, Jan L. M. A. Gielen2, 3, 4 and Mario Maas1
(1)
Department of Radiology, Academic Medical Center (AMC), Amsterdam, The Netherlands
(2)
Department of Radiology, Antwerp University Hospital, Wilrijkstraat 10, B2650 Edegem, Belgium
(3)
Department of Sports Medicine, Antwerp University Hospital, Wilrijkstraat 10, B2650 Edegem, Belgium
(4)
Department of Medicine, Antwerp University, Wilrijkstraat 10, B2650 Edegem, Belgium
32.1 Introduction
32.2.1 Background
32.2.2 Risk Factors
32.2.3 Clinical Picture
32.2.4 Clinical Diagnostics
32.2.5 Radiologic Imaging
32.3.1 Background
32.3.2 Risk Factors
32.3.3 Clinical Picture
32.3.4 Clinical Diagnostics
32.3.5 Radiologic Imaging
32.4 MTSS
32.4.1 Background
32.4.2 Risk Factors
32.4.3 Clinical Picture
32.4.4 Clinical Diagnostics
32.4.5 Radiologic Imaging
32.4.6 Treatment
32.5.1 Background
32.5.2 Risk Factors
32.5.3 Clinical Picture
32.5.4 Clinical Diagnostics
32.5.5 Radiologic Imaging
32.5.6 Treatment
32.6 Nerve Entrapment
32.6.1 Background
32.6.2 Clinical Picture
32.6.3 Clinical Diagnostics
32.6.4 Radiological Diagnosis
32.7 Acute Muscle Trauma
32.7.1 Muscle Strain
32.7.2 Morel-Lavallée Lesion
Abbreviations
ABI
Ankle-brachial index
BMI
Body mass index = mass (kg)/(height (m))2
CECS
Chronic exertional compartment syndrome
CT
Computed tomography
CTA
Computed tomographic arteriography
FS
Fat suppression
MIP
Maximal intensity multiplanar reconstruction
MPR
Multiplanar reconstruction
MRI
Magnetic resonance imaging
MTJ
Musculotendinous junction
MTSS
Medial tibial stress syndrome
ROM
Range of motion
SI
Signal intensity
32.1 Introduction
Lower leg injuries are a common problem in athletes. One-third of injuries in long-distance runners are lower leg injuries (Brewer and Gregory 2012). The spectrum of lower leg injuries in athletes contains a number of injuries with very similar presentations. In a retrospective review assessing 150 athletes with exercise-induced leg pain, 33 % had chronic compartment syndrome, 25 % had a stress fracture, 13 % had medial tibial stress syndrome and 10 % had a nerve entrapment syndrome (Clanton and Solcher 1994). Another study among 98 patients with recurrent lower leg pain reported medial tibial stress syndrome in 42 %, chronic compartment syndrome in 27 % and entrapment of the superficial peroneal nerve in 13 % of patients (Styf 1988). This chapter aims to offer a few key points that differentiate between these injuries both in clinical presentation and in imaging characteristics.
The differential diagnosis of (exercise-induced) lower leg pain (in athletes) includes the following disorders:
Chronic exertional compartment syndrome (CECS)
Stress fractures of the tibia or fibula
Medial tibial stress syndrome (MTSS)
Muscle strains
Nerve entrapment syndromes
Popliteal artery entrapment syndrome
Fascial defects
Referred pain from the hip or the knee
Vascular claudication
Lumbar disc herniation
Tendinitis/tendinosis
Effort-induced venous thrombosis
Neoplasm
Infection
The most common causes of lower leg pain in athletes and their preferred imaging techniques will be discussed more extensively in the paragraphs below.
32.2 Chronic Compartment Syndrome
32.2.1 Background
Chronic compartment syndrome is also known as chronic exertional compartment syndrome (CECS). The pathophysiology of chronic exertional compartment syndrome is multifactorial (Brewer and Gregory 2012). It is thought to differ from acute compartment syndrome in the sense that in CECS tissue pressures are elevated, but not to the extent that tissue ischaemia occurs, as is the case in acute compartment syndrome (Bong et al. 2005).
Some authors have postulated that in CECS increased compartmental pressure is caused by a combination of physiological factors such as increased blood flow, oedema and muscle volume during strenuous exercise, combined with decreased compliance of the fascia surrounding the compartment (Bong et al. 2005). It is still unclear, however, if pain in CECS is caused by subsequent decreased arterial blood supply and relative ischaemia or rather by periosteal nerve compression (Bong et al. 2005).
The lower leg is divided into multiple compartments containing muscles and other anatomic structures and separated by fascia. The contents of these compartments are described below:
Anterior compartment: tibialis anterior, extensor hallucis longus, extensor digitorum longus and peroneus tertius muscles, anterior tibial artery and vein and deep branch of the common peroneal nerve.
Lateral compartment: peroneus longus and peroneus brevis muscles and superficial branch of the common peroneal nerve.
Superficial posterior compartment: soleus, plantaris and gastrocnemius muscles and the sural nerve.
Deep posterior compartment: flexor digitorum longus, flexor hallucis longus and popliteus muscles, posterior tibial artery and vein and the tibial nerve.
The tibialis posterior muscle has its own fascial enclosure and is sometimes considered a separate ‘fifth compartment’ (Davey et al. 1984).
32.2.2 Risk Factors
Apart from the main patient group of long-distance runners, chronic compartment syndrome is also common in athletes performing sports that require extensive running, such as soccer, football and basketball (Bong et al. 2005). Several studies have observed fascial defects in 20–60 % of patients with chronic compartment syndrome (Pedowitz and Gershuni 1995). These defects are commonly found in the fascia surrounding the lateral compartment (Edwards et al. 2005). Muscle tissue can herniate through a fascial defect, and some authors have considered such herniations to be an indicator of increased compartmental pressure (Blackman 2000).
However, fascial herniations have also been shown to occur in patients without chronic compartment syndrome (Bong et al. 2005).
32.2.3 Clinical Picture
Patients suffering from chronic compartment syndrome commonly present with a dull ache in the leg within the first thirty minutes of exercise. This is then followed by a cramping, burning or aching pain and tightness occurring with continuing exercise (Pedowitz and Gershuni 1995; Bong et al. 2005). Pain often increases during running and quickly disappears when exercise is ceased. Most often, pain is located over the involved compartment(s). In the vast majority of patients, symptoms are bilateral (Bong et al. 2005). Some patients may experience neurological symptoms as well, such as weakness or sensory abnormalities (Blackman 2000; Bong et al. 2005).
32.2.4 Clinical Diagnostics
Compartment hydrostatic pressure testing is the gold standard for the diagnosis of chronic compartment syndrome, requiring pre-exertional and post-exertional measurements (Bong et al. 2005; Edwards 2005; Brewer and Gregory 2012). Exact methods and techniques and normal values of measuring pressure vary, but measurements should always be done in rest, followed by measurement after exercise similar to the level of exercise in the athlete’s regular routine (Bong et al. 2005). Pedowitz et al. have described criteria for these pressure measurements to be applied in clinical practice (Pedowitz and Gershuni 1995). However, compartment pressure testing is an invasive diagnostic method, which explains why imaging modalities such as MRI and ultrasound also have a valuable role in the diagnosis of chronic compartment syndrome.
32.2.5 Radiologic Imaging
The additional value of radiologic imaging in CCS is limited.
32.2.5.1 Magnetic Resonance Imaging
In two studies, MRI has been shown to detect an increased T2-weighted signal in the affected compartment in patients with chronic anterior compartment syndrome, where in one study this increased signal disappeared after fasciotomy (Eskelin et al. 1998; Verleisdonk et al. 2001). One study describes significant post-exertional MRI findings in CCS at forearm muscles in motocross racers (Gielen et al. 2009). However, the interpretation of increased signal intensity (SI) should be interpreted carefully as increased muscle signal is also described in non-symptomatic racers. However, more research is necessary before MRI can be applied universally as a method for detecting increased compartmental pressure (Bong et al. 2005).
32.2.5.2 Ultrasound
The use of ultrasound in the diagnosis of chronic compartment syndrome has been evaluated on a small scale (Rajasekaran et al. 2013). The diagnosis is based on the anterior compartment thickness as measured on ultrasound images (Rajasekaran et al. 2013). Although ultrasound is a promising non-invasive diagnostic modality, general criteria do not yet exist for the diagnosis of chronic compartment syndrome.
32.2.5.3 Other Imaging Methods
32.2.5.4 Treatment
The conservative treatment of chronic compartment syndrome consists of sport cessation or massage therapy, but these are not considered successful options (Bong et al. 2005). A more effective treatment but invasive option is surgical fasciotomy of the involved compartments of the leg (Bong et al. 2005).
32.3 Lower Leg Stress Fractures
32.3.1 Background
In literature, various terms are used for describing stress fractures, such as insufficiency, fatigue, incremental, low-trauma and march fractures (Liong and Whitehouse 2012). The cause is thought to be excessive, prolonged or recurrent loading of the bone producing a misbalance between bone resorption and bone formation processes, which leads to stress injury (Lassus et al. 2002). If overuse with microtrauma is continued, eventually a stress fracture can develop (Lassus et al. 2002; Brewer and Gregory 2012). As much as 80–95 % of all stress fractures are located in the lower extremity, with the majority of these fractures involving the tibia and a minor part concerning the fibula (Brewer and Gregory 2012). Stress fractures of the tibia can be either transverse or longitudinal (Liong and Whitehouse 2012). Transverse fractures are more common, whereas longitudinal fractures are seen in only 10 % of tibial stress fractures. Tibial stress fractures usually affect the distal two-thirds of the posteromedial tibia, categorised as low-risk stress fractures, compared to anterior cortex stress fractures, which are considered high risk (Liong and Whitehouse 2012). Stress fracture of the fibula occurs most often in the lower third part of the fibula, just proximal to the attachment of the tibiofibular ligament (Fredericson et al. 2006).
32.3.2 Risk Factors
Stress fractures of the lower leg are common in military recruits, dancers and athletes, especially long-distance runners. Females have a four times higher risk of a stress injury in general (Lassus et al. 2002). Other risk factors for stress fractures include Caucasian race, irregular menses, eating disorders, osteoporosis or osteopenia, and previous stress fractures (Lassus et al. 2002; Edwards 2005). Additionally, multiple concurrent stress fractures are not unusual, as is seen in 10 % of runners and in 20 % of female athletes (Sullivan et al. 1984; Bennell and Brukner 1997).
32.3.3 Clinical Picture
At first, patients experience only pain during exercise, but later on pain is also present in rest and in some cases even at night (Lassus et al. 2002; Edwards 2005; Fredericson et al. 2006). Swelling of the surrounding soft tissues may be present (Lassus et al. 2002).
On physical examination, the leg commonly appears normal. Palpation or tapping at the site of fracture can produce tenderness if extensive bone marrow involvement is present (Fredericson et al. 1995). In a third of patients, the posterior part of the tibia can be painful (Fredericson et al. 2006). Pain can be accompanied by erythema or localised swelling of the leg (Edwards 2005). The bony tenderness can be more discrete than in chronic compartment syndrome or MTSS (Bong et al. 2005). Vibratory pain at the site/location of the stress fracture is thought to be able to confirm the diagnosis (Edwards 2005).
32.3.4 Clinical Diagnostics
Imaging has an important role in the diagnosis of lower leg stress fractures, especially because early identification of stress fractures is essential in order to prevent long-term complications.
In some cases, the diagnosis of stress fracture can be made exclusively based on patient history and physical examination. In inconclusive cases, however, imaging can provide additional information that is essential for the diagnosis. The imaging modalities used most commonly in the diagnostic workup of lower leg stress fractures are plain radiographs, CT, MRI and bone scintigraphy.
32.3.5 Radiologic Imaging
32.3.5.1 Conventional Radiography
In the diagnostic workup of (chronic) leg pain in athletes, plain radiographs are mainly used to rule out fatigue fractures (Bong et al. 2005). However, false negatives are not uncommon, and because of this low sensitivity, X-ray is not decisive in the diagnosis of fatigue fractures of the lower leg (Lassus et al. 2002; Beck et al. 2012). Especially in early fatigue fractures, plain radiographs are usually negative, and sensitivity can be as low as 10 % (Brewer and Gregory 2012). Additionally, radiographic signs only become visible after 2–12 weeks after the onset of symptoms, if at all (Lassus et al. 2002; Edwards 2005). Nevertheless, radiography is considered the imaging option of choice when suspecting a stress fracture of the lower leg to rule out other bony pathology.
The first sign of a stress fracture on radiography is the ‘grey cortex sign’, a grey-looking hypodense area seen in the bone cortex, which is thought to be related to the resorption phase of bone remodelling (Mulligan 1995) (Fig. 32.4a). Subsequently, new periosteal bone formation and endosteal bone thickening can be observed in this area (Lassus et al. 2002; Fredericson et al. 2006) (Fig. 32.1). A fracture line, if at all present, can be visible as a radiolucent line in compact cortical bone or as a sclerotic line in cancellous bone. Especially the midanterior cortex should be thoroughly evaluated for a subtle lucency, labelled ‘the dreaded black line’, which can indicate the presence of a midanterior cortex tibial stress fracture (Edwards 2005).
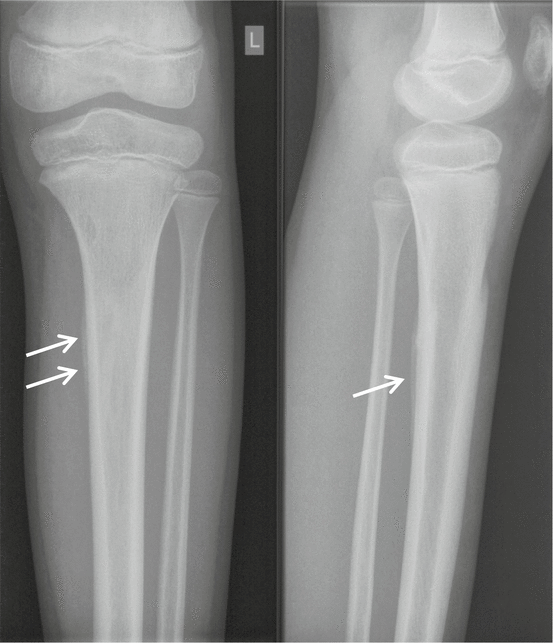
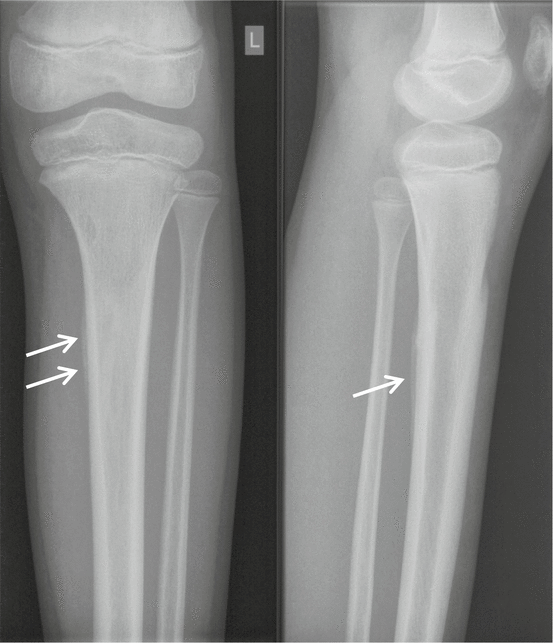
Fig. 32.1
A 9-year-old boy with exertion-related chronic tibia shaft pain at the left side. Radiograph demonstrates unilamellar calcified periosteal reaction at the medial and posterior aspect of the proximal third of the tibia diaphysis (arrows). No fracture signs are present
Other signs of stress fracture on conventional imaging include osteopenia, endosteal reaction, vague cortical margin and, in advanced stages, partial or complete fracture (Edwards 2005; Fredericson et al. 2006) (Fig. 32.2).
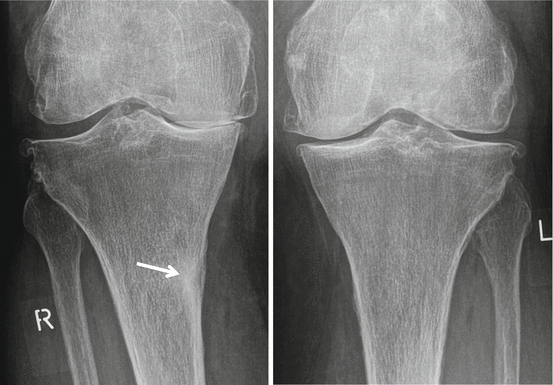
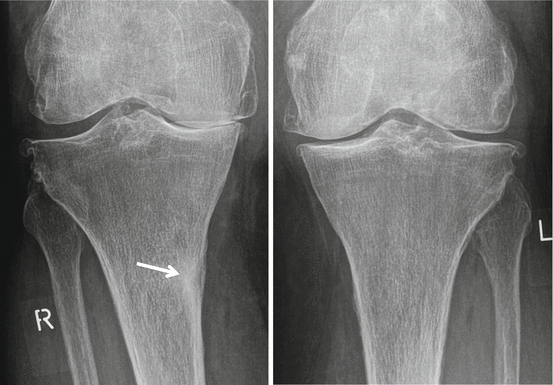
Fig. 32.2
A 69-year-old woman with stress fracture at the right proximal tibia diaphysis. Left side for comparison. Demonstration of cortical osteopenia, periosteal and endosteal reaction with sclerosis at the endosteal lining (arrow)
Transverse stress fractures of the tibia are identified as a transverse fracture line, perpendicular to the bone cortex, but in many cases no abnormalities are visible at all (Lassus et al. 2002). Longitudinal stress fractures of the tibia can be difficult to recognise on conventional radiographs because of the lengthwise fracture line orientation (Lassus et al. 2002). In fibular stress fractures, the first sign on radiographs is a hazy periosteal reaction, which is followed by callus or a fracture line in persistent disease (Lassus et al. 2002).
32.3.5.2 Computed Tomography
Computed tomography (CT) is used less frequently than radiography in the diagnosis of lower leg stress fractures, but it is able to differentiate stress fractures from stress reactions. This can aid in the exclusion of other causes of stress reaction such as malignancy (Brewer and Gregory 2012). Although CT is considered less sensitive than X-ray, longitudinal and spiral fracture lines are better visible on CT than on conventional radiographs (Lassus et al. 2002). Additionally, CT is able to depict osteopenia as a first sign of fatigue damage of the cortical bone and can detect bone loss better than X-ray (Gaeta et al. 2005). This is particularly useful in the diagnosis of stress fractures, because osteopenia is the earliest sign of fatigue injury to bone cortex (Gaeta et al. 2005). In certain cases, CT can even distinguish fractures lines that are not visible on MRI (Lassus et al. 2002).
32.3.5.3 Magnetic Resonance Imaging
Several authors have found MRI with fat suppression to be useful or promising in the diagnosis and grading of lower leg stress fractures (Fredericson et al. 1995). MRI can often detect bone marrow oedema within days of the onset of symptoms and is more sensitive and specific than CT or bone scintigraphy in diagnosing stress fractures of the tibia, with a reported sensitivity of 88 % and a specificity of 100 % (Fredericson et al. 1995; Ishibashi et al. 2002; Gaeta et al. 2005; Brewer and Gregory 2012). Because of these characteristics, MRI is increasingly popular in the workup of suspected lower leg stress fractures. However, this also implies that MRI is most useful during the first 3 weeks of symptoms, because the oedema disappears before signs of new bone formation appear (Lassus et al. 2002). Abnormalities on MRI have been correlated to a longer recovery period in bone stress injuries in general (Arendt et al. 2003).
Especially in cancellous bone stress injuries, bone marrow oedema is the primary sign of injury, which is not visible on conventional radiographs or CT (Gaeta et al. 2005). In both cancellous and cortical stress injuries, MRI with T2-weighted FS or STIR images is the imaging method of choice (Gaeta et al. 2005) (Figs. 32.3 and 32.4). The amount of bone marrow oedema is commonly more extensive than in medial tibial stress syndrome, and, in severe cases, a fracture line can be seen (Lassus et al. 2002). In fibular stress fractures, radiographs are usually positive, but MRI can show earlier abnormalities such as periosteal or bone marrow oedema and even fracture lines (Bergman and Fredericson 1999).
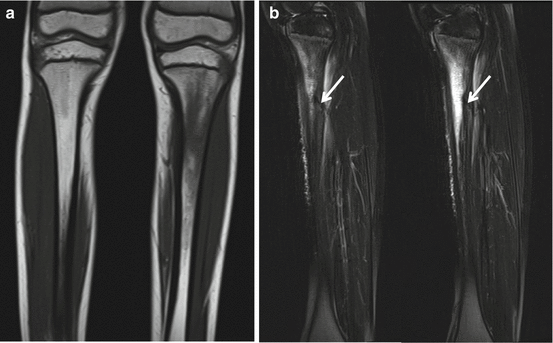
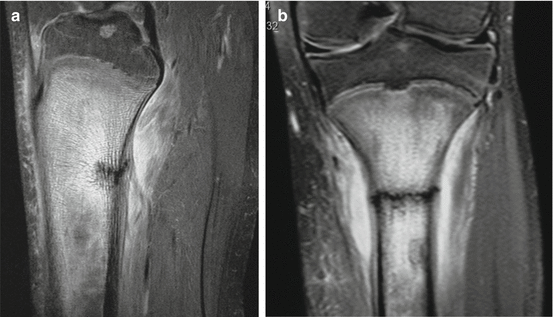
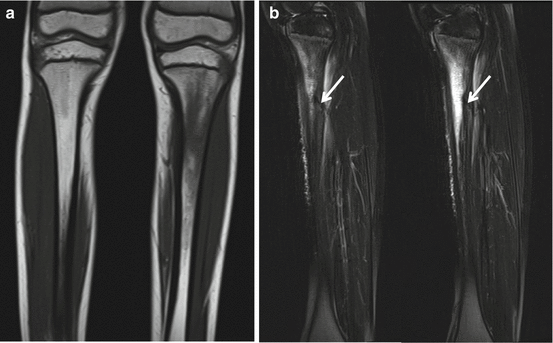
Fig. 32.3
MRI, same patient as 32.1. (a) coronal T1-WI left tibia with right tibia for comparison. Decreased SI at the medulla of the tibia diaphysis – proximal third at the left tibia – with thickening of the periosteum and cortical bone at the medial aspect. (b) Sagittal T2-WI with fat suppression. Increased SI at the medulla and posterior periosteum and periosseous soft tissues with demonstration of cortical fracture line with low SI (arrows). Thickening of the cortex related to ossified periosteal reaction
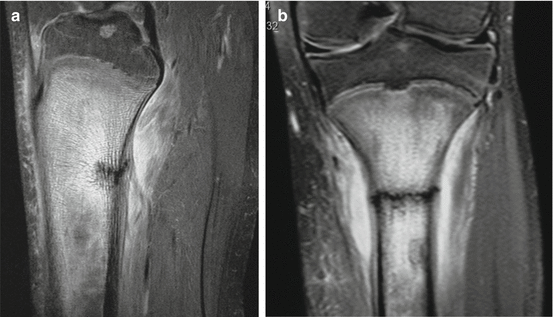
Fig. 32.4
Transverse fatigue fracture of the proximal right tibia. MRI of the right knee with sagittal (a) and coronal (b) T2-WI with FS demonstrating an extensive area with bone marrow oedema and hypointense fracture line with transverse orientation at the posterior third of the proximal tibia diaphysis. Periosteal new bone formation with multilamellar character is demonstrated at the posterior corticalis on sagittal image (a). Soft tissue oedema is surrounding the proximal metaphyseal and diaphyseal parts of the tibia
Despite these diagnostic advantages, MRI should still be interpreted with caution. A clear fracture line is confirmative of the diagnosis of stress fracture, but the presence of solely oedema in the absence of a fracture line does not rule out other stress reaction of the bone (Lassus et al. 2002) (Fig. 32.3). In contradistinction with bone marrow oedema, fracture lines at the spongious bone are easier documented on T1-WI compared to T2-WI with FS (Fig. 32.5). Furthermore, in a study by Bergman et al., 43 % of 21 asymptomatic runners were found to have abnormalities suggesting stress reaction on MRI of the lower leg, while none developed a stress injury after 12 months (Bergman et al. 2004). This is interpreted as physiologic increased bone remodelling and production in line with Wolff’s law, with adaption of the bone turnover of a healthy person to increased load resulting in increased strength and ability to resist higher loading. This is specifically the case in sports with increased activity or training. MRI findings should thus always be combined with the clinical picture.
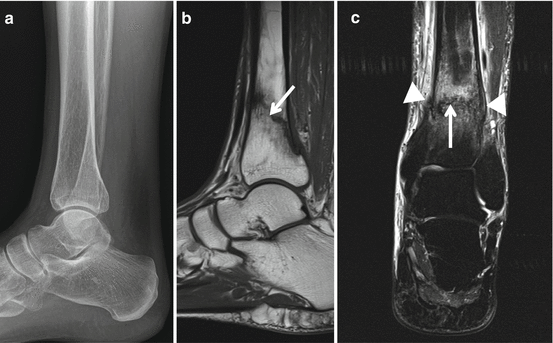
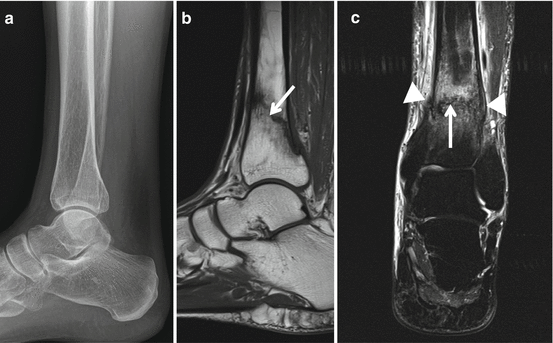
Fig. 32.5
Radiograph and MRI of a 54-year-old female with fatigue fracture at the left distal tibia meta- to diaphysis. No signs of stress fracture are observed on radiograph (a). MRI examination is performed 2 months later with sagittal T1-WI (b) and coronal T2-WI with fat suppression (c). Hypointense fracture line is easier documented on T1 compared to T2-WI (arrow). Complete fracture with medial translation over 5 mm is seen on the coronal image. Calcified periosteal reaction with low SI is demonstrated on the medial and lateral aspect of the fracture line (arrowheads). Non-calcified periosteal reaction and adjacent soft tissue oedema with increased SI on T2-WI FS are demonstrated. Discrimination of ossified and not ossified periosteal reaction is not possible on T1-WI
Several studies have compared MRI with scintigraphy, concluding that both are useful imaging modalities for the early diagnosis of lower leg stress fractures (Fredericson et al. 1995). The sensitivity of MRI in diagnosing stress injuries is similar to that of bone scintigraphy, but the specificity is higher (Lassus et al. 2002).
32.3.5.4 Ultrasound
The value of ultrasound in the diagnosis of lower leg stress fractures is very limited. In some cases, periosteal or muscle oedema, a cortical fracture line or callus can be seen (Kiuru et al. 2004) (Fig. 32.6).
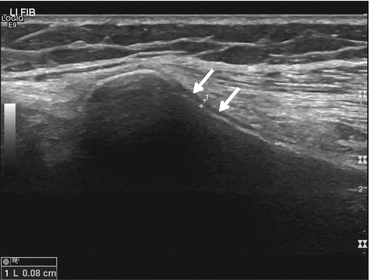
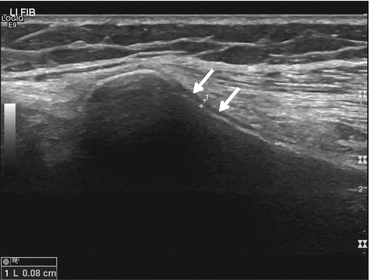
Fig. 32.6
A 20-year-old female basketball player with chronic exertional pain at the level of the posterolateral knee. Longitudinal ultrasound examination at the level of the proximal third of the left fibula metaphysic reveals hyposonant thickening of the periosteum (arrow) with regular cortical margin
32.3.5.5 Grading Systems
Based on conventional radiographs, CT, MRI and nuclear imaging methods, grading systems have been developed for the diagnosis and staging of stress fractures (Beck et al. 2012). In 1989, Jones et al. developed a radiological classification of bone stress injuries (Jones et al. 1989). Fredericson et al. developed a classification of bone stress injuries using MRI (Fredericson et al. 1995). Gaeta et al. described criteria for the diagnosis of early tibial stress injuries on CT and MRI (Gaeta et al. 2005). Recently, Kaeding et al. have introduced a classification of stress fractures (Kaeding and Miller 2013). Arendt et al. developed a grading system in 2003 (Arendt et al. 2003) (Table 32.1).
Table 32.1
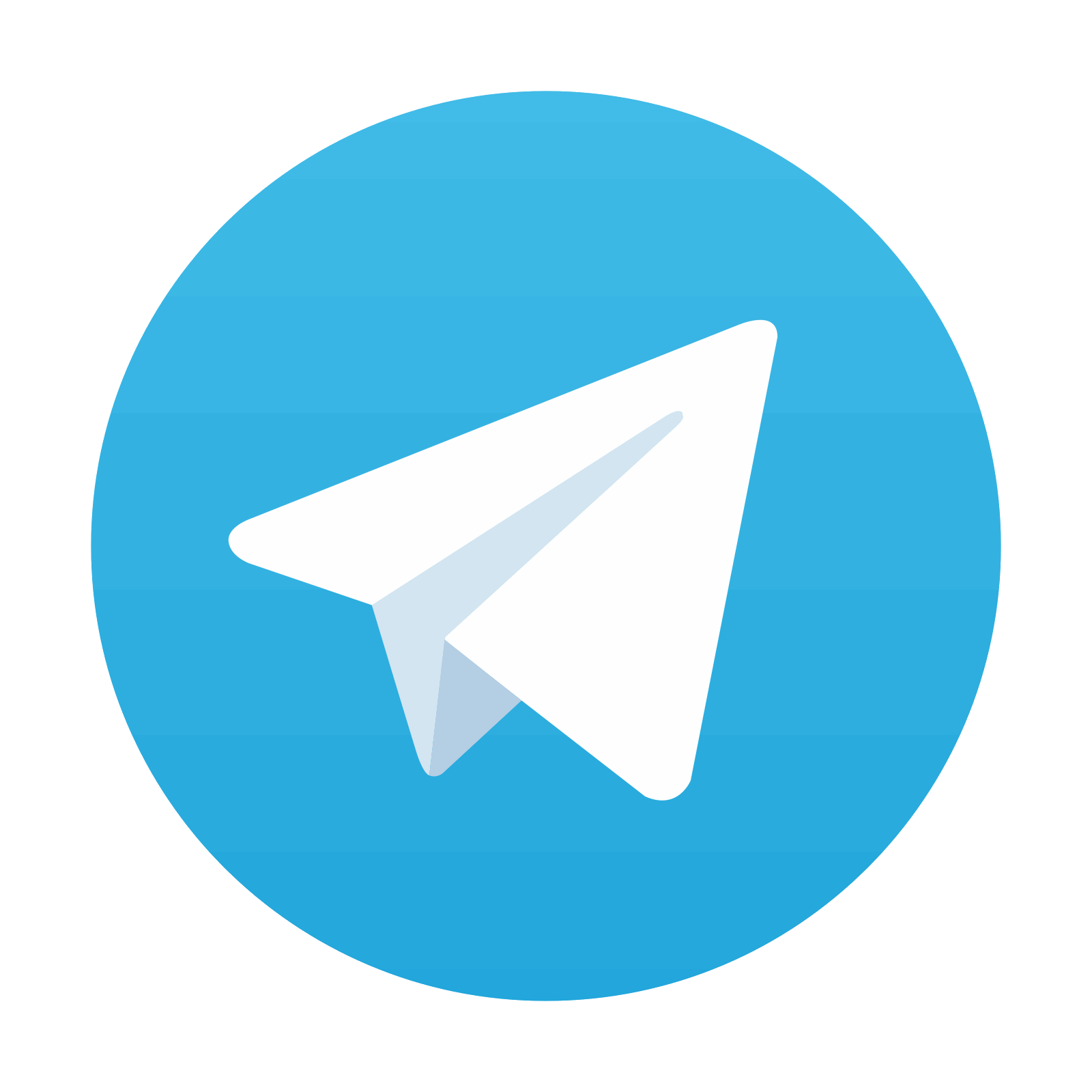
Imaging classification by Kaeding et al. (2013)
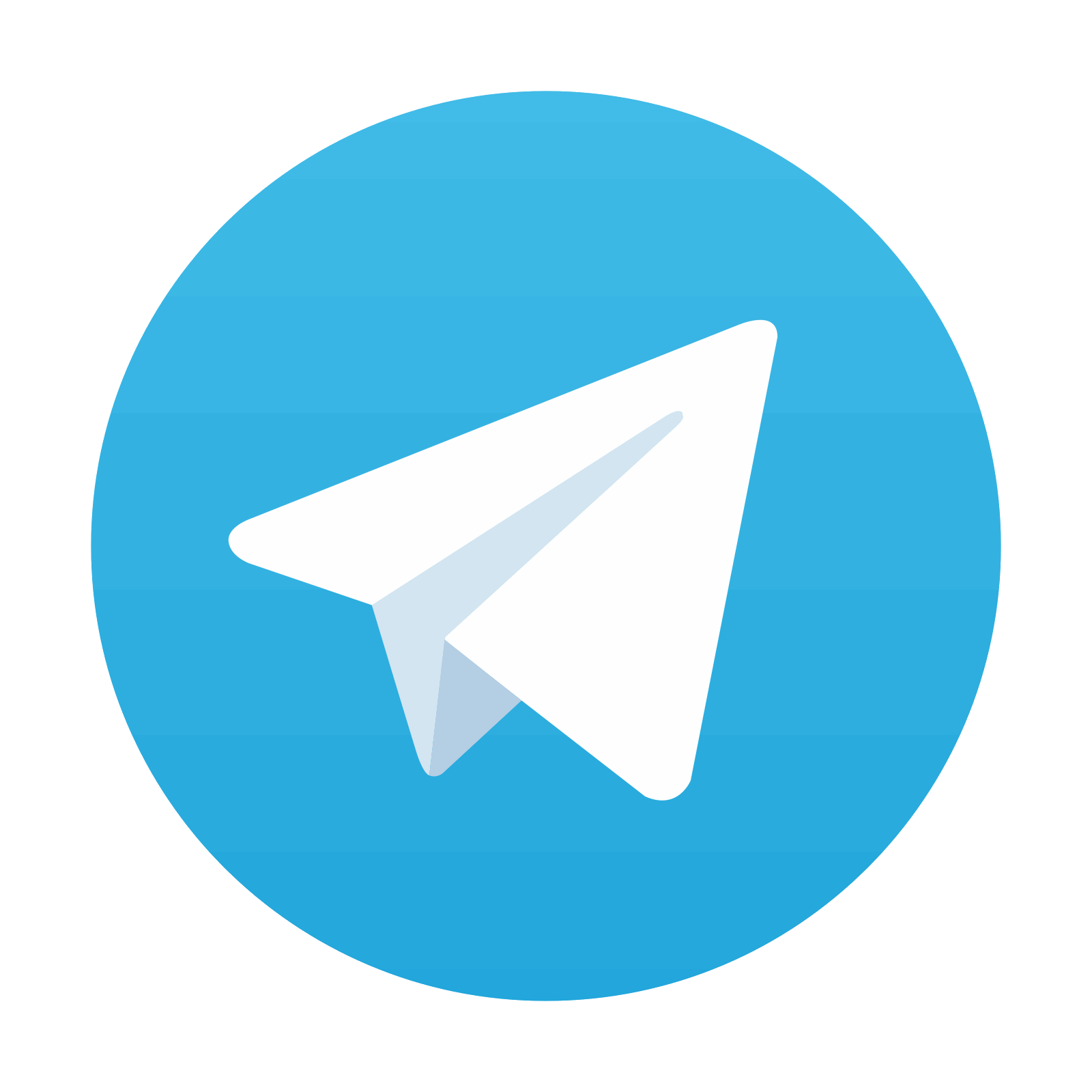
Stay updated, free articles. Join our Telegram channel

Full access? Get Clinical Tree
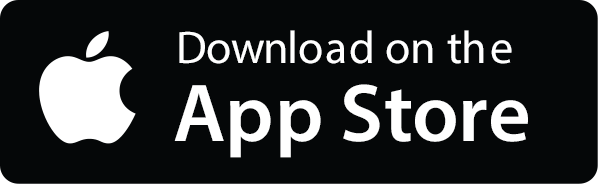
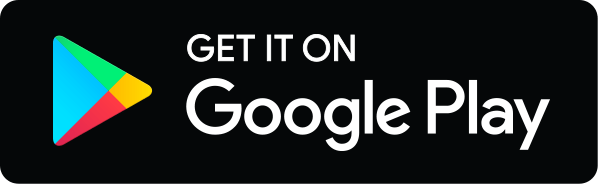
