Radiologic Imaging Modalities
RADIOGRAPHY AND DIGITAL RADIOGRAPHY (DR)
Among the variety of available imaging techniques, the most important modality for the evaluation of arthritis is conventional radiography. It is clearly the procedure of choice for the initial examination because of its effectiveness in evaluating the bone and joint changes associated with arthritic disorders, including diminution of the radiographic joint space, osteopenia, sclerosis, subchondral cysts, erosions, osteophytosis, periosteal reaction, and soft tissue calcifications. Characteristic radiographic features, when pathognomonic, may suggest a diagnosis or at least a differential diagnosis. The radiographs may provide objective evidence for the presence and extent of abnormalities, and serial studies may be utilized for recording progress or its lack during the course of a therapy and thus may influence the choice of treatment. In addition, correlation of the radiographic findings with clinical impressions may be useful in determining prognosis. Standard radiographic techniques with minor technical adjustments provide indirect and direct information regarding almost all the tissues within and surrounding the joint. Detailed features of osseous structures along with less well-defined visualization of soft tissues can be demonstrated. Although articular cartilage is not visible, its thickness can be gauged by determining the width of radiographic joint space, with thinning of the cartilage reflected by diminution of visible joint space.
Standard radiographs of the involved joint should be obtained in at least two projections at 90 degrees to each other. Special projections may at times be required to demonstrate destructive changes in the joint to better advantage. In the shoulder, the special posterior oblique view, known as the Grashey projection, permits the glenoid to be seen in profile. This variant of the anteroposterior (AP) projection in shoulder imaging is obtained by placing the patient in a 40-degree posterior oblique position and directing the center beam toward the glenohumeral joint. This projection shows the glenoid in true profile and clearly visualizes the glenohumeral joint space, which is obscure in the standard AP projection (Fig. 2.1). The Grashey view has been found to be particularly effective in demonstrating subtle shoulder joint erosions in inflammatory arthritides. A superoinferior view, known as the axillary projection, is helpful to determine the exact relationship of the humeral head and the glenoid (Fig. 2.2A,B) and is effective in demonstrating the degenerative and inflammatory abnormalities of the glenohumeral joint (Fig. 2.2C). This view, however, may at times be difficult to obtain, particularly if the patient is unable to abduct the arm, in which case a variant of the axillary projection known as the West Point view may be similarly effective (Fig. 2.3). Another useful variant of the axillary projection is the Lawrence view. The importance of this projection lies in the fact that it does not require full abduction of the arm because it can be compensated for by angulation of the radiographic tube (Fig. 2.4). Abnormalities of the acromioclavicular joint are best seen on the special view of this structure obtained with 15 degrees cephalad tilt of the radiographic tube (Fig. 2.5A,B). The key to this other variant of the AP view of the shoulder is a special low-kilovoltage technique in which the radiographic factors are reduced to ˜ 33% to 50% of those used in the standard technique for the AP view. The excellent visualization of the acromial end of the clavicle, the acromion, and the acromioclavicular joint afforded by this projection can be helpful in demonstrating early resorptive changes of the distal end of the clavicle seen in rheumatoid arthritis (Fig. 2.5C) and hyperparathyroid arthropathy. In the elbow, the radial head-capitellum view, by eliminating overlap of the radial head and coronoid
process and by more clearly demonstrating the humeroradial and humeroulnar joints, shows the inflammatory changes in the elbow joint to better advantage (Fig. 2.6). This projection has proved to be particularly useful in evaluating the capitellum, radial head, and coronoid process. The semisupinated oblique view of the hand and wrist (the so-called ball-catcher view), introduced by Nørgaard in 1965, effectively demonstrates the radial aspects of the
metacarpal heads and of the base of the proximal phalanges in the hand and the triquetrum-pisiform joint in the wrist (Fig. 2.7). Because the earliest erosive changes of some inflammatory arthritides begin in these areas, the Nørgaard view may provide important information at the early stages of arthritides. It may also demonstrate subtle subluxations in metacarpophalangeal joints frequently seen in systemic lupus erythematosus (SLE). In the hip, demonstration of the anatomic structures of the proximal femur including the greater and lesser trochanters is facilitated by the frog-leg lateral projection (Fig. 2.8). Demonstration of the sacroiliac joints requires either a posteroanterior projection, which is obtained to greater advantage with 25 to 30 degrees caudal angulation of the radiographic tube, or an AP view with 30 to 35 degrees cephalad angulation, known as the Ferguson view (Fig. 2.9). In the knee, a weight-bearing view may be of value, particularly for a dynamic evaluation of any decrement in the joint space under the weight of the body (Fig. 2.10). On the Merchant projection (Fig. 2.11), the patellofemoral disorders are better evaluated than on the standard sunrise view (Fig. 2.12). This projection is especially effective in demonstrating the articular facets of the patella and femur and can be helpful in detecting subtle subluxations of the patella. In the ankle, the Harris-Beath view is effective in demonstrating the posterior and medial facets of the subtalar joint (Fig. 2.13), whereas Broden’s view, in addition to clear visualization of the posterior facet of the subtalar joint, provides a good image of the sustentaculum tali, talofibular joint, and talofibular syndesmosis (Fig. 2.14). In the cervical spine, an open-mouth view provides effective visualization of the structures of the first two cervical vertebrae. The body of C2 is clearly imaged, as well as the atlantoaxial joints, the odontoid process, and the articular pillars of C1 (Fig. 2.15). If the open-mouth view is difficult to obtain, or the odontoid process is not clearly visualized, the Fuchs
view may be helpful (Fig. 2.16). Flexion-extension views in the lateral projections are needed to evaluate stability of C1-C2 articulation and predental space and are particularly effective in demonstrating the abnormalities of these structures in the patients with rheumatoid arthritis affecting the cervical spine (Fig. 2.17). Oblique view is critical for evaluation of cervical neural foramina (Fig. 2.18). Because the visualization of C7 and T1 vertebrae is often obscured on the standard projections owing to overlap of the clavicle and soft tissues of the shoulder girdle, the swimmer’s view provides the solution to this problem (Fig. 2.19). In the thoracic spine, to clearly demonstrate the vertebral bodies and disk spaces on the lateral projection, the special technique known as autotomography is used, which requires shallow breathing by the patient to blur the structures involved in respiratory motion and gives a clear view of the thoracic vertebral column (Fig. 2.20).
process and by more clearly demonstrating the humeroradial and humeroulnar joints, shows the inflammatory changes in the elbow joint to better advantage (Fig. 2.6). This projection has proved to be particularly useful in evaluating the capitellum, radial head, and coronoid process. The semisupinated oblique view of the hand and wrist (the so-called ball-catcher view), introduced by Nørgaard in 1965, effectively demonstrates the radial aspects of the
metacarpal heads and of the base of the proximal phalanges in the hand and the triquetrum-pisiform joint in the wrist (Fig. 2.7). Because the earliest erosive changes of some inflammatory arthritides begin in these areas, the Nørgaard view may provide important information at the early stages of arthritides. It may also demonstrate subtle subluxations in metacarpophalangeal joints frequently seen in systemic lupus erythematosus (SLE). In the hip, demonstration of the anatomic structures of the proximal femur including the greater and lesser trochanters is facilitated by the frog-leg lateral projection (Fig. 2.8). Demonstration of the sacroiliac joints requires either a posteroanterior projection, which is obtained to greater advantage with 25 to 30 degrees caudal angulation of the radiographic tube, or an AP view with 30 to 35 degrees cephalad angulation, known as the Ferguson view (Fig. 2.9). In the knee, a weight-bearing view may be of value, particularly for a dynamic evaluation of any decrement in the joint space under the weight of the body (Fig. 2.10). On the Merchant projection (Fig. 2.11), the patellofemoral disorders are better evaluated than on the standard sunrise view (Fig. 2.12). This projection is especially effective in demonstrating the articular facets of the patella and femur and can be helpful in detecting subtle subluxations of the patella. In the ankle, the Harris-Beath view is effective in demonstrating the posterior and medial facets of the subtalar joint (Fig. 2.13), whereas Broden’s view, in addition to clear visualization of the posterior facet of the subtalar joint, provides a good image of the sustentaculum tali, talofibular joint, and talofibular syndesmosis (Fig. 2.14). In the cervical spine, an open-mouth view provides effective visualization of the structures of the first two cervical vertebrae. The body of C2 is clearly imaged, as well as the atlantoaxial joints, the odontoid process, and the articular pillars of C1 (Fig. 2.15). If the open-mouth view is difficult to obtain, or the odontoid process is not clearly visualized, the Fuchs
view may be helpful (Fig. 2.16). Flexion-extension views in the lateral projections are needed to evaluate stability of C1-C2 articulation and predental space and are particularly effective in demonstrating the abnormalities of these structures in the patients with rheumatoid arthritis affecting the cervical spine (Fig. 2.17). Oblique view is critical for evaluation of cervical neural foramina (Fig. 2.18). Because the visualization of C7 and T1 vertebrae is often obscured on the standard projections owing to overlap of the clavicle and soft tissues of the shoulder girdle, the swimmer’s view provides the solution to this problem (Fig. 2.19). In the thoracic spine, to clearly demonstrate the vertebral bodies and disk spaces on the lateral projection, the special technique known as autotomography is used, which requires shallow breathing by the patient to blur the structures involved in respiratory motion and gives a clear view of the thoracic vertebral column (Fig. 2.20).
Digital (computed) radiography (DR or CR) is the name given to the process of digital image acquisition using an x-ray detector comprising a photostimulable phosphor imaging plate and image reader-writer that processes the latent image information for subsequent brightness scaling
and laser printing on film (Fig. 2.21). The system works on the principle of photostimulated luminescence. When the screen absorbs x-rays, the x-ray energy is converted to light energy by the process of fluorescence, with the intensity of light being proportional to the energy absorbed by the phosphor. The stimulated light is used to create a digital image (a computed radiograph). A major advantage of DR/CR over conventional film-screen radiography is that once acquired, the digital image data are readily manipulated to produce alternative renderings. Moreover, advantages of digitalization include contrast and brightness optimization by the manipulation of window width and level settings as well as a variety of image processing capabilities, quantization of image information, and facilitation of examination storage and retrieval. Digital imaging has vastly replaced conventional radiography, and images are mostly viewed in a PACS (picture archive and communication system) workstation, allowing one to change contrast and orientation, choose magnification, and obtain measurements of linear distances and angles, among many other customizations.
and laser printing on film (Fig. 2.21). The system works on the principle of photostimulated luminescence. When the screen absorbs x-rays, the x-ray energy is converted to light energy by the process of fluorescence, with the intensity of light being proportional to the energy absorbed by the phosphor. The stimulated light is used to create a digital image (a computed radiograph). A major advantage of DR/CR over conventional film-screen radiography is that once acquired, the digital image data are readily manipulated to produce alternative renderings. Moreover, advantages of digitalization include contrast and brightness optimization by the manipulation of window width and level settings as well as a variety of image processing capabilities, quantization of image information, and facilitation of examination storage and retrieval. Digital imaging has vastly replaced conventional radiography, and images are mostly viewed in a PACS (picture archive and communication system) workstation, allowing one to change contrast and orientation, choose magnification, and obtain measurements of linear distances and angles, among many other customizations.
TOMOGRAPHY AND COMPUTED TOMOGRAPHY
Among the ancillary imaging techniques, conventional tomography was used in the past, its major purpose being to demonstrate to better advantage the degree of joint destruction. Currently it has been replaced by computed tomography (CT).
CT is a radiologic modality containing an x-ray source, detectors, and a computer data processing system. The essential components of CT system include a circular scanning gantry, which houses the x-ray tube and image sensors; a table for the patient; an x-ray generator; and a computerized data processing unit. The patient lies on the table and is placed inside the gantry. The x-ray tube is rotated 360 degrees around the patient while the computer collects the data and formulates an axial image, or “slice.” Each cross-sectional slice represents a thickness between 0.1 cm and 1.5 cm of body tissue. The newest CT scanners use a rotating fan of x-ray beams, a fixed ring of detectors, and predetector
collimator. A highly collimated x-ray beam is transmitted through the area being imaged. The tissues absorb the x-ray beam to various degrees depending on the atomic number and density of the specific tissue. The remaining, unabsorbed (unattenuated) beam passes through the tissues and is detected and processed by the computer. The CT computer software converts the x-ray beam attenuations of the tissue into a CT number (Hounsfield units) by comparing them with the attenuation of water. The attenuation of water is designed as 0 (zero) H; that of air is designated as -400 to -1,000 H, fat as -60 to -100 H, body fluid as +20 to +30 H, muscle as +40 to +80 H, trabecular bone as +100 toa300 H, and normal cortical bone as +1,000 H. Routinely, axial sections are obtained; however, computer-reformatted images taken in multiple planes or reconstructed with three-dimensional (3D) techniques may be generated if desired.
collimator. A highly collimated x-ray beam is transmitted through the area being imaged. The tissues absorb the x-ray beam to various degrees depending on the atomic number and density of the specific tissue. The remaining, unabsorbed (unattenuated) beam passes through the tissues and is detected and processed by the computer. The CT computer software converts the x-ray beam attenuations of the tissue into a CT number (Hounsfield units) by comparing them with the attenuation of water. The attenuation of water is designed as 0 (zero) H; that of air is designated as -400 to -1,000 H, fat as -60 to -100 H, body fluid as +20 to +30 H, muscle as +40 to +80 H, trabecular bone as +100 toa300 H, and normal cortical bone as +1,000 H. Routinely, axial sections are obtained; however, computer-reformatted images taken in multiple planes or reconstructed with three-dimensional (3D) techniques may be generated if desired.
The introduction of spiral (helical) scanning was a further improvement of CT. This technique, referred to as volume-acquisition CT, has made possible a data-gathering system using continuous rotation of the x-ray source and the detectors. It allows the rapid acquisition of volumes of CT data and renders the ability to reformat the images at any predetermined intervals ranging from 0.5 mm to 10.0 mm. Unlike standard CT, in which up to a maximum of 12 scans could be obtained per minute, helical CT acquires all data in 24 or 32 seconds, generating up to 92 sections. This technology has markedly reduced scan times and has eliminated interscan delay and hence interscan motion. It also has decreased the motion artifacts, improved the definition of scanned structures, and markedly facilitated the ability to obtain 3D reconstructions generated from multiple overlapping transaxial images acquired in a single breath-hold. Spiral CT allows data to be acquired during the phase of maximum contrast enhancement, thus optimizing the detection of abnormality. The data volume may be viewed either as conventional transaxial images (Figs. 2.22 and 2.23) or as multiplane reformation (Fig. 2.24) and 3D reconstruction (Fig. 2.25).
Most recently, with the advent of multichannel multi-detector-row CT (MDCT), images can be generated with subsecond gantry rotation times yielding high-resolution volume data sets, and at the same time minimizing the radiation dose to the patient. Even more advanced is high-resolution flat-panel volumetric CT (fpVCT), which uses digital flat-panel detectors and provides volumetric coverage as well as ultra-high spatial resolution in two-dimensional (2D) and 3D projections. Furthermore, it reduces metal and beam-hardening artifacts. In addition to the aforementioned features, fpVCT also allows dynamic imaging of time-varying processes.
Occasionally, iodinated contrast agents may be used intravenously to enhance the CT images. A contrast agent directly alters image contrast by increasing the x-ray attenuation, thus displaying increased brightness in the CT images.
CT is effective in evaluating degenerative and inflammatory changes of various joints (see Figs. 2.22B, C, 2.24, and 2.25), and in the spine to document spinal stenosis (see Fig. 2.23). In the assessment of spinal stenosis secondary to degenerative changes, CT examination may also be performed after myelography (Fig. 2.26), although myelography alone is often sufficient (see later and Fig. 2.38).
![]() Figure 2.25 ▪ Computed tomography, 3D reconstruction. A, B: 3D CT images of an elbow joint in shaded surface display demonstrate advanced osteoarthritis. |
Recently dual-energy CT (DECT) gained a wide acceptance as a modality used for detection or exclusion of tophaceous gout. The DECT system is equipped with two x-ray tubes with different peak kilovoltages (80 kVp and 140 kVp), thus allowing simultaneous acquisition of two sets of images of the desired anatomic region. The material-specific differences in attenuation of various elements enable classification of the chemical composition of scanned tissue, allowing accurate and specific characterization and separation of monosodium urate from calcium-containing mineralization. DECT data yield color-coded cross-sectional images, clearly depicting the foci of accumulation of urate crystals (Fig. 2.27). The 3D reconstructed images may also be generated (Fig. 2.28). In addition, in patients with known tophaceous gout, this technique may be used for serial volumetric quantification of subclinical tophus to evaluate response to treatment.
ARTHROGRAPHY
ANGIOGRAPHY, CT ANGIOGRAPHY, AND MR ANGIOGRAPHY (MRA)
The use of contrast material injected directly into selective branches of both the arterial (arteriography) and venous (venography) circulation has aided greatly in assessing the involvement of the vascular system in systemic rheumatologic diseases and has provided a precise method for defining local pathology. Characteristic anatomic locations and patterns of involvement can be seen in such disorders as Takayasu arteritis, temporal arteritis, polyarteritis nodosa, and other forms of systemic vasculitis. Because generalized arteritis commonly involves the bowel, resulting in ischemia, necrosis, and hemorrhage, selective arteriography may be used diagnostically to differentiate diffuse from localized processes. Currently, instead of arteriography, CT angiography (Figs. 2.31, 2.32, and 2.33) and magnetic resonance arteriography (Figs. 2.34, 2.35, and 2.36) are more commonly performed. In particular, the latter imaging technique, with recent innovations in hardware and software and the advent of 3.0-Tesla magnets
has allowed reduction in exogenous contrast dose without compromising overall image quality. It is an effective modality allowing detection of aneurysms, vessel stenosis, and obstruction; by using the double invasion recovery (DIR) “black blood” technique (by suppressing the signal from flowing blood while maintaining high signal in the surrounding stationary tissues), it can also assess the vessel wall thickness. Conventional arteriography, however, can be used therapeutically by employing embolization with specially designed coils or clot-promoting or endogenously formed clotted material to stop localized areas of hemorrhage, often preventing a major abdominal surgical procedure in an otherwise severely compromised patient. Central vessels occlusion is achieved by detachable balloons, metallic coils, or Gelfoam particles. Conversely, for peripheral and capillary vessels occlusion, Ethibloc, tissue glue and particles with an average size from 500 µm to 750 µm can be employed.
has allowed reduction in exogenous contrast dose without compromising overall image quality. It is an effective modality allowing detection of aneurysms, vessel stenosis, and obstruction; by using the double invasion recovery (DIR) “black blood” technique (by suppressing the signal from flowing blood while maintaining high signal in the surrounding stationary tissues), it can also assess the vessel wall thickness. Conventional arteriography, however, can be used therapeutically by employing embolization with specially designed coils or clot-promoting or endogenously formed clotted material to stop localized areas of hemorrhage, often preventing a major abdominal surgical procedure in an otherwise severely compromised patient. Central vessels occlusion is achieved by detachable balloons, metallic coils, or Gelfoam particles. Conversely, for peripheral and capillary vessels occlusion, Ethibloc, tissue glue and particles with an average size from 500 µm to 750 µm can be employed.
MYELOGRAPHY AND CT MYELOGRAPHY
Myelography is still occasionally being used alone (Figs. 2.37, 2.38, 2.39, 2.40) or in conjunction with CT in evaluation of spinal stenosis or disk herniation (Figs. 2.41 and 2.42); however, its role has been significantly diminished with use of CT and magnetic resonance imaging (MRI).
DISKOGRAPHY
Diskography is a radiologic procedure consisting of injection of a contrast material into the nucleus pulposus under fluoroscopic guidance. Although this is a controversial procedure abandoned by many radiologists, under tightly restricted indications and immaculate technique, a diskogram can yield valuable information. Diskography is an
effective aid to determine the source of a patient’s low back pain. It is not purely an imaging technique because the symptoms produced during the test (pain during the injection of contrast, known as pain provocation) are considered to have even greater diagnostic value than do the obtained radiographs. This technique should always be combined with CT examination (so-called CT diskography) (Figs. 2.43 and 2.44).
effective aid to determine the source of a patient’s low back pain. It is not purely an imaging technique because the symptoms produced during the test (pain during the injection of contrast, known as pain provocation) are considered to have even greater diagnostic value than do the obtained radiographs. This technique should always be combined with CT examination (so-called CT diskography) (Figs. 2.43 and 2.44).
![]() Figure 2.40 ▪ Myelography of disk herniation. Lateral spot film obtained during myelography in a 38-year-old man demonstrates a large posterior herniation of the intervertebral disk L4-L5 (arrow). |
SCINTIGRAPHY (RADIONUCLIDE BONE SCAN)
Scintigraphy is a modality that detects the distribution in the body of a radioactive agent injected into the vascular system. After an intravenous administration of a radiopharmaceutical agent, the patient is placed under a scintillation camera, which detects the distribution of radioactivity in the body by measuring the interaction of gamma rays emitted from the body with sodium iodide crystals in the head of the camera. The photoscans are obtained in multiple projections and may include either the entire body or selected parts. One major advantage of skeletal scintigraphy over all other imaging techniques (excluding positron emission tomography [PET]) is its ability to image the entire skeleton at once (Fig. 2.45). As Johnson remarked, it provides a “metabolic picture,” anatomically localizing a lesion by assessing its metabolic activity compared with that of adjacent normal bone. A bone scan can confirm the presence of the disease, demonstrate the distribution of the lesion,
and help to evaluate the activity of the pathologic process. Although it is a very sensitive modality, its specificity is very low. Indications for skeletal scintigraphy include traumatic conditions, tumors, various arthritides, infections, and metabolic bone diseases. The detected abnormality may consist of either decreased uptake of a bone-seeking radiopharmaceutical agent (e.g., in the early stage of osteonecrosis) or increased uptake (e.g., in case of fractures, neoplasms, or arthritis). Some anatomic structures under normal conditions may show increased activity (such as sacroiliac joints or growth plates).
and help to evaluate the activity of the pathologic process. Although it is a very sensitive modality, its specificity is very low. Indications for skeletal scintigraphy include traumatic conditions, tumors, various arthritides, infections, and metabolic bone diseases. The detected abnormality may consist of either decreased uptake of a bone-seeking radiopharmaceutical agent (e.g., in the early stage of osteonecrosis) or increased uptake (e.g., in case of fractures, neoplasms, or arthritis). Some anatomic structures under normal conditions may show increased activity (such as sacroiliac joints or growth plates).
In rheumatology, radionuclide bone scanning is much more commonly used than other techniques, mainly for evaluating the distribution of arthritis in different joints. The radiopharmaceuticals currently in use in bone scanning include organic diphosphonates—ethylene diphosphonate (HEPD) and methylene diphosphonate (MDP)—labeled with 99m technetium (99mTc), a gamma emitter with a 6-hour half-life; MDP is more commonly used, typically in a dose that provides 15 mCi (555 MBq) of 99mTc (see later). Its uptake in bone is related to both osteoblastic activity and the regional blood flow. After intravenous injection of the radiopharmaceutical, ˜ 50% of the dose localizes in bone, with the remainder circulating freely in the body and eventually excreted by the kidneys. A gamma camera can then be used in a procedure known as a four-phase radionuclide bone scanning. Scintigraphy can determine the distribution of arthritic changes in the skeleton (Figs. 2.46 and 2.47) and at the specific sites (Figs. 2.48, 2.49, 2.50, 2.51, 2.52), as well as in areas usually not detected by standard radiography like sternomanubrial or temporomandibular joints among others. This technique is also useful in distinguishing infected joint from infected periarticular soft tissues if radiography is not diagnostic and the patient cannot undergo MRI because of contraindications such as the presence of cardiac pacemaker, cerebral aneurysm clips, or claustrophobia. With cellulitis, diffusely increased uptake of the radiopharmaceutical agent is present in the first two phases, but there is no significant increase of uptake in the third or fourth delayed phases (Fig. 2.53). Conversely, osteomyelitis and septic arthritis cause focally increased uptake in all four phases (Fig. 2.54). Radionuclide bone scanning is also an effective modality to discriminate whether the failure of arthroplasty occurred secondary to mechanical loosening of the prosthesis or infection (Figs. 2.55, 2.56, 2.57, 2.58, 2.59, 2.60). To distinguish infectious arthritis from other forms of arthritides, indium-111 (111In) labeled white blood cells (WBCs), either alone or in combination with 99mTc sulfur colloid (also known as indium leukocyte imaging) (Figs. 2.61 and 2.62)
and 57Ga scans are employed. The use of 99mTc hexamethylpropylene amine oxime (HMPAO)-labeled WBC for diagnosing infectious processes has also been recently advocated. The kinetics and normal distribution of such leukocytes are similar to those of 111In-labeled leukocytes. The superior resolution and count density of 99mTc, however, gives this technique an advantage over the use of 111In-labeled WBCs. Another radiopharmaceutical used for detection of infection and inflammation is 99mTc-labeled interleukin 8 (IL-8). A small protein (8.5 kDa) that belongs to the CXC subfamily of chemokines, IL-8 is a chemotactic cytokine that binds with a high affinity to receptors expressed on neutrophils. More recently, imaging of the joints affected by rheumatoid arthritis was attempted using 99mTc-labeled specific murine anti-CD4 and nonspecific human immunoglobulin. The rationale to use this radiopharmaceutical agent was the presence of CD4 molecules on T helper cells and macrophages, both abundantly present in inflammatory infiltrates of rheumatoid arthritis. Moreover, some investigators developed a method for imaging inflammatory joints using a monoclonal antibody (1.2B6) against E-selectin, an endothelial cell-specific adhesion molecule. They compared 111In-anti-E-selectin
mAb and 99mTc-labelled human nonspecific immunoglobulins in the scintigraphic imaging of rheumatoid arthritis, concluding that 111In-1.2B6 is a sensitive method by which to assess RA activity and that targeting is more intense and specific than using 99mTc human immunoglobulin.
and 57Ga scans are employed. The use of 99mTc hexamethylpropylene amine oxime (HMPAO)-labeled WBC for diagnosing infectious processes has also been recently advocated. The kinetics and normal distribution of such leukocytes are similar to those of 111In-labeled leukocytes. The superior resolution and count density of 99mTc, however, gives this technique an advantage over the use of 111In-labeled WBCs. Another radiopharmaceutical used for detection of infection and inflammation is 99mTc-labeled interleukin 8 (IL-8). A small protein (8.5 kDa) that belongs to the CXC subfamily of chemokines, IL-8 is a chemotactic cytokine that binds with a high affinity to receptors expressed on neutrophils. More recently, imaging of the joints affected by rheumatoid arthritis was attempted using 99mTc-labeled specific murine anti-CD4 and nonspecific human immunoglobulin. The rationale to use this radiopharmaceutical agent was the presence of CD4 molecules on T helper cells and macrophages, both abundantly present in inflammatory infiltrates of rheumatoid arthritis. Moreover, some investigators developed a method for imaging inflammatory joints using a monoclonal antibody (1.2B6) against E-selectin, an endothelial cell-specific adhesion molecule. They compared 111In-anti-E-selectin
mAb and 99mTc-labelled human nonspecific immunoglobulins in the scintigraphic imaging of rheumatoid arthritis, concluding that 111In-1.2B6 is a sensitive method by which to assess RA activity and that targeting is more intense and specific than using 99mTc human immunoglobulin.
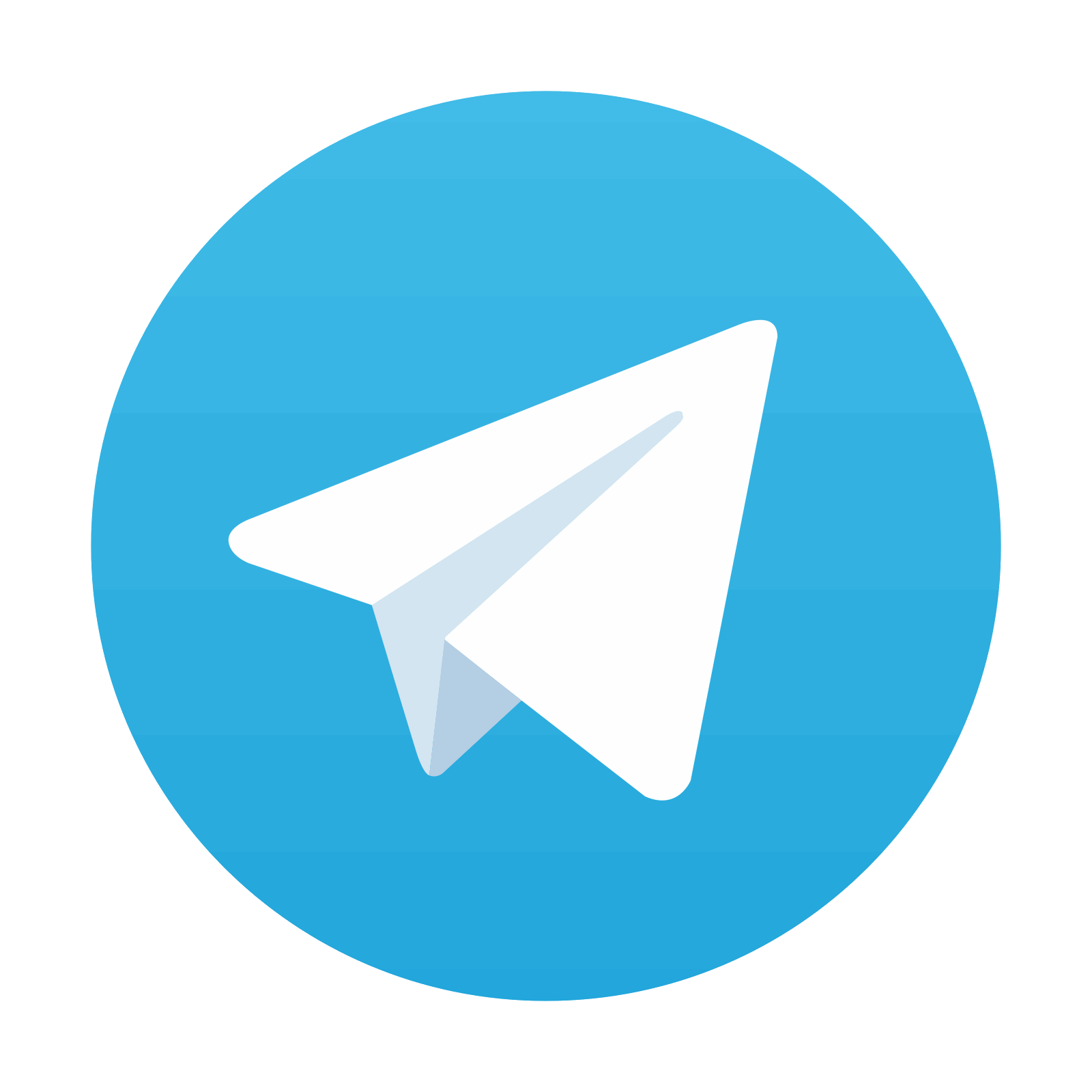
Stay updated, free articles. Join our Telegram channel

Full access? Get Clinical Tree
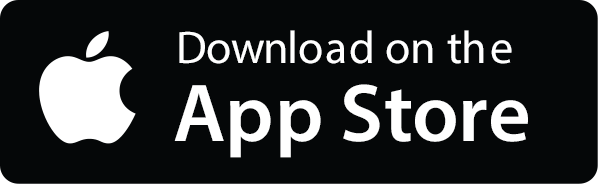
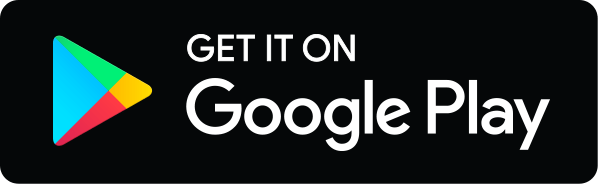