lesionally. These cytokines were selected based on their supporting role in proliferation and homeostasis of effector and memory T cells . In contrast to IL-2, they do not induce activation-associated cell death, nor are they needed for the maintenance of regulatory T cells . Only combinational treatment resulted in tumor-specific IFN-g responses in splenocytes and protected the mice following a tumor re-challenge. Similarly, combining RFA of murine hepatoma with three (every 2 days) subcutaneous injections of an active variant of CC chemokine ligand 3/macrophage inflammatory protein-1alpha (ECI301) eliminated the RFA-treated primary tumors and retarded the growth of contra-laterally injected (non-RFA-treated) tumors, accompanied by CCR1-dependent T cell infiltration [106].
We previously established a mouse model in which B16 melanoma tumors were destructed by RFA and showed that 10–40 % of the RFA-treated mice were able to reject a re-challenge with an otherwise lethal dose of B16. Additional systemic administration of anti-CTLA4 mAb (clone 9H10) increased the survival rate to 70 % and drastically elevated the levels of tumor-specific T cells [34]. These data implicated that blocking the transmission of T cell inhibitory signals is a potent strategy to increase the clinical efficacy after RFA. It would be interesting to study the infiltration of CTLs into the ablated tumor area after these treatments.
Recently, T cell transfer approaches have been tested in combination with RFA. Transfer of autologous mononuclear cells stimulated with anti-CD3 and human fibronectin fragment (CH-296) in the presence of IL-2, to prepare so-called RetroNectin activated killer (RAK) cells, after RFA was found to be safe and induce increased IFN-g levels in five out of six patients. No clinical data is yet available.
3.6 Conclusions and Future Perspective
Percutaneous RFA has emerged as a promising treatment alternative for cancer patients, offering several advantages over conventional treatments; the technique is minimally invasive, encompasses low morbidity and mortality rates and has shown superior efficacy in comparison with other alternative treatments, such as percutaneous ethanol injections.
Although very diverse in their ways to induce cell death, ablative techniques share one key feature: the in situ availability of the ablated material. During the efforts of the body to clear this dead material there is a time frame in which the immune system is actively controlling immune responses directed towards antigens from this antigen ‘depot’. The ability to stimulate the immune system with antigens from dead tumor cells has led to the idea that RFA can be used to achieve ‘in vivo vaccination’ against tumors.
Many studies have demonstrated antigen-specific T cells or antibodies following RFA, but results show that this does not necessary dictate effectiveness of the induced immune response . Even activation of APCs by the cell-death associated mediators, may not be enough to generate immune responses that clear entire tumors. The possibility that not only intact tumors employ mechanisms to suppress immune responses, but also the body itself when its facing massive cell death of self-based tissue, is unfortunately less well studied. Most studies, including ours, report only low numbers of specific T cells following RFA as a stand-alone treatment. It therefore seems fair to conclude that tumor-directed immune responses exist, but that they are relatively weak. The fact that only very rarely spontaneous regression of metastases after RFA of the primary tumor is observed is illustrative for this. Currently, data is lacking about which ablation technique might result in the most effective release of antigens and the most immunostimulatory environment. Unlike RFA, techniques like for instance cryoablation will not denature a large part of the proteins and cause release of different antigens . Future research will have to compare the distinct types of ablation in a context of immune stimulation.
The weak immune responses following ablation suggest that local enforcement by immune interventions can be helpful to boost immunity . As discussed in this review, strategies that employ or activate DCs in the tumor area seem to be most effective in this. Additional stimulation with TLR ligands or immune stimulating antibodies might be valuable tools to create solid immune responses. The immune modulating antibody CTLA4 is an example of a tool that is now available for clinical use and could be easily combined with RFA treatment [107].
It remains a challenge to link the quality of the antigens generated and the potency of the endogenous or exogenous immune modulation to clinical results. Of importance here is the question which immunological correlate is predictive for clinical outcome and should therefore be used to monitor the effects of ablation . De Vries et al. [108] reported that the presence of tumor-specific T cells in delayed-type hypersensitivity skin biopsies, rather than levels of specific T cells found in peripheral blood, after DC vaccination of melanoma patients correlated with clinical outcome. In line with these results, we predict that the presence of activated effector CD8 + T cells in the ablated tumor area and/or the intra-tumor ratio of CD8 + T cells versus Treg ratios may be a better prognostic marker for treatment efficacy than the presence in peripheral blood.
In conclusion, immunomodulatory approaches are likely to become an important part of the armamentarium in the treatment of cancer, as mono-therapies, but especially in combination approaches together with in situ tumor destruction techniques. Immunotherapy will benefit from the primary function of radiofrequency ablation , which is debulking of tumor mass, as immunotherapy itself will most likely not be sufficient to cure end-stage cancers. Learning to ‘shape’ the initial ablation-induced immunity by external enhancements will provide us with robust treatment modalities for combination with in situ tumor destruction techniques.
Radiofrequency ablation instantly releases tumor antigens from the tumor mass, which may flow to the draining lymph through lymphatic drainage or may be taken up by phagocytic cells, such as DCs, and transported to the lymph node. RF-induced cell damage will release endogenous danger signals as well as (pro-) inflammatory mediators that further DC maturation, resulting in the (specific) stimulation of immune effector cells. Nevertheless, RFA induces (weak) immune responses that are only occasionally strong enough to lead to spontaneous regression and does poorly protect against secondary growth, local recurrence, or metastasis. Combinational therapies of RFA with immune stimulatory approaches may further stimulate tumor-specific immunity leading to enhanced local and systemic tumor control. For example, local DC vaccinations may increase the level of antigen presentation. Furthermore, immune adjuvants can be administered to ensure full DC maturation, and the administration of immune stimulating compounds, such as anti-CTLA-4, will result in profound T cell proliferation. Such approaches may be best positioned in a regimen together with strategies to abrogate immune regulatory networks.
References
1.
Tiong L, Maddern GJ (2011) Systematic review and meta-analysis of survival and disease recurrence after radiofrequency ablation for hepatocellular carcinoma. Br J Surg 98:1210–1224PubMed
2.
Guglielmi A, Ruzzenente A, Valdegamberi A et al (2008) Radiofrequency ablation versus surgical resection for the treatment of hepatocellular carcinoma in cirrhosis. J Gastrointest Surg 12:192–198PubMed
3.
Rossi S, Di Stasi M, Buscarini E et al (1996) Percutaneous RF interstitial thermal ablation in the treatment of hepatic cancer. AJR Am J Roentgenol 167:759–768PubMed
4.
Guglielmi A, Ruzzenente A, Battocchia A et al (2003) Radiofrequency ablation of hepatocellular carcinoma in cirrhotic patients. Hepato Gastroenterol 50:480–484
5.
Buscarini L, Buscarini E, Di Stasi M et al (2001) Percutaneous radiofrequency ablation of small hepatocellular carcinoma: long-term results. Eur Radiol 11:914–921PubMed
6.
Giovannini M, Moutardier V, Danisi C et al (2003) Treatment of hepatocellular carcinoma using percutaneous radiofrequency thermoablation: results and outcomes in 56 patients. J Gastrointest Surg 7:791–796PubMed
7.
Abdalla EK, Vauthey J-N, Ellis LM et al (2004) Recurrence and outcomes following hepatic resection, radiofrequency ablation, and combined resection/ablation for colorectal liver metastases. Ann Surg 239:818–825PubMed
8.
Machi J, Oishi AJ, Sumida K et al (2006) Long-term outcome of radiofrequency ablation for unresectable liver metastases from colorectal cancer: evaluation of prognostic factors and effectiveness in first- and second-line management. Cancer J 12:318–326PubMed
9.
Elias D, De Baere T, Smayra T et al (2002) Percutaneous radiofrequency thermoablation as an alternative to surgery for treatment of liver tumour recurrence after hepatectomy. Br J Surg 89:752–756PubMed
10.
Henn AR, Levine EA, McNulty W et al (2003) Percutaneous radiofrequency ablation of hepatic metastases for symptomatic relief of neuroendocrine syndromes. AJR Am J Roentgenol 181:1005–1010PubMed
11.
Zgodzinski W, Espat N-J (2005) Radiofrequency ablation for incidentally identified primary intrahepatic cholangiocarcinoma. World J Gastroenterol 11: 5239–5240PubMed
12.
Sudheendra D, Barth MM, Hegde U et al (2006) Radiofrequency ablation of lymphoma. Blood 107:1624–1626PubMed
13.
Owen RP, Ravikumar TS, Silver CE et al (2002) Radiofrequency ablation of head and neck tumors: dramatic results from application of a new technology. Head Neck 24:754–758PubMed
14.
Zlotta AR, Djavan B, Matos C et al (1998) Percutaneous transperineal radiofrequency ablation of prostate tumour: safety, feasibility and pathological effects on human prostate cancer. Br J Urol 81:265–275PubMed
15.
Thanos L, Mylona S, Pomoni M et al (2006) Percutaneous radiofrequency thermal ablation of primary and metastatic lung tumors. Eur J Cardiothorac Surg 30:797–800PubMed
16.
Susini T, Nori J, Olivieri S et al (2007) Radiofrequency ablation for minimally invasive treatment of breast carcinoma. A pilot study in elderly inoperable patients. Gynecol Oncol 104:304–310PubMed
17.
Poggi G, Gatti C, Melazzini M et al (2003) Percutaneous ultrasound-guided radiofrequency thermal ablation of malignant osteolyses. Anticancer Res 23:4977–4983PubMed
18.
Mouraviev V, Joniau S, Van Poppel H et al (2007) Current status of minimally invasive ablative techniques in the treatment of small renal tumours. Eur Urol 51:328–336PubMed
19.
Goldberg SN, Solbiati L, Halpern EF et al (2000) Variables affecting proper system grounding for radiofrequency ablation in an animal model. J Vasc Interv Radiol 11:1069–1075PubMed
20.
Nikfarjam M, Muralidharan V, Christophi C (2005) Mechanisms of focal heat destruction of liver tumors. J Surg Res 127:208–223PubMed
21.
Vanagas T, Gulbinas A, Sadauskiene I et al (2009) Apoptosis is activated in an early period after radiofrequency ablation of liver tissue. Hepato Gastroenterol 56:1095–1099
22.
Wiersinga WJ, Jansen MC, Straatsburg IH et al (2003) Lesion progression with time and the effect of vascular occlusion following radiofrequency ablation of the liver. Br J Surg 90:306–312PubMed
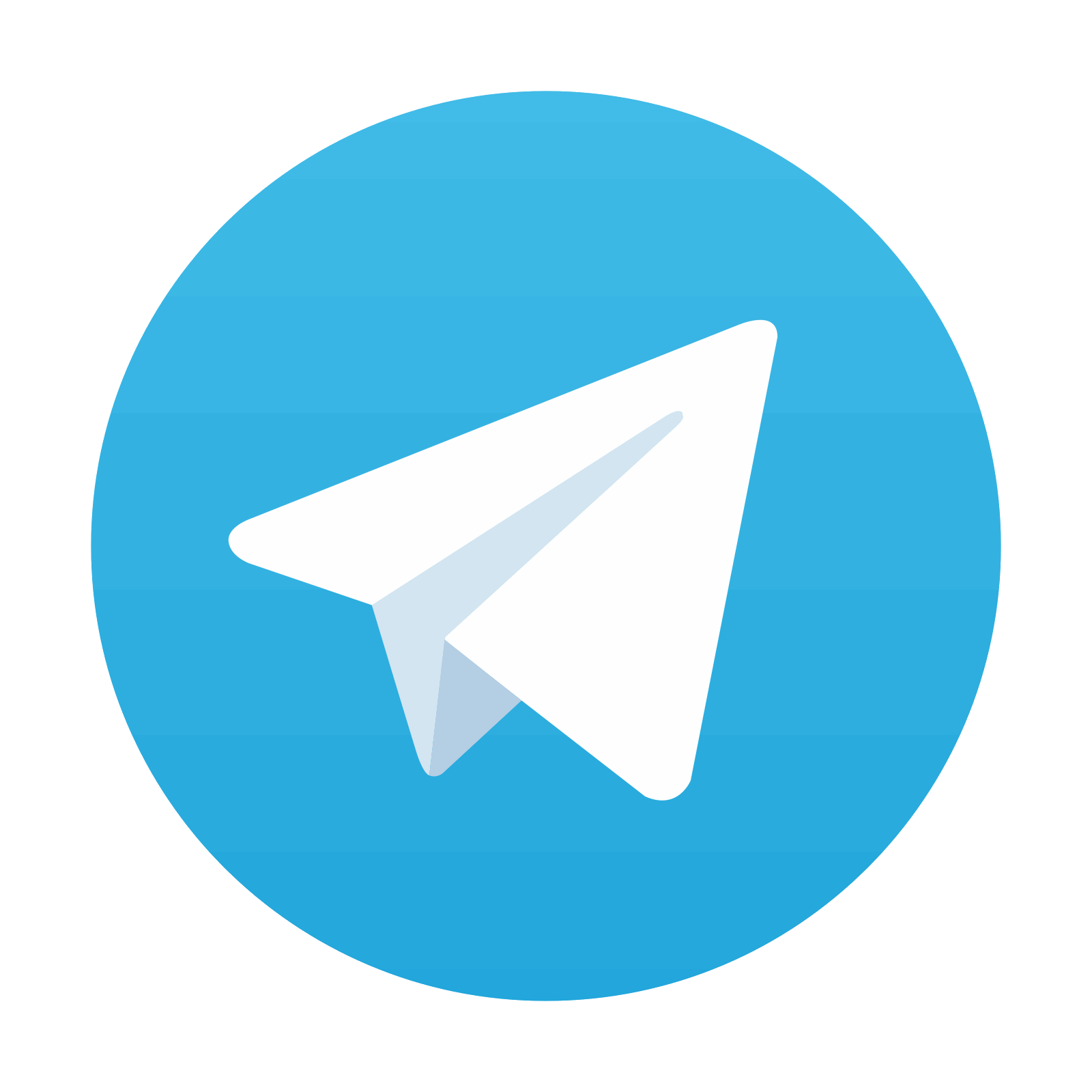
Stay updated, free articles. Join our Telegram channel

Full access? Get Clinical Tree
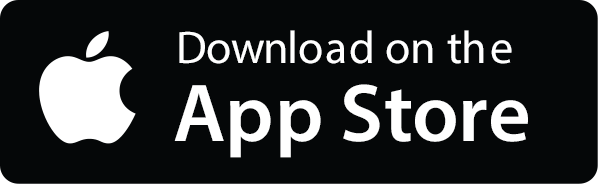
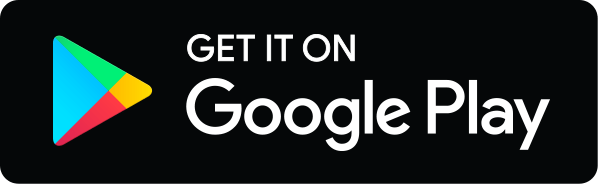