Fig. 14.1
Lateral chest CT scan showing posterior dislocation of the sternum (Courtesy of Elsevier: Lyons et al. 2010)
14.4 Pneumothorax
Pneumothorax is a possible life-threatening condition and should therefore be diagnosed and treated rapidly (Putukian 2004). Pneumothorax (PTX) is seldom a result of sporting activity, although it may not always be diagnosed correctly by emergency physicians, and thus the incidence might be higher than the literature suggests (Karnik 2001). Due to the high level of fitness of athletes, symptoms might remain unattended or be perceived as not significant, resulting in a delay of diagnosis (Soundappan et al. 2005; Pfeiffer and Young 1980).
Sports-related pneumothorax can be subdivided in spontaneous PTX and traumatic PTX. Traumatic PTX is associated with blunt trauma as well as pulmonary barotrauma.
Case reports of spontaneous pneumothorax indicate an association with sports activities like scuba diving (Harker et al. 1993), weightlifting (Marnejon et al. 1995), jogging (Pfeiffer and Young 1980; Kizer and MacQuarrie 1999), athletics (track running, Davis 2002) and basketball (Curtin et al. 2000). Incorrect breathing techniques or Valsalva manoeuvres might play a role in the onset of spontaneous pneumothorax in these sporting activities (Marnejon et al. 1995). Spontaneous pneumothorax occurs more frequently in tall and thin young males (Mulder et al. 2012).
Traumatic PTX can occur during sports activity. Blunt trauma during contact sports (football, ice hockey (Curtin et al. 2000; Partridge et al. 1997)) and noncontact sports (skiing, snowboarding, hiking, bicycling (Kizer and MacQuarrie 1999)) can lead to traumatic PTX. Blunt trauma can also lead to fractures of thoracic structures; sharp fracture fragments can perforate the parietal pleura and may lead to a traumatic pneumothorax. This injury mechanism is described in rib (Partridge et al. 1997) and clavicle (Dugdale and Fulkerson 1987) fractures. Children also can develop a traumatic pneumothorax after high-energy collisions during sports, as presented in case reports from patients playing rugby, football and bicycling (Soundappan et al. 2005).
Another injury mechanism for pneumothorax during sports is observed in scuba divers. Pulmonary barotrauma of ascent can result from scuba diving (Russi 1998; Buzzacott 2012). During the ascent the pressure of surrounding water to the body is decreased, leading to expansion of air inside the lungs and thus to expansion of the lungs through distention of the alveoli (Salahuddin et al. 2011). The overinflated lungs can get damaged on alveolar level if the diver does not exhale sufficiently when ascending. In this situation the alveoli may rupture, and air will enter the perivascular sheath that surrounds the alveoli, giving rise to interstitial emphysema. This interstitial emphysema can result in mediastinal emphysema (if perivascular sheaths are ruptured), arterial gas embolism (if pulmonary capillaries are ruptured) and pneumothorax (if the visceral pleura is ruptured) (Russi 1998; Salahuddin et al. 2011).
Imaging
If pneumothorax (and/or pneumomediastinum) is suspected, standard PA and lateral radiography should be performed (Partridge et al. 1997; MacDuff et al. 2010). Displacement of the pleural line and absence of lung markings are diagnostic for the (partial) collapse of the lung (MacDuff et al. 2010). PA and lateral X-rays in inspiration increase the sensitivity for minor pneumothorax by increasing the relative size of the affected area. CT scanning is the gold standard test if an exact estimation of the collapsed part is necessary or if standard radiography was not able to demonstrate pneumothorax in clinical suspected cases. Due to its low density, air is black on CT, and therefore a clear distinction between PTX (black) and lung tissue is seen when lung window is used (MacDuff et al. 2010; Perron 2003).
14.5 Rib Contusion, Fracture and Costochondral Separations
Rib fractures are the most common injury of the chest, especially in high-energy sports (Gielen et al. 2007; Mulder et al. 2012).
Middle (5–10) and lower ribs (11 and 12) are more frequently fractured than ribs 1–4 (Gielen et al. 2007). Fracture of one or more ribs during sports activity can be due to blunt trauma, compression trauma or other direct or indirect external forces. The fracture is mostly situated at the site of impact, but direct force to a small area coming from anterior can also fracture a rib at lateral position (Perron 2003). It is also possible that the costochondral junction is injured in this situation. Rib fractures are observed in both adults and children but occur more commonly in adults (Perron 2003; Miles and Barrett 1991).
Fractures can also be due to internal forces: locally violent muscle contraction in a different direction of pull can give rise to a typical athletes’ avulsion fracture. The insertion of the scalenus anterior muscle on the relatively flat and weak scalene tubercle at the first rib is a known avulsion fracture site (Sakellaridis et al. 2004). When the scalenus anterior contracts, for example, while playing tennis or throwing, the scalene tubercle can fracture, especially when the serratus anterior muscle also contracts pulling the first rib in caudal direction (Sakellaridis et al. 2004). These avulsion fractures have only been reported in the first and floating ribs (Miles and Barrett 1991). In the floating ribs it is the powerful contraction of the external or internal oblique, latissimus dorsi or serratus posterior muscles that can fracture the 11th or 12th rib(s) at the origin of these muscles.
Complicated rib fractures in athletes consist of multiple fracture sites (occurring, e.g. when the thorax is compressed), fractures resulting in flail chest and fractures in the first four and last two ribs (Miles and Barrett 1991). Flail chest can occur when multiple ribs are fractured at more than one site per rib, giving rise to a paradoxically moving rib cage segment. Flail chest, when not timely diagnosed, can result in respiratory insufficiency. Fractures in the first four and last two ribs can be dangerous since there might be a loose bone fragment present. Sharp fragments of these ribs can possibly damage important intrathoracic or intra-abdominal structures. A bone fragment can, for example, perforate the parietal pleura, causing a traumatic pneumothorax. Fracture of the first rib can result in neurovascular complications by injuring the subclavian artery and/or the brachial plexus (Phillips et al. 1981). When one of the lower ribs is involved, a bone fragment can result in hepatic, splenic or renal injury (Gielen et al. 2007; Al-Hassani et al. 2010).
Imaging
Imaging is unnecessary only if just one rib fracture is suspected and there are no other complications present (Mulder et al. 2012). If this is not the case, imaging starts with standard chest and rib radiographs, especially evaluating the region where the trauma took place (Perron 2003). Any visible cortical interruption points to a rib fracture. Costovertebral dislocation might also be identified on radiographs. Moreover, pneumothorax can be excluded using standard radiographs. However ultrasound is more sensitive for rib fractures, especially if the fracture is situated close to the costochondral junction and the trauma mechanism is mild to moderate (Turk et al. 2010). The fracture will be seen as an interruption of the anterior echogenic margin (Turk et al. 2010). Additionally ultrasound can be used to examine the patient for costochondral lesions and costochondral separations (Gielen et al. 2007; Turk et al. 2010). Using ultrasound, dynamic movement of involved structures can be seen during inspiration and expiration. A costochondral lesion presents itself with a non-gradually movement at the painful area and again a noncontinuous anterior echogenic margin (Gielen et al. 2007; Turk et al. 2010). A drawback of ultrasound is that it is highly dependent on operator experience and that some areas of the ribs are not accessible by ultrasound due to the overlying clavicle or scapula. CT can be used (Fig. 14.2) when X-ray and ultrasound of chest were negative but rib fracture is still considered presumable. In case of complicated rib fractures, additional imaging techniques such as angiography (suspected injury to subclavian artery) or CT abdomen should be considered.
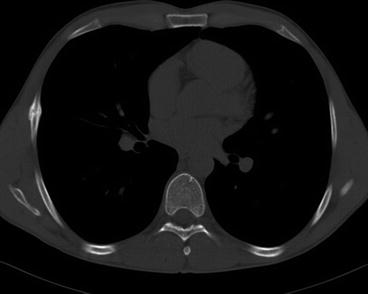
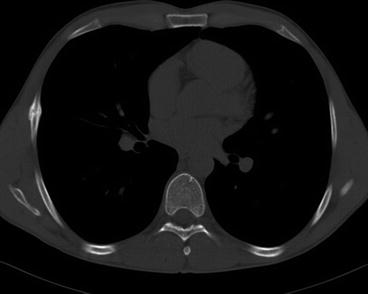
Fig. 14.2
Axial CT scan of a right fifth-rib fracture in a 29-year-old elite rower. Chronic phase with reparative callus bridging the fracture line. Window settings: W 1,600, C 400, slice thickness 2 mm
Caption
A professional sidecar motocross racer injured during the world championship race in 2013 demonstrated multiple rib fractures with serious injury. During the crash the driver fell below the sidecar. The clavicle and 12 ribs fractured; the racer was in the intensive care unit for 5 days.
14.6 Rib Stress Fracture
In a healthy subject, the bone is constantly remodelling, adapting to new or different loads the body puts on the skeleton. On the tissue level osteoblasts constantly produce lamellar bone. Osteoclasts enzymatically destruct bone and then resorb the damaged bony tissue.
When disbalance between osteoclastic bone resorption and osteoblastic bone production is present, bone gets weak and is vulnerable for microdamage, which can result in stress fractures (Anderson 2006). Different mechanisms play a role in the genesis of disbalance. In athletes, especially in beginners or when training is intensified, repetitive motion locally stimulates osteoclasts to resorb bone. Osteoblasts are also activated, but in the first weeks these cells are unable to keep up with the increased osteoclastic activity (Anderson 2006). During the first few weeks after beginning a sport or changing training or equipment, bone is more vulnerable for stress fractures (Anderson 2006).
An analysis of 263 Japanese athletes with a stress fracture showed that 14.1 % of all stress fractures in athletes occur in the ribs: only a stress fracture of the tibia is more often seen (Iwamoto et al. 2011). Of course this percentage is largely dependent on the type of sports that these athletes perform.
Rib stress fractures are associated with different sports, among others golf (Lord et al. 1996), canoeing (Maffulli and Pintore 1990), swimming (Taimela et al. 1995) and squash (Orava et al. 1991). Stress fractures of the middle ribs (5–9) are described extensively in rowers (Warden et al. 2002; Karlson 1998; Iwamoto et al. 2011). Repetitive contraction of the rectus abdominis, obliquus externus and/or serratus anterior muscle in rowing weakens the bone of the middle ribs and can result in a stress fracture, especially at the lateral part of the ribs where the muscles insert (Connolly and Connolly 2004; Karlson 1998; Rumball et al. 2005).
Stress fracture of the first rib has also been described but is more rare. In these fractures, the repeated pull from the scalenus anterior muscle (helping the thorax and shoulder girdle to retract the arms and paddle) and the serratus anterior muscle is thought to be the cause of first-rib stress fracture (Anderson 2006; Connolly and Connolly 2004). Sports/activities associated with first-rib stress fractures are basketball (Sacchetti et al. 1983), baseball pitching, weightlifting, volleyball and soccer (Matsumoto et al. 2003). Male ballet dancers who prepare for partner lifting with weight training are described to suffer from first-rib stress fractures (Prisk and Hamilton 2008); female ballet dancers are thought to be at risk for posterior lower rib stress fractures due to compression during the uplifting by their male partners (Bar-Sever et al. 1997).
Imaging
To identify stress fractures, a bone scintigraphy is described superior to standard X-rays and CT scan (Anderson 2006). The labelled diphosphonates show uptake where active bone turnover takes place, showing a ‘hot spot’ on bone scintigraphy scanning. Both the American College of Radiology in its Appropriateness Criteria and the IOC Manual of Sports Injuries point to a nuclear bone scan as the gold standard for diagnosing rib stress fractures (Mohammed et al. 1995; Mulder et al. 2012).
However, Anderson claims that MRI is as sensitive as bone scintigraphy. On MRI, bone marrow oedema and periosteal fluid are indicative for a stress fracture, and sometimes a thin fracture line can be seen. However caution should be taken, since asymptomatic persons may also show bone marrow oedema on MRI (Navas and Kassarjian 2011). Therefore, a precise history and clinical examination should precede MRI.
14.7 Sternoclavicular Joint Disorders
The sternoclavicular (SC) joint articulates using a small fibrocartilaginous disc. The SC joint is remarkably stable because of the tight capsular and ligamentous structures (Brys and Geusens 2007; Wirth and Rockwood 1996): three ligaments connect the sternum and clavicle directly to each other (interclavicular ligament, anterior SC ligament, posterior SC ligament). The costoclavicular ligament stabilises the joint by connecting the first rib to the rhomboid fossa of the clavicle (located posteriorly). Because of these structures, a dislocation of the SC joint is very rare: just 2–3 % of dislocations in the shoulder girdle are located at the sternoclavicular joint (Brys and Geusens 2007; Restrepo et al. 2009).
If a dislocation occurs, it involves anterior dislocation of the SC joint in the majority of patients (Restrepo et al. 2009). However in athletes who perform contact sports or high-energy sports, a posterior dislocation can be present. Anterior dislocation (or presternal dislocation) can be expected when a direct force hits the lateral part of the clavicle, pressing the sternal part of the clavicle forward/in ventral direction (Sakellaridis et al. 2004).
A forceful lateroposterior blow to the shoulder forces the lateral clavicle anteriorly, imposing a backward force at the sternoclavicular joint eventually leading to posterior dislocation of the sternal part of the clavicle (Buckley and Hayden 2008; Brys and Geusens 2007). An anteriorly directed force to the sternomedial part of the clavicle can also give rise to a posterior (or retrosternal) dislocation, but this is not often the case (Buckley and Hayden 2008). Contact sports as American football (Brys and Geusens 2007), rugby (Brys and Geusens 2007; Laffosse et al. 2010; Blakeley et al. 2011), judo and wrestling (Laffosse et al. 2010) and high-speed sports as motorcycling (Brys and Geusens 2007), skiing and equitation (Laffosse et al. 2010) are most often the cause of sports-related posterior sternoclavicular joint dislocation (Brys and Geusens 2007).
Posterior sternoclavicular dislocation can be dangerous since vital structures as the trachea, larynx, oesophagus, brachial plexus, recurrent laryngeal nerve, lungs, venous or arterial vessels and even the aorta can be compressed and/or ruptured (Buckley and Hayden 2008; Chaudhry et al. 2011). This may result in potentially life-threatening vascular and respiratory complications as laceration or occlusion of major vessels, pneumothorax and/or respiratory insufficiency.
Diagnosing sternoclavicular injuries in children and young adults can be difficult due to the late fusion of the medial epiphysis of the clavicle. This epiphysis ossifies at the age of 18–20, and only at age 23–25, it fuses with the clavicle shaft (Groh and Wirth 2011). Therefore in these patients, a physeal injury (Salter-Harris type 1 and 2 fractures) can be mistaken for a SC joint dislocation (Wirth and Rockwood 1996; Macdonald and Lapointe 2008).
14.7.1 Atraumatic Sternoclavicular Joint Disorders
Next to the traumatic sternoclavicular dislocation, athletes can suffer from sternoclavicular subluxation, which can be provoked by overuse. Cases from an adolescent female swimmer (Echlin and Michaelson 2006) and a military who was repetitively making heavy lifting and overhead movements (Gleason 2006) demonstrate the occurrence of overuse injury to the sternoclavicular joint.
Other atraumatic sternoclavicular joint disorders that might be important to consider for differential diagnosis of a swollen or painful SC joint are condensing osteitis, sternocostoclavicular hyperostosis and Friedreich’s disease (aseptic necrosis of medial clavicle), although they are not reported to have a direct relation with sporting activities (Carroll 2011; Hiramuro-Shoji et al. 2003). A thorough description of these rare conditions (including radiological findings) was made by Hiramuro-Shoji and colleagues (Hiramuro-Shoji et al. 2003).
Imaging
Sternoclavicular dislocation and subluxation is difficult to identify on AP and lateral radiographs. The so-called serendipity view (40° cephalic tilt view in AP) yields a somewhat better view of the SC joints (Wirth and Rockwood 1996). Another disadvantage of radiography is the impossibility to detect any intrathoracic complications. Cross-sectional imaging is advised in order to assess the joint and evaluate for asymmetry. In children and young adults, MRI is preferable because injury to the epiphysis is visible only on MRI, and this injury can then be differentiated from SC dislocation (Groh and Wirth 2011). Also, intrathoracic damage can be imaged using MRI.
14.8 Pectoralis Major Muscle Rupture
Partial or total pectoralis major muscle ruptures are relatively rare injuries that can result from an unsuspected fall or blow but also from eccentric muscle contraction as in heavy lifting (Provencher et al. 2010). The latter injury mechanism is observed more often in the last decades, accompanied by an increased rate of physical activity involving bench press and other muscle exercise/work-out in the gym. According to a systematic review by ElMaraghy, 48 % of all cases of muscle injury to the pectoralis major can be accounted for by the deep part of the lift in a bench press manoeuvre (ElMaraghy and Devereaux 2012). This also explains why case reports about men are more predominant than reports describing women with this particular muscle injury (Provencher et al. 2010).
Sports-related cases of pectoralis major rupture other than the gym include among others boxing, jiu-jitsu, windsurfing, soccer, skateboarding, Olympic gymnastics (De Castro Pochini et al. 2010), American football, rugby, ice hockey (Mulder et al. 2012) and steer wrestling (Wheat Hozack et al. 2013).
The complex anatomy of the pectoralis major muscle poses difficulties for both diagnosing and treating these injuries. The pectoralis major muscle is the major muscle in adduction, anteflexion and endorotation of the arm. The muscle can be divided in two parts: the clavicular head and the sternal head (ElMaraghy and Devereaux 2012). The sternal head is subdivided in 6–7 muscle segments. The sixth and seventh segment attach at the cartilage of (floating) ribs six and seven rather than at the costosternal border (ElMaraghy and Devereaux 2012). The most inferior part of the pectoralis major muscle is also called the sternoabdominal part and is different from the other parts due its steeper pennation angle (Elmaraghy and Devereaux 2012).
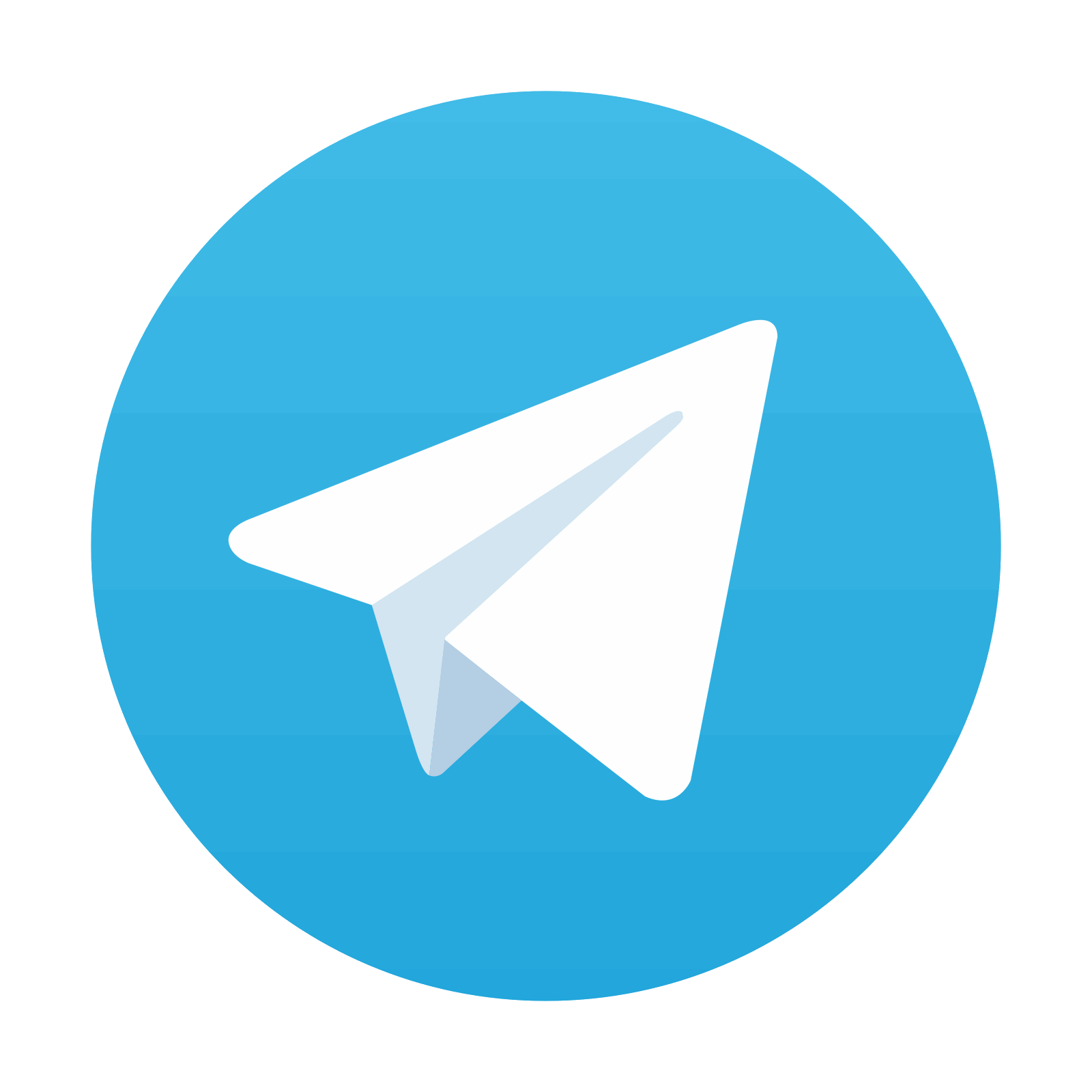
Stay updated, free articles. Join our Telegram channel

Full access? Get Clinical Tree
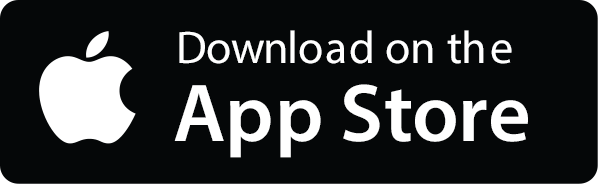
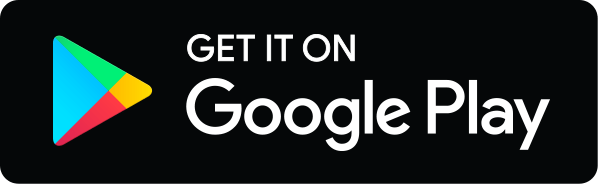