Fig. 29.1
Tibial plateau fracture. (a) AP radiograph, (b) coronal and (c) sagittal reformatted CT image, and (d) 3D reconstruction. The fracture lines at the eminentia tibia and posterolateral tibial plateau are clearly delineated by CT
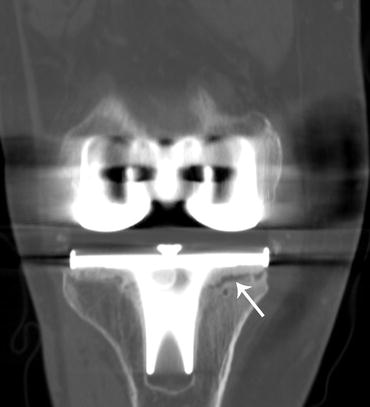
Fig. 29.2
Loosening TKP. Coronal reformatted CT image shows periprosthetic reduced bone density with translucency at the medial tibial plateau (arrow)
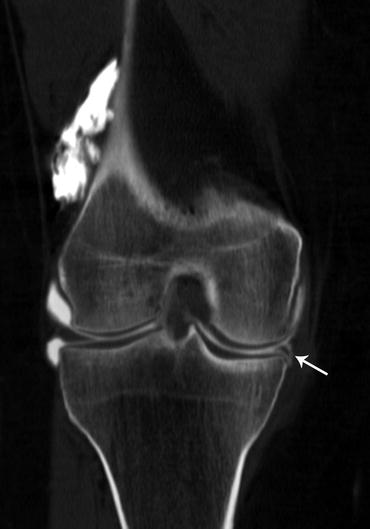
Fig. 29.3
Recurrent tear after medial partial meniscectomy. Coronal reformatted CT arthrography image shows contrast leakage in medial meniscal residue (arrow)
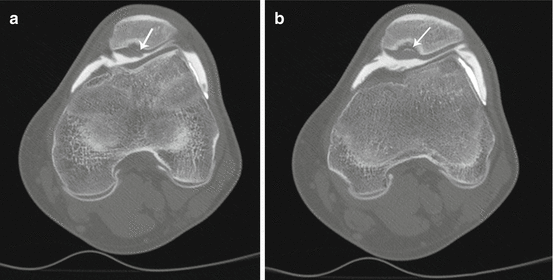
Fig. 29.4
Osteochondritis dissecans (OCD) of the patella. (a, b) Axial-reformatted CT arthrography images show OCD lesion at the patella. Cartilage fissure with contrast leakage at the base of the OCD lesion is seen (arrow)
29.2.3 Ultrasound
Ultrasound (US) is largely limited to an evaluation of the extra-articular soft tissues of the knee (Fig. 29.5). Due to the excellence of spatial resolution, US keeps its leading edge when dealing with muscle and tendon pathology around the knee (Peetrons 2002). The major indication for US in clinical practice is evaluating overuse conditions of the extensor apparatus or affirming a clinically suspected Baker’s cyst. High-frequency (13.5 MHz) linear-array probes are used to perform US examinations of the knee. US palpation is a very valuable tool, trying to find the point of maximal tenderness or swelling (Peetrons 2002). Dynamic US study may be very helpful to assess a “snapping” sensation that is felt by the patient during knee movement. Major advantages of US are its low cost, wide availability, short examination times, and lack of radiation exposure. An addition of color power Doppler imaging to US has allowed for the noninvasive study of blood flow and vascularization within anatomic structures and lesions. Although initial studies (Weinberg et al. 1998) reported that increased tendon vascularization is typically correlated with clinical symptoms, more recent studies have reported the opposite (Tol et al. 2012). Furthermore, US provides image guidance for interventional procedures such as drainage of fluid collections and cysts. The trade-off for high-frequency, linear transducers is their small, static scan field. Extended field-of-view ultrasound (EFOVS) overcomes this disadvantage by generating a panoramic image (Fig. 29.6). With this technique, sequential registration of images along a broad examination region and their subsequent combination into an image of larger dimension and format is obtained (Weng et al. 1997). Disadvantages of US include operator dependency, selective and often incomprehensible documentation, and the inability to evaluate the articular structures of the knee joint.
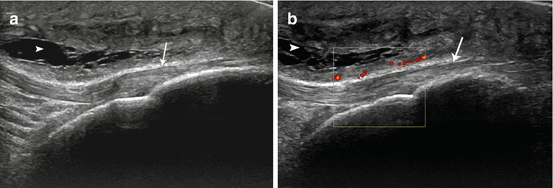
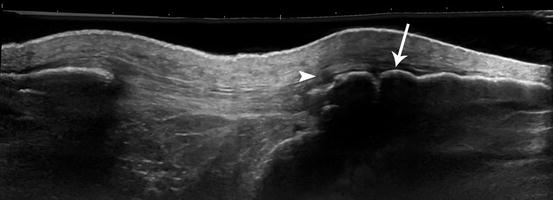
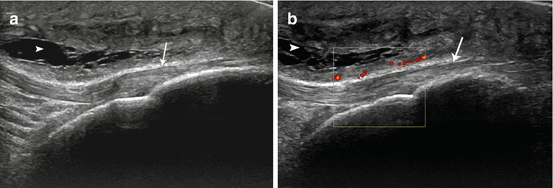
Fig. 29.5
Superficial infrapatellar bursitis. Longitudinal grayscale (a) and color Doppler (b) US images of infrapatellar region demonstrate thickening of prepatellar soft tissues with increased vascularity and distended infrapatellar bursa (arrowhead). The patellar tendon is normal (arrow)
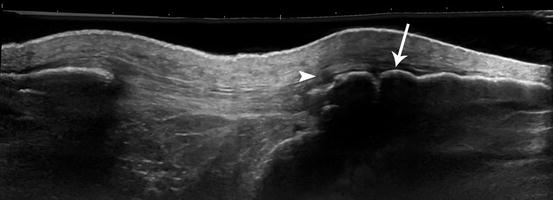
Fig. 29.6
Longitudinal extended field-of-view US image of the infrapatellar region reveals thickening of distal patellar tendon and adjacent soft tissues (arrowhead). Note bony hypertrophy at the tibial tuberosity (arrow) in keeping with chronic Osgood-Schlatter disease
29.2.4 Magnetic Resonance Imaging
The noninvasiveness of magnetic resonance imaging (MRI) combined with its relatively low cost has led to its acceptance by the orthopedic community, and MRI of the knee is the most frequently ordered MR examination of the musculoskeletal system (Farjoodi et al. 2010). Numerous diagnostic studies have compared MRI and arthroscopy of the knee, and most have shown good diagnostic performance of MRI in detecting internal derangements of the knee (Oei et al. 2003). MRI not only contributes to diagnosis but also serves as an important guide to treatment planning and prognostication (Milewski et al. 2011).
Diagnostic knee MRI can be performed using a variety of magnet designs (closed bore, whole body, open whole body, dedicated extremity). Also, a variety of magnetic field strengths can be used but the current standard for clinical knee imaging is 1.5 tesla (T). The patient is positioned supine with the affected knee completely or near completely extended. A local coil is positioned to provide adequate anatomic coverage. Typically, an extremity knee coil that completely surrounds the knee is used to ensure maximal and uniform signal-to-noise ratio (SNR) across the entire image.
A complete MR exam of the knee requires that images be obtained in the axial, sagittal, and coronal planes. Although techniques for knee MRI vary widely, a typical imaging protocol uses a field of view (FOV) of 160 mm or smaller, a slice thickness in the sagittal and coronal plane of 3 mm or less, and an imaging matrix with at least 140 steps in the phase direction and 256 steps in the frequency direction for 2D imaging (Tuite et al. 2012). The use of a contrast agent (e.g., DOTAREM®) is only indicated when dealing with a tumoral or tumorlike mass, in cases of knee inflammation, or as part of arthrography (performed only for postoperative knee imaging when conventional MRI reveals equivocal findings).
Knee MRI can be performed with a wide variety of pulse sequences. Most commonly, the proton-density (PD) and T2-weighted 2D turbo spin-echo (TSE) sequence and the T1-weighted 3D gradient-recalled (GRE) sequence are used for routine imaging of the knee (Recht et al. 2005; Kijowski 2010; Trattnig et al. 2009).
TSE sequences are advantageous in daily clinical practice because they allow assessment of the menisci, ligaments, and bony structures in addition to the articular cartilage, in a relatively short time (Recht et al. 2005). Fat suppression (FS) TSE images are most sensitive to bone marrow and soft tissue edema or joint effusion. Different FS techniques are available (spectral FS, Dixon method, inversion recovery technique), but in our institution, we prefer the spectral FS technique because of its better SNR and spatial resolution compared to the other FS techniques (Fleckenstein et al. 1991). Also, for good detection of fluid with preservation of anatomic detail and good differentiation between joint fluid and articular cartilage, we include an FS TSE intermediate-weighted sequence (TR/TE = 3,500/30–35 ms) in at least one imaging plane in our routine knee MR protocol (Fig. 29.7). Although 2D TSE acquisitions provide excellent tissue contrast and high in-plane spatial resolution, they may suffer from partial volume averaging artifacts because they use relatively thick slices with small gaps between the slices, possibly obscuring pathology (Hargreaves et al. 2003; Link et al. 2007). To overcome this disadvantage, 3D TSE sequences with isotropic resolution have recently been developed and are now commercially available on many MR vendor platforms. These sequences include 3D fast spin-echo (FSE) cube (GE Healthcare), 3D Fourier transform (FT, Philips Medical Systems), and sampling perfection with application-optimized contrasts using different flip-angle evolutions (SPACE, Siemens Medical Systems) (Kijowski and Gold 2011; Gold et al. 2007; Notohamiprodjo et al. 2009; Ristow et al. 2009). The advantage of these new 3D TSE acquisitions is their capability of mimicking the contrast properties of conventional 2D TSE PD-weighted acquisitions (Gold et al. 2007). In addition, high quality MPR images may be created in any orientation from the volumetric source data (Fig. 29.8). Although the first clinical results on the diagnostic performance of 3D isotropic resolution TSE sequences were encouraging, it remains uncertain whether a single 3D TSE acquisition has potential for replacing the multiple conventional 2D acquisitions currently used (Notohamiprodjo et al. 2009; Jung et al. 2009; Kijowski et al. 2009, 2012b; Subhas et al. 2011; Van Dyck et al. 2012b).
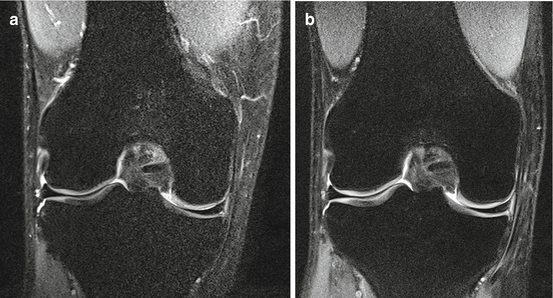
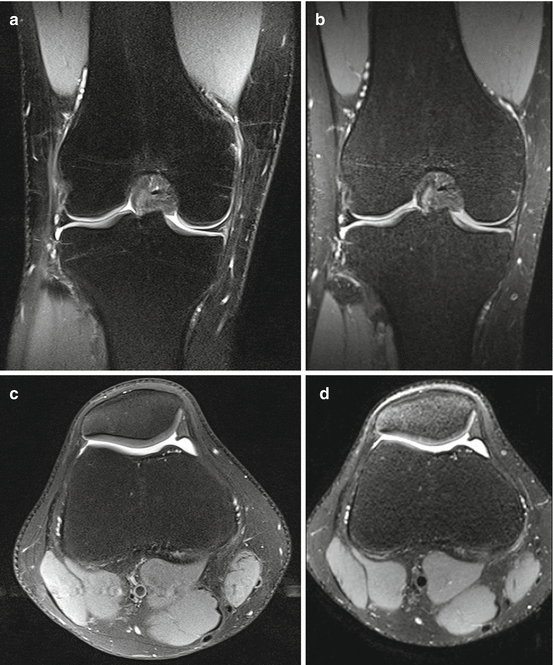
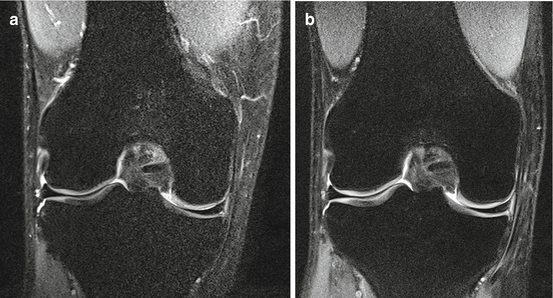
Fig. 29.7
Coronal fat-suppressed intermediate-weighted MR images obtained at 1.5 T (a) and 3 T (b). Better delineation of articular cartilage (intermediate signal intensity) from joint fluid (high signal intensity) is seen at 3 T compared to 1.5 T
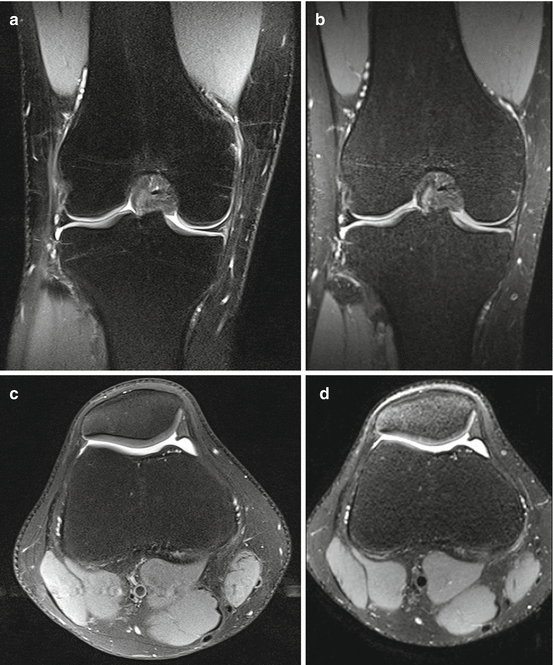
Fig. 29.8
Conventional 2D versus isotropic 3D fat-suppressed intermediate-weighted turbo spin-echo (TSE) images of the knee at 3 T. Conventional 2D TSE images in the coronal (a) and axial (c) plane with corresponding 3D TSE SPACE images (b, d). Note similar contrast properties of bone, cartilage, menisci, and ligaments with both 2D and 3D TSE imaging
Thin-partitioned, 3D gradient-recalled echo (GRE) sequences, either using selective FS (FS 3D SPGR) or selective water excitation (WE) (WE 3D FLASH; WE 3D DESS), also have been used to assess the knee. These sequences provide higher spatial and contrast resolution but require longer acquisition times (7–10 min) and are more vulnerable to magnetic susceptibility and metallic artifacts. Moreover, GRE images can be used for evaluating the articular cartilage of the knee joint but the menisci, ligaments, and bone marrow are only poorly visualized with this sequence (Kijowski and Gold 2011).
MRI has the disadvantage of not always being accepted well by patients, of being incompatible with dynamic maneuvers, and of not always being possible in emergency conditions. Also, specific MRI safety measures and contraindications for the use of MRI (e.g., claustrophobia, filters, stents, clips, cardiac pacemakers, etc.) must be considered.
29.3 Specific Disorders
29.3.1 Meniscus
The suspicion of a tear of the knee meniscus constitutes one of the leading indications for MRI examination of this articulation. Although arthroscopy is still considered the most reliable standard in the assessment of meniscal tears, MRI has emerged as the most important modality in the noninvasive evaluation of the knee meniscus and has proved useful as a screening procedure to avoid diagnostic arthroscopy (Milewski et al. 2011).
Normal menisci demonstrate low signal intensity on MRI because of their fibrocartilage composition. They are triangular in cross section. MR criteria used for diagnosis of meniscal tear include one or more of the following: (1) abnormal meniscal signal touching an articular surface, (2) missing meniscal tissue, and (3) displaced meniscal fragment. Because abnormal meniscal morphology (with or without displaced meniscal fragment) is less frequent, MR diagnosis of a meniscal tear usually comes down to “grading” the abnormal meniscal signal, as proposed by Lotysch et al. (1986):


Grade 0, overall low signal intensity, normal; grade I, intrasubstance globular-appearing signal not extending to an articular surface; grade II, linear increased signal not extending to an articular surface; grade III, abnormal signal extending to an articular surface. Grades I and II represent intrasubstance degeneration in an adult or prominent vascularity in a child – not a tear – and are not clinically relevant. Only an unequivocal grade III signal that is seen on 2 or more images represents an arthroscopically evident tear (Fig. 29.9). This observation has been referred to as the “two-slice-touch rule” (De Smet 2012).
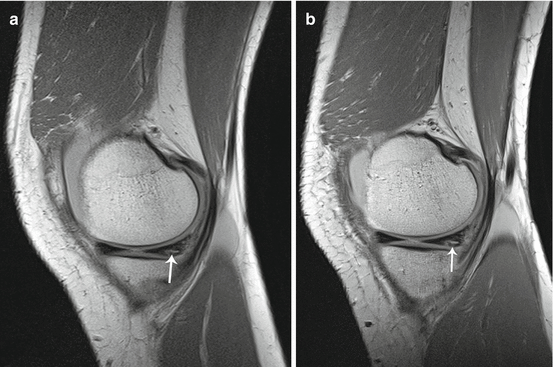
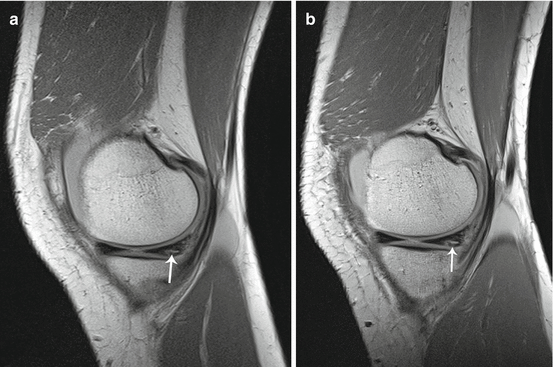
Fig. 29.9
Posterior horn medial meniscal tear. Sagittal proton density-weighted images of the knee obtained at 1.5 T (a) and 3.0 T (b) show surfacing hyperintense grade III signal in the posterior horn of the medial meniscus (arrow). Despite improved image quality at 3 T, the tear is clearly identifiable at both field strengths. Posterior horn medial meniscal tear was confirmed by arthroscopy
Meniscal tear types most commonly seen on MRI include horizontal, vertical, radial, bucket handle, flap, and complex tears (Fox 2007). Extrusion of joint fluid through a meniscal tear may give rise to a fluid collection in the parameniscal soft tissues, called a meniscal cyst. Meniscal cysts are frequently located adjacent to the posterior horn of the medial meniscus (Campbell et al. 2001).
A discoid meniscus is a large, congenitally dysplastic meniscus that has lost its normal shape and has a broad disklike configuration. Lateral discoid menisci are more common than medial discoid menisci. They are susceptible to tears and cysts (Yoo et al. 2012).
PD-weighted TSE sequences are used most frequently to examine the menisci. Although several authors have advised against the use of TSE sequences for the menisci because of image blur, more recent studies have indicated that properly optimized TSE imaging compares favorably with conventional SE imaging for this purpose (Ramnath et al. 2006).
The evaluation of menisci with MRI is a highly accurate endeavor with sensitivities ranging between 80 and 100 %, specificities ranging between 72 and 100 %, and accuracies ranging between 75 and 96 % (Oei et al. 2003). Sensitivity for diagnosing lateral meniscal tears is still lower than for the diagnosis of medial meniscal tears, particularly on the posterior horn of the lateral meniscus in cases with a background of anterior cruciate ligament (ACL) injury (Savoye et al. 2011). A meta-analysis of articles published between 1991 and 2000, including studies performed with magnets operating in a range from 0.1- to 1.5-T, revealed that diagnostic performance for meniscal tears is only modestly improved with increased magnetic field strength (Oei et al. 2003). One could suppose that with 3-T MRI, the confidence and accuracy of detecting meniscal tears will continue to increase (Gold et al. 2004; Ramnath 2006; Magee 2007; Krampla et al. 2009). Although initial reports on the detection of meniscal tears with 3-T MRI showed that 3-T imaging has a sensitivity and specificity greater than previously reported results at 1.5- T or less field strength (Magee and Williams 2006), further studies are required to determine the true impact of 3-T in evaluating the meniscus of the knee (Fig. 29.9).
29.3.2 Cruciate Ligaments
MRI is most frequently chosen to confirm the diagnosis of ACL injury and to document the extent of associated injuries.
On MRI, the normal ACL can be followed as a continuous band of low signal intensity from the femoral to the tibial attachment on the sagittal, coronal, and axial images. Oblique imaging planes may be useful to accurately depict the normal double-bundle anatomy of the ACL (Steckel et al. 2006). Tears of the ACL are primarily diagnosed on MRI on the basis of the presence of abnormalities seen in the ligament itself. Replacement of the ACL by an edematous mass with nonvisualization of its fibers and a wavy contour of the ligament is considered a sign of a complete tear. Also, if there is ligament retraction and no identifiable central ligament present, the tear is designated as complete. Hyperintense signal within the ACL substance, distortion of fibers without obvious complete discontinuity, and attenuation and/or abnormal orientation of the ACL with respect to the roof of the intercondylar notch (Blumensaat’s line) are all considered MR signs of a partial ACL tear (Moore 2002). If fiber disruption can clearly be detected in the anteromedial or posterolateral bundle of the ACL, an isolated bundle tear is reported (Van Dyck et al. 2012a). Diffuse ACL thickening with a fluidlike signal separating the longitudinally oriented fiber bundles (“celery stalk appearance”) is typical of mucoid degeneration of the ACL and should not be reported on MRI as a partial tear (Bergin et al. 2004). Secondary MR signs of ACL injury, including lateral bone bruise, anterior translation of the tibia with respect to the distal femur, uncovering of the posterior horn of the lateral meniscus, and an increased buckling of the posterior cruciate ligament (PCL), if present, are helpful signs in the diagnosis of an ACL tear (Van Dyck et al. 2012c).
Many investigators demonstrated success with MRI in the diagnosis of ACL tears, reporting sensitivities between 92 and 100 %, specificities between 85 and 100 %, and accuracies between 89 and 100 % (Moore 2002). Only few MR studies have considered complete and partial ACL tears separately, and in these studies, MRI has not been shown to be accurate in the differentiation of complete from partial ACL tears, nor were they successful in the identification of partial ACL tears (Defranco and Bach 2009; Steckel et al. 2006). For partial ACL tears, a sensitivity range of 40–75 % and specificity range of 62–89 % have been published (Umans et al. 1995). According to the meta-analysis performed by Oei et al. (2003), higher magnetic field strength significantly improves the diagnostic performance of MRI in the detection of ACL tears. Although initial reports on the detection of partial tears with 3-T MRI showed that 3-T imaging has a sensitivity comparing favorably with previously published results at 1.5-T or less field strength (Van Dyck et al. 2011), further studies are required to determine the true impact of 3-T in evaluating the ACL (Fig. 29.10).
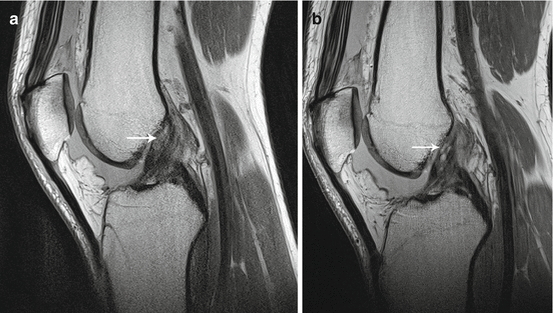
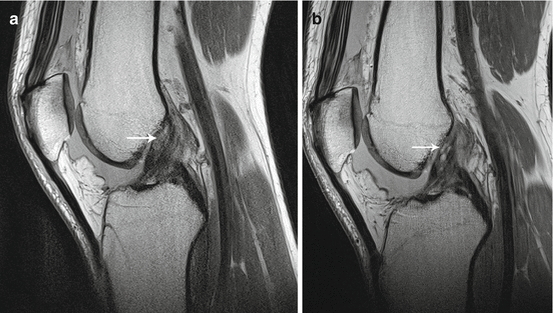
Fig. 29.10
Partial ACL tear. Sagittal proton density-weighted images of the knee obtained at 1.5 T (a) and 3 T (b) show hyperintense signal abnormality and cyst formation at the ACL origin (arrow) in keeping with partial ACL tear. Improved image quality is seen at 3 T. Partial ACL tear was confirmed by arthroscopy
Injury to the PCL is not as common as injury to the ACL. The PCL is often injured in conjunction with the ACL and, in particular, the lateral collateral ligament and the posterolateral corner (Cummings and Pedowitz 2005). This combination can lead to early osteoarthritis and failure of PCL reconstruction if the associated injuries are not addressed properly (Covey 2001). Because not all PCL injuries are apparent on clinical examination, even under general anesthesia, and the PCL may be difficult to identify at arthroscopy in the presence of an intact ACL, MRI plays an important role in the assessment of these lesions (Grover et al. 1990). The normal PCL has homogeneous low signal intensity on MRI and is typically about twice the thickness of the ACL. Intrasubstance or discrete tears of the PCL are identified commonly due to the tight synovial sheath within which the ligament is invested (Grover et al. 1990). The PCL is often injured as a result of a direct trauma to the anterior aspect of the tibia with the knee in flexion (“dashboard trauma”), and this may result in a bone contusion of the anterior aspect of the proximal part of the tibia. Therefore, the presence on MRI of such a bone contusion in the anterior tibia should prompt a detailed search for associated lesions of the PCL and posterior capsule of the knee (Fig. 29.11) (Cummings and Pedowitz 2005).
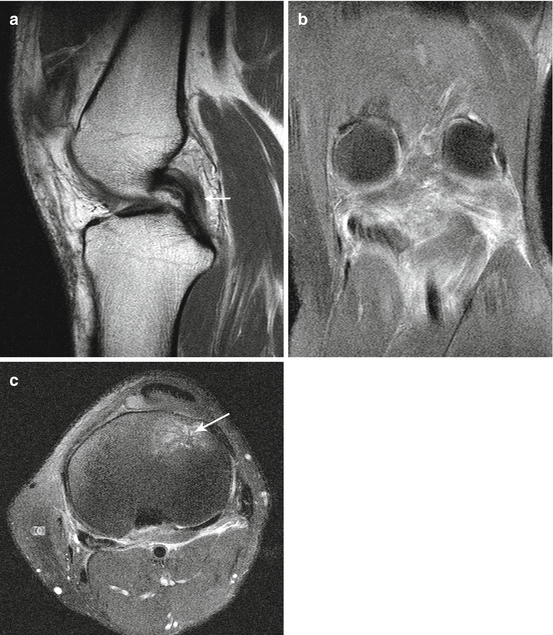
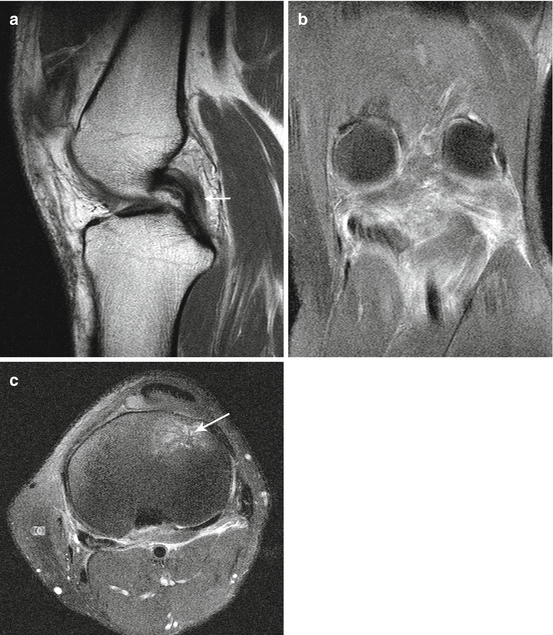
Fig. 29.11
Dashboard trauma of the knee. Sagittal proton density-weighted image (a) shows hyperintense signal in posterior cruciate ligament due to mild sprain (arrow). Coronal (b) and axial (c) fat-suppressed intermediate-weighted images show extensive edema in the posterolateral soft tissues in keeping with capsular sprain. Also note bone contusion at the anterior aspect of the tibia in (c) (arrow)
29.3.3 Articular Cartilage and Bone
Accurate assessment of the articular cartilage of the knee in patients undergoing MRI is clinically important (Milewski et al. 2011). The appearance of cartilage lesions on MRI depends on whether they are degenerative or posttraumatic in cause (Recht et al. 2005; Kijowski 2010). Early degenerative cartilage lesions appear as fibrillation or fissuring of the articular surface. As the degeneration process progresses, partial- and full-thickness cartilage defects with obtuse margins may develop. In patients with advanced osteoarthritis, cartilage lesions are typically associated with subchondral bone marrow edema (BME), presenting as ill-defined areas of high signal intensity on T2-weighted images. The presence of these subchondral bone marrow changes has been correlated with pain (Felson et al. 2001). Traumatic cartilage lesions tend to have more acutely angulated margins that may also involve the underlying subchondral bone. Cartilage flap tears or delamination injuries at the junction between the cartilage and subchondral bone may occur due to shearing forces (Recht et al. 2005). Posttraumatic BME (“contusions or bruises”) results from impaction or traction forces acting on the joint. Impaction injuries, whether resulting from direct or indirect trauma, typically appear on MRI as areas of extensive BME. The traction or avulsion BME pattern is less extensive. Moreover, the avulsed bone fragment may be very difficult to detect on MRI. In most instances, a small avulsion is far better demonstrated on CR or CT. Chronic repetitive stress injuries about the knee typically involve the tibia. MRI has become the imaging modality of choice not only for diagnosing these lesions but also to grade the severity of the stress injury, thereby assisting in the clinical management of the patient (Kijowski et al. 2012a).
The most common sequences used in clinical practice to evaluate the articular cartilage of the knee joint are 2D TSE sequences with PD/T2-weighted or intermediate-weighted contrast (Recht et al. 2005; Kijowski 2010; Roemer et al. 2011). Various 3D imaging sequences also have been used to evaluate the articular cartilage of the knee joint (Hargreaves et al. 2003; Kijowski and Gold 2011). These 3D sequences can be broadly divided into dark-fluid (e.g., spoiled gradient-recalled echo [SPGR], fast low-angle shot [FLASH]) and bright-fluid (e.g., dual echo in the steady state [DESS], driven equilibrium Fourier transform [DEFT]) sequences on the basis of the signal intensity of the synovial fluid (Fig. 29.12). Balanced steady-state free precession (SSFP) sequences are additional 3D sequences that have been used to evaluate the knee cartilage. Balanced SSFP sequences have higher cartilage SNR and greater contrast between cartilage and adjacent joint structures than 2D TSE sequences (Kijowski 2010).
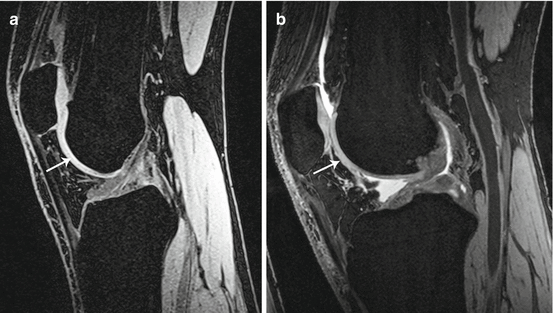
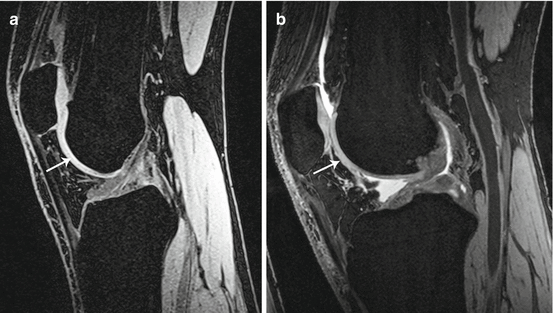
Fig. 29.12
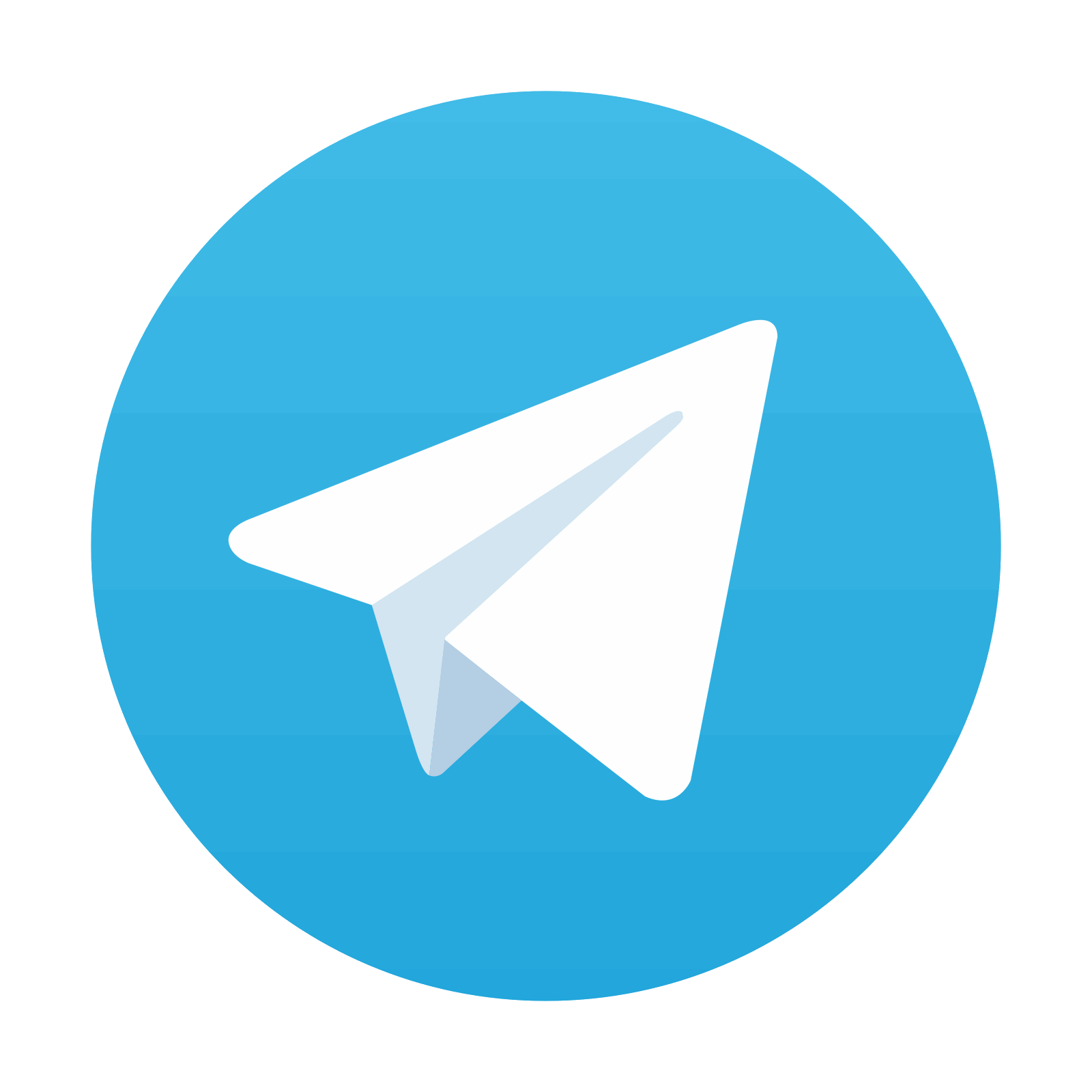
Cartilage-specific MR imaging sequences. Sagittal 3D SPGR (a) and DESS (b) images of the knee. Both “dark” (a) and “bright” (b) fluid 3D sequences clearly demonstrate hyaline cartilage of the knee (arrow)
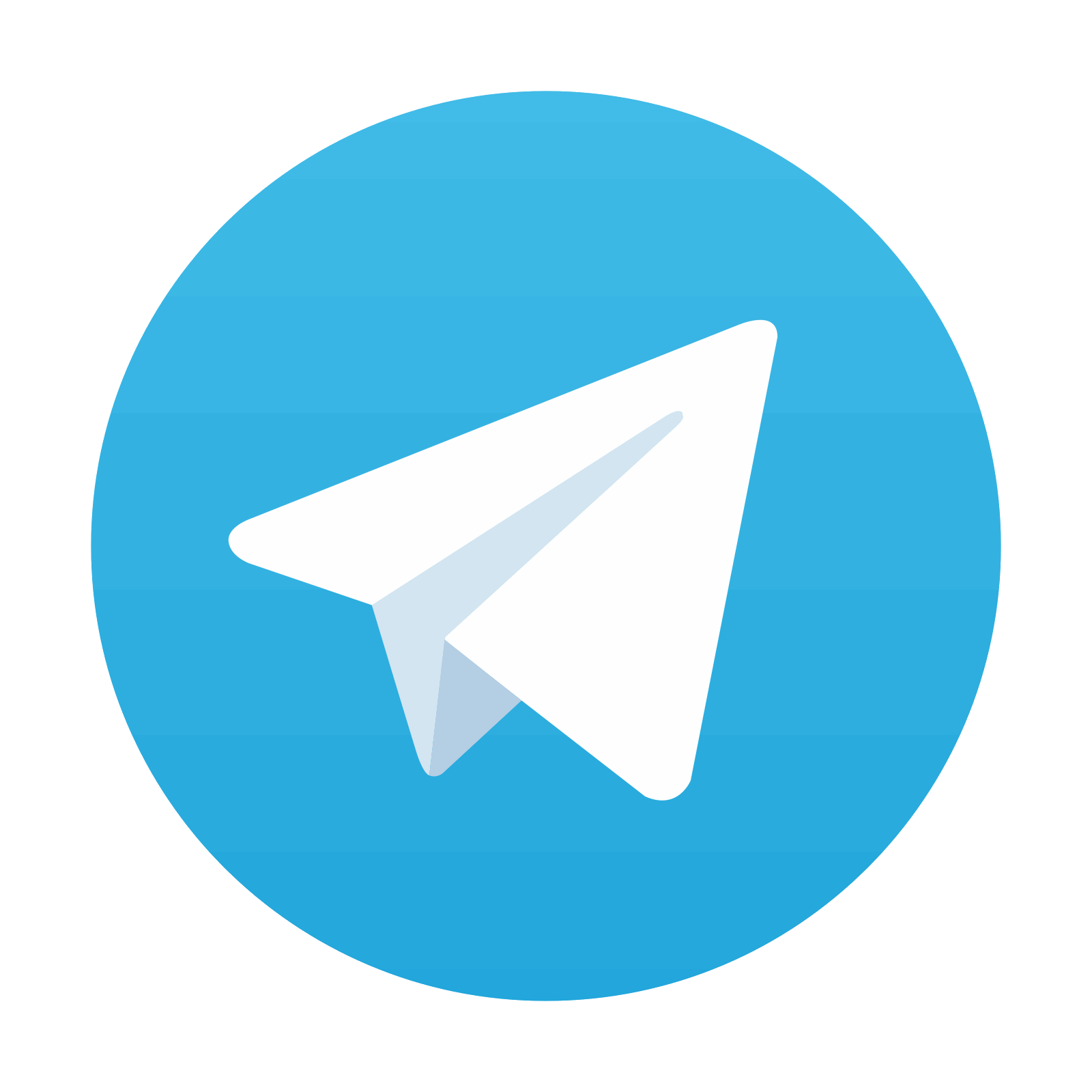
Stay updated, free articles. Join our Telegram channel

Full access? Get Clinical Tree
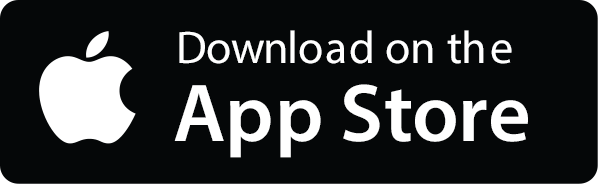
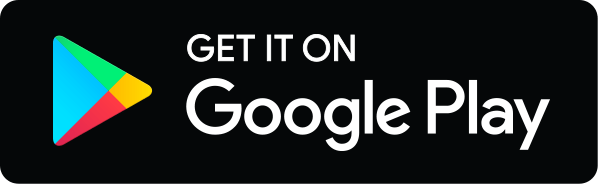
