Introduction to PET radiopharmaceuticals and radionuclides
PET radiopharmaceuticals are radioactive tracers used for diagnostic purposes in PET imaging. They consist of two main components, a positron-emitting radionuclide and a tracer/carrier molecule that delivers the radioactive label to the target ( ). The most commonly utilized PET radionuclides are short-lived ( ; ; ) as shown in Table 2.1 .
Radionuclide | Half-life |
---|---|
Fluorine-18 | 110 min |
Gallium-68 | 68 min |
Carbon-11 | 20 min |
Nitrogen-13 | 10 min |
Oxygen-15 | 2 min |
Rubidium-82 | 1.3 min |
Copper-64 | 12.7 h |
Selected clinically-relevant PET radiopharmaceuticals
18F- fluorodeoxyglucose (FDG)
Introduction and mechanism of uptake
FDG is a marker of glucose metabolism and the most widely used PET radiopharmaceutical worldwide ( ; ). FDG is incorporated into the cells through a family of glucose transporters (GLUT) and is subsequently phosphorylated by the hexokinase enzyme ( ; ). The resultant product, FDG-6-Phosphate, is trapped intracellularly without undergoing further metabolism.
Most tumors demonstrate increased glucose metabolism, a feature exploited by FDG PET for oncologic imaging, due to overexpression of membrane glucose transporters (especially GLUT-1 and GLUT-3) and increased hexokinase activity. Additionally, neoplasms often exhibit decreased levels of glucose-6-phosphatase ( ).
However, increased FDG uptake is not restricted to malignancies, since several benign pathologies/conditions demonstrate increased glucose metabolism, which could act as a confounder in tumor imaging ( ). Such processes include active inflammation, hypermetabolic brown fat, and muscular activity ( ). Therefore, the diagnosis of malignancy needs to be established in the proper clinical and imaging context. Additionally, the metabolic activity of tumors can vary, resulting in variable PET appearance.
Indications/uses
FDG PET is commonly used for diagnosis, staging, and restaging of tumors, treatment response evaluation, and radiation and surgical planning. Common oncologic applications include evaluation of a wide range of malignancies such as lymphoma, lung cancer, head and neck malignancies, gastrointestinal cancers, melanoma, and multiple myeloma among others. Some of the most common nononcologic indications include work-up of fever of unknown origin, vasculitis, sarcoidosis, vascular graft infection, dementia, seizures, and assessment of myocardial viability ( ; ).
Patient preparation
While specific recommendations vary by institution and clinical indications, some general guidelines are provided below ( ; ):
Dietary preparation
- a.
Fasting: complete fasting for a minimum of 4–6 h before the scan is usually recommended, to minimize glucose-related competitive inhibition of FDG uptake and maintain near baseline serum insulin level. Parenteral and tube feeding as well as dextrose-containing intravenous fluids should also be stopped 4–6 h prior to the study.
- b.
Hydration: proper hydration is recommended before the study for maintaining low radiopharmaceutical concentration in the serum and urine and limiting the radiation dose to the renal collecting system and bladder, which is the critical organ ( ).
- c.
Special diets:
- –
Diet for cardiac sarcoidosis evaluation: sarcoidosis-affected myocardium demonstrates upregulation of glucose metabolism. Suppression of physiologic FDG uptake by normal unaffected cardiac myocytes is key for cardiac sarcoidosis evaluation. This is typically achieved by avoidance of carbohydrates-containing food and consumption of fat- and protein-rich food for at least 24 h prior to imaging followed by overnight fasting. Suppression of myocardial glucose uptake could be boosted by intravenous administration of unfractionated heparin, which elevates plasma levels of free fatty acids with resultant increased cardiac utilization of free fatty acids instead of glucose ( ).
- –
Diet for myocardial viability study: to maximize FDG uptake in the myocardium, the patient receives a glucose load after a fasting period of approximately 6 h to increase pancreatic insulin production and release. This in turn reduces plasma fatty acid levels and favors the myocardial consumption of glucose over fatty acid. This is most commonly achieved with an oral glucose load of 25–50 g ( ).
- –
Activity restriction
Strenuous activities such as running should be avoided for a minimum of 24 h to minimize muscular uptake of the radiopharmaceutical. Chewing gum should be avoided to decrease uptake in the muscles of mastication. The patient should remain seated or recumbent before and after 18F-FDG administration to avoid muscular uptake.
Glucose levels and diabetes
Elevated blood glucose level results in insulin release, which subsequently upregulates GLUT4 translocation. This elicits FDG uptake in organs with high insulin receptor density including the skeletal muscles. The overall process causes altered radiopharmaceutical biodistribution and potentially decreases the sensitivity of the FDG PET. Additionally, hyperglycemia is associated with decreased cerebral uptake; therefore, even tighter blood glucose control is recommended for brain PET studies. Most guidelines recommend blood glucose levels to not exceed 200 mg/dL prior to radiopharmaceutical injection. While glycemic control is important, chronic hyperglycemia due to uncontrolled diabetes is far less problematic for FDG tumor imaging compared to acute insulin rise in response to eating. Therefore, in patients with poorly-controlled chronic hyperglycemia, a higher glucose level threshold could be tolerated. For diabetic patients, scheduling the exam early in the morning in the fasting condition with insulin withheld, or later in the day but at least 4 h after administration of short-acting insulin is encouraged. Oral hyperglycemic agents are typically allowed and not withheld before the study. However, metformin could cause diffuse intense bowel uptake that limits bowel evaluation. Some studies have suggested that replacing metformin with other oral antidiabetic medications for 3 days before the scan could facilitate bowel evaluation ( ).
Environmental precautions
Cold exposure variably stimulates brown adipose tissue via an adrenergic mechanisms. This sometimes results in intense radiopharmaceutical uptake in areas where brown fat is more represented, including the cervical, thoracic, and upper abdominal regions, as described below. To minimize brown fat uptake, patients are advised to stay in a warm environment for the 2 days preceding the scan and even more so for at least 30–60 min before injection of the radiopharmaceutical. Additionally, and especially for brain imaging, the patient should remain in a quiet and dimly lit room for FDG administration and during the subsequent uptake phase.
Radiopharmaceutical administration and postinjection wait period
The radiopharmaceutical is administered intravenously. An incubation period of approximately 60 min is usually recommended for radiopharmaceutical uptake and distribution throughout the body. Ideally, the patient should stay in a seated or recumbent position in a quiet dimly lit and warm room.
Normal biodistribution
Variable degrees of uptake could be seen in different organs and regions as shown and discussed below ( Fig. 2.1 ) ( ; ):
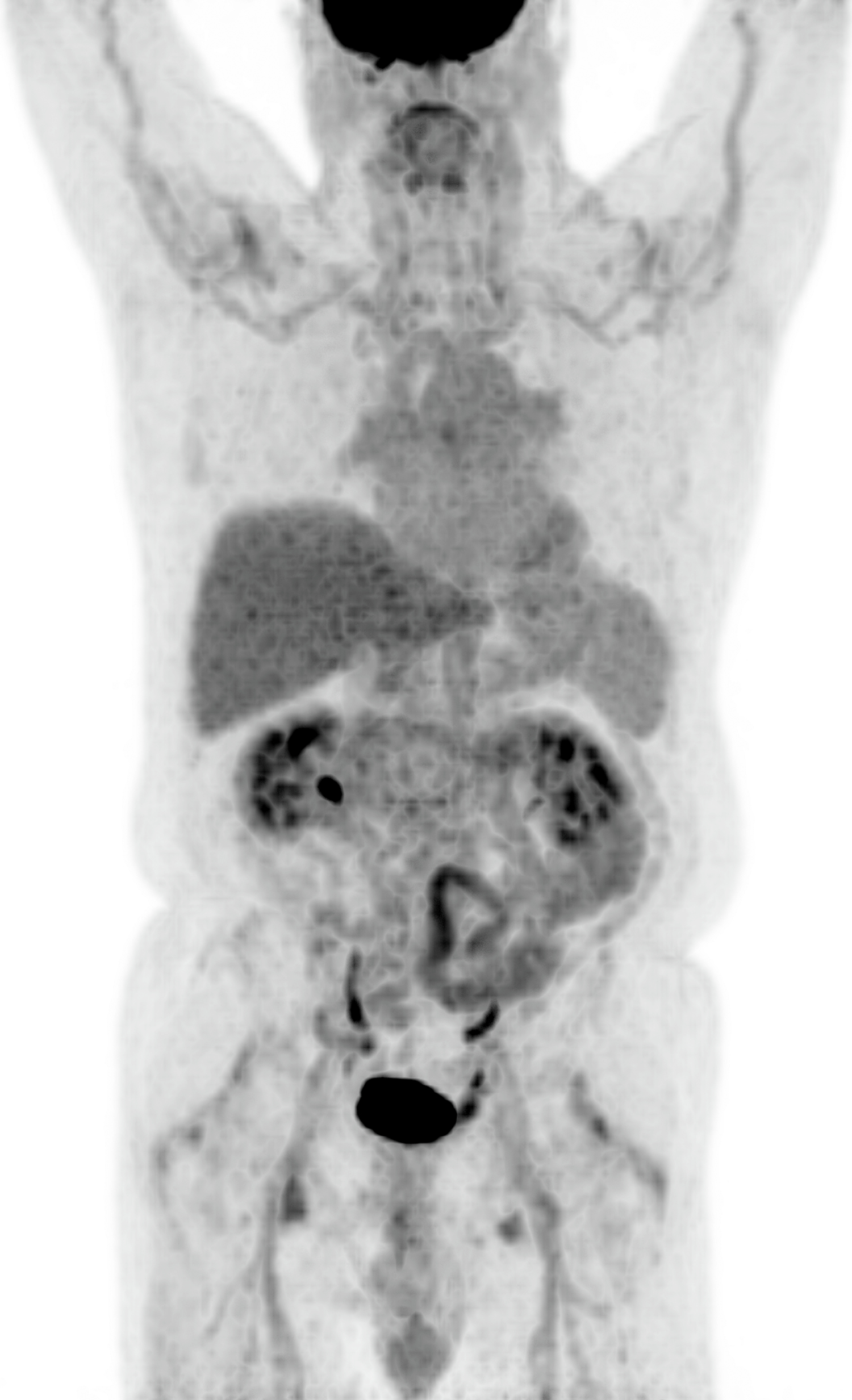
Head and Neck: intense physiologic uptake is usually seen in the cerebral cortex and basal ganglia. Variable degrees of uptake are seen in lymphatic tissues including the tonsils and Waldeyer’s ring, more prominent in young patients. Variable degrees of uptake can be seen in the ocular muscles, muscles of mastication, and the tongue. Variable but usually low-level uptake is seen in the salivary glands and vocal cords. Phonation could result in intense vocal cord uptake.
Thorax: There is usually low-level uptake to mediastinal structures with variable uptake to the thymus, glandular breast tissue and the spinal cord, particularly at the level of T12-L2. Marked uptake in the breasts is usually seen in lactating mothers. Myocardial uptake is dependent on fatty acid metabolism. However, in the cases of hyperinsulinemia, including the nonfasting state, the myocardium switches to glucose metabolism and intense uptake in the myocardium could be seen. Therefore, myocardial uptake is very variable and can range from completely suppressed to very intense, depending on the dietary preparation.
Abdomen/pelvis: splenic uptake is usually similar to or less than the liver. FDG is usually filtered by the glomeruli resulting in significant activity in the renal collecting system, ureter, and bladder. Additionally, there is typically moderately intense physiologic uptake in the renal parenchyma. The stomach may show low-level homogenous uptake. Diffuse nonfocal variable uptake to the bowel is common. High uptake is usually observed in the colon followed by the small bowel. Symmetric diffuse testicular uptake and faint uterine activity are commonly seen. Besides FDG uptake in the case of corpus luteum, ovarian uptake is not common.
Skeleton and soft tissues: bone marrow normally shows low-level uptake that is less intense than the liver in the absence of conditions that lead to bone marrow stimulation. However, the patient’s receiving granulocyte colony-stimulating factor (G-CSF) may have increased bone marrow and splenic activity. Rested/relaxed skeletal muscles typically do not demonstrate significant uptake. Increased skeletal muscle uptake could be seen after physical activity and/or in the setting of hyperinsulinemia, usually due to nonfasting state, as insulin increases muscle uptake. Multiple nonmalignant processes could result in osseous and/or soft tissue uptake including degenerative changes, fractures, postoperative changes, biopsy sites, etc.
Brown adipose tissue: inactive brown fat shows no significant FDG uptake. However, metabolically active brown fat is intensely avid. Brown fat is activated by cold temperatures. Children have high brown fat stores, which classically decline with age. The typical distribution of brown fat is symmetric and includes the neck, supraclavicular, axillary, mediastinal, paraspinal, and upper abdominal perinephric, para-aortic, and suprarenal regions ( Fig. 2.2 ). Brown fat could potentially obscure suspicious findings in these regions; therefore, careful evaluation of these areas on anatomic imaging is encouraged. These areas of uptake should correspond to fat density on CT and MRI. Keeping the patient warm is the easiest approach to minimize brown fat hypermetabolism. However, certain pharmacologic interventions could also be utilized, including the administration of diazepam ( ) and beta-blockers such as propranolol ( ).
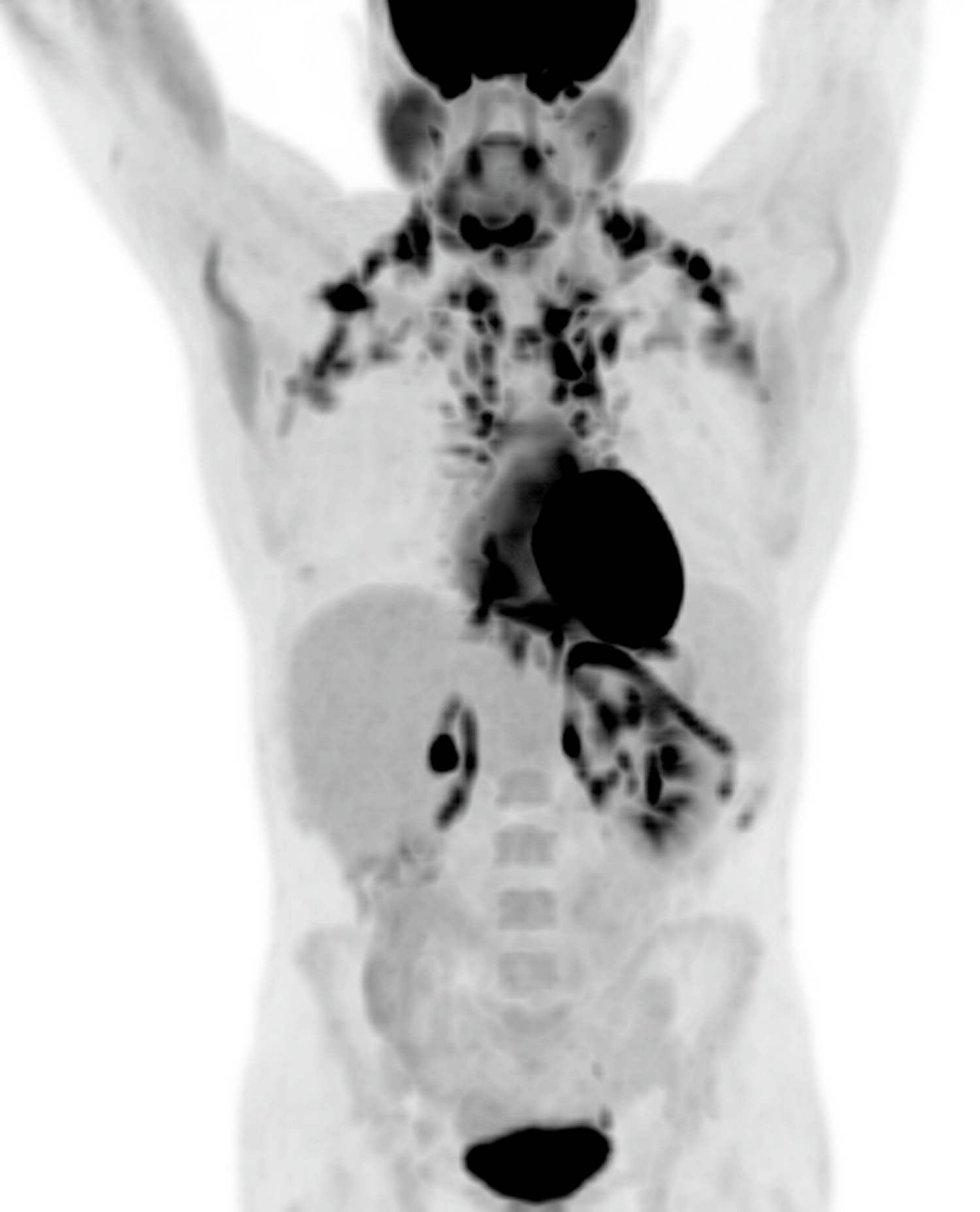
Pitfalls
As mentioned earlier, any process that results in glucose hypermetabolism could cause elevated FDG uptake ( ). Such nonmalignant processes include some benign tumors (such as some bowel adenomas, benign parotid tumors, renal oncocytoma), infectious processes, noninfectious inflammatory processes (such as colitis, vasculitis, and IgG4-related disease), granulomatous disease such as sarcoidosis ( Fig. 2.3 ), hyperplasia or dysplasia (such as Cushing’s disease, Paget’s disease, and bone marrow hyperplasia in the setting of anemia), postoperative and posttraumatic changes, and other conditions (such as Erdheim-Chester disease). Additionally, physiologic uptake may lead to false-positive interpretations such as brown fat hypermetabolism, lymphoid tissue uptake, thymic uptake especially in children, and endometrial uptake during menstruation and ovarian uptake in premenopausal women ( ).
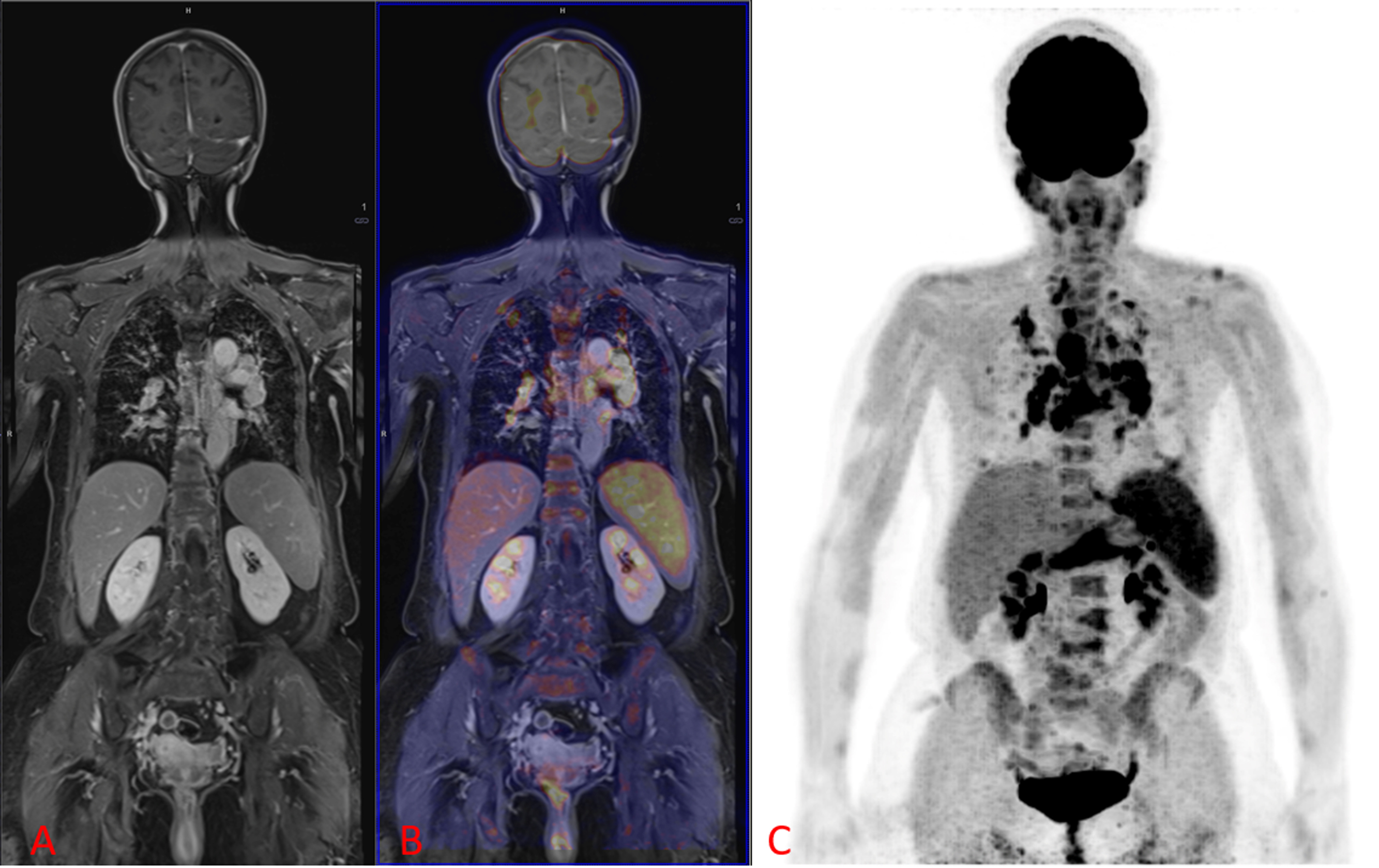
Many tumors are not particularly very FDG-avid, which can lead to false-negative reads ( ). These include low-grade tumors such as low-grade lymphomas, predominantly mucinous tumors, some hepatocellular carcinomas (HCCs), well-differentiated prostate adenocarcinoma and neuroendocrine tumors. Other causes of false-negative interpretation include small lesion size, tumor necrosis, ground-glass pulmonary nodules, hyperglycemia and/or hyperinsulinemia, and recent high-dose steroid therapy.
68Ga-DOTATATE, 64Cu-DOTATATE and 68Ga-DOTATOC
Introduction and mechanism of uptake
68Ga-1,4,7,10-tetraazacyclododecane-1,4,7,10-tetraacetic acid (DOTA)-conjugated peptides target somatostatin receptors (SSTR), which are expressed in several normal tissues as well as in neoplasms, such as neuroendocrine tumors (NETs) ( ). The different subtypes of 68Ga-DOTA-conjugated peptides, while demonstrating diverse affinity toward the various SSTR subtypes, all bind to SSTR2. The most commonly used radiopharmaceuticals are SSTR agonists ( ; ; ; ; ) such as:
- –
68Ga-DOTATOC ([68Ga-DOTA0 -Tyr3] octreotide): Binds predominantly to SSTR2, and SSTR5 (with lower affinity compared to DOTANOC).
- –
68Ga-DOTANOC ([68Ga-DOTA0-1NaI3] octreotide): Has a higher affinity for SSTR2, although it also shows a high affinity for SSTR3 and SSTR5 compared to 68Ga-DOTATATE.
- –
68Ga-DOTATATE Octreotate ([68Ga-DOTA0 -Tyr3] octreotate): Has a high affinity for SSTR 2, and higher physiological uptake in the pituitary and salivary gland compared to 68Ga-DOTANOC.
- –
68Ga-DOTA-lanreotide: Binds to SSTR2 and SSTR5.
64Cu-DOTA-TATE, an additional radiopharmaceutical, has favorable positron energy (maximum positron energy: 0.653 MeV). In addition, it has a considerably lower positron range than 68Ga (maximum positron energy, 1.899 MeV). 64Cu-DOTATATE has shown advantages over 68Ga-DOTATOC in the evaluation of NETs, as significantly more lesions may be detected by 64Cu-DOTATATE PET ( ).
Indications/uses
SSTR targeting radiopharmaceuticals are used for the detection of well- or moderately differentiated NETs that express SSTR(s), most of which predominantly express SSTR2. PET imaging could be performed for diagnosis, initial staging, restaging, or evaluation of treatment response. In addition, it is used as a prognostic tool to select candidates for treatment with somatostatin analogues or SSTR-targeting radionuclide therapy ( ; ).
Patient preparation
Although specific recommendations vary by institution and clinical indication, some general guidelines are as follows:
- 1
Withdrawal of somatostatin analog medications, when possible and not contraindicated, to avoid potential SSTR blockade 1 day prior to PET for short-acting SSTR analogs and at least 3–4 weeks prior to PET for long-acting analogs. These recommendations are currently not standardized worldwide ( ).
- 2
Dietary preparation:
- (a)
Fasting is not necessary prior to radiopharmaceutical injection ( ).
- (b)
Hydration: adequate hydration is recommended prior to imaging to maintain low serum and urine concentration of the radiopharmaceutical and to limit the radiation dose to the renal collecting system and bladder, which are the critical organs.
- (a)
- 3
Immediately prior to image acquisition, patients are asked to void to help eliminate urinary excreted 68Ga not bound to peptides, thus reducing background noise, and decreasing the genitourinary radiation dose ( ).
Radiopharmaceutical administration and image acquisition
The radiopharmaceutical is administered intravenously. After injection of the radiopharmaceutical, an incubation period of approximately 60 min is usually recommended for radiopharmaceutical uptake and distribution throughout the body.
Normal biodistribution
SSTRs are expressed in many neuroendocrine and nonneuroendocrine cells in the body resulting in 68Ga-DOTA-conjugated peptides accumulation in several normal organs ( Fig. 2.4 ). Physiological 68Ga-DOTATATE biodistribution is described below ( ; ).
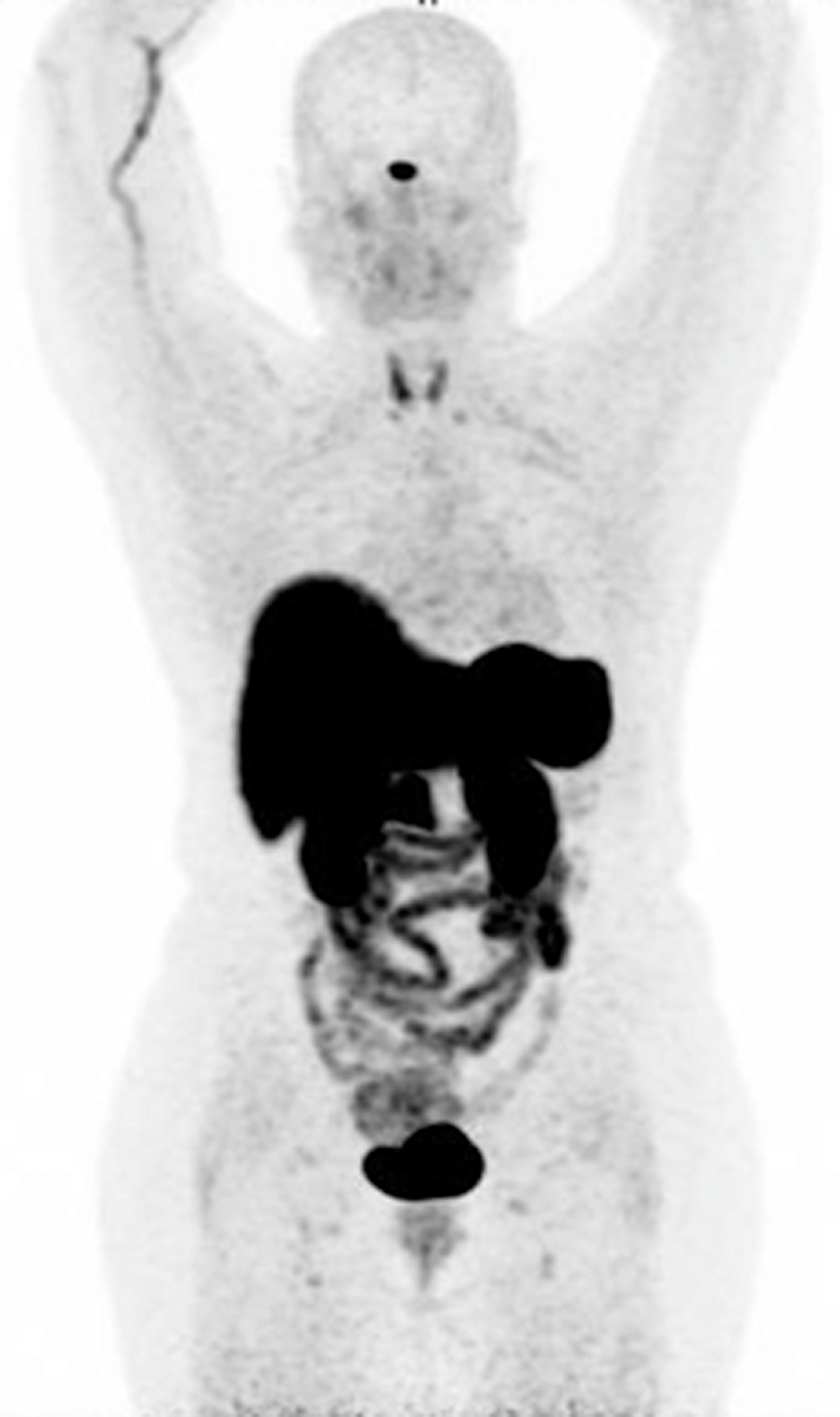
Head/Neck: intense physiologic uptake is observed in the pituitary gland, with mild to moderate uptake in the salivary glands and thyroid.
Thorax: breast glandular tissue may show diffuse low-grade physiological uptake.
Abdomen/pelvis: marked physiologic uptake is shown in the spleen, kidneys, adrenals and liver. The highest physiologic uptake is usually seen in the spleen, which is the critical organ ( ). Variable moderate uptake is observed in the stomach wall and intestine. The pancreas shows variable uptake of 68Ga-DOTA-conjugated peptides. Although all five SSTR subtypes are present in the pancreas, SSTR2 receptors are preferentially localized in the islets. The higher concentration of islets cells in the head and uncinate process of the pancreas results in intense physiological uptake in this region. The prostate gland may demonstrate mild diffuse uptake.
Skeleton and soft tissues: musculoskeletal uptake is usually low/negligible.
PET interpretation
Different tumor histotypes have variable degrees of DOTA radiopharmaceuticals uptake ( ).
- 1.
Tumors typically with high SSRT expression level: Functioning and nonfunctioning gastro-entero-pancreatic NET (e.g., gastrinoma, insulinoma glucagonoma, VIPoma), NET of the lungs, tumors of the sympathetic-adrenal system (e.g., paraganglioma) and meningioma.
- 2.
Tumors typically with low or variable expression of SSTRs: Breast carcinoma, melanoma, lymphoma, prostate carcinoma, nonsmall cell lung cancer, head and neck tumors, sarcoma, renal cell carcinoma, differentiated thyroid carcinoma and astrocytoma.
Pitfalls
68Ga-DOTA radiopharmaceuticals demonstrate high physiological uptake to the uncinate process of the pancreas, spleen, pituitary gland, kidneys and adrenal glands, which limits evaluation for underlying pathologies in these regions. Some nonneoplastic conditions can also induce increased uptake, particularly inflammation (e.g., prostatitis, postradiation changes, reactive lymph nodes), bone remodeling (e.g., osteoarthritis and recent fractures), fibrous dysplasia, epiphyseal growth plates and splenosis. In addition, some benign neoplasms could demonstrate uptake (e.g., meningiomas, osteomas and hemangiomas).
Endogenous somatostatin production by the tumor(s) and exogenous somatostatin analogue therapy by short- or long-acting octreotide may interfere with the degree of DOTA peptide uptake by the tumors, which could result in a false-negative read. Tumor differentiation level and inter and intratumoral heterogeneous SSTR expression, and small lesion size (<5 mm) may also lead to false-negative results ( ).
18F-fluciclovine
Introduction and mechanism of uptake
Anti-1-amino-3-fluorocyclobutane-1-carboxylic acid labeled with [18F]Fluorine (also known as 18F-Fluciclovine or 18F-FACBC) is an FDA approved prostate cancer imaging agent. 18F-fluciclovine is an amino acid (leucine) analog, which shows high uptake in cancer cells due to increased protein turnover ( ). Currently, 18F-fluciclovine is mainly used for the evaluation of patients with biochemical recurrence of prostate cancer. The sensitivity for detecting recurrent disease increases with higher Gleason scores and higher prostate specific antigen (PSA) levels ( ; ). Sensitivity reaches up to 85% at PSA levels above 6 ng/mL ( ). 18F-fluciclovine PET is highly sensitive but not very specific for detecting local recurrence within the prostate gland as intraprostatic uptake could be benign or due to residual cancer. In addition, 18F-fluciclovine PET alone underperforms in detecting the osseous metastatic disease when compared to bone scintigraphy or combined 18F-fluciclovine PET/MRI ( ).
Indications/uses
18F-Fluciclovine is currently used for suspected prostate cancer recurrence.
Patient preparation
Fasting for at least 4 h is usually recommended. Strenuous exercise should be avoided for at least a day before the study as it could lead to an increase in protein synthesis and muscle uptake ( ).
Following the study, the patient should remain hydrated and urinate frequently in order to reduce the radiation exposure to the bladder.
Radiopharmaceutical administration and image acquisition
18F-Fluciclovine is administered intravenously and is rapidly taken up by prostate cancer cells. The tumor-to-background ratio is the highest between 1 and 15 min postadministration ( ). Therefore, early image acquisition is encouraged, ideally within 3–5 min after injection of 18F-fluciclovine. PET scanning proceeds from the proximal thigh toward the base of the skull to minimize urinary accumulation of the radiopharmaceutical in the bladder, which could obscure suspicious findings ( ).
Normal biodistribution
Variable degrees of uptake can be seen in different organs and regions as shown ( Fig. 2.5 ) and discussed below ( ).
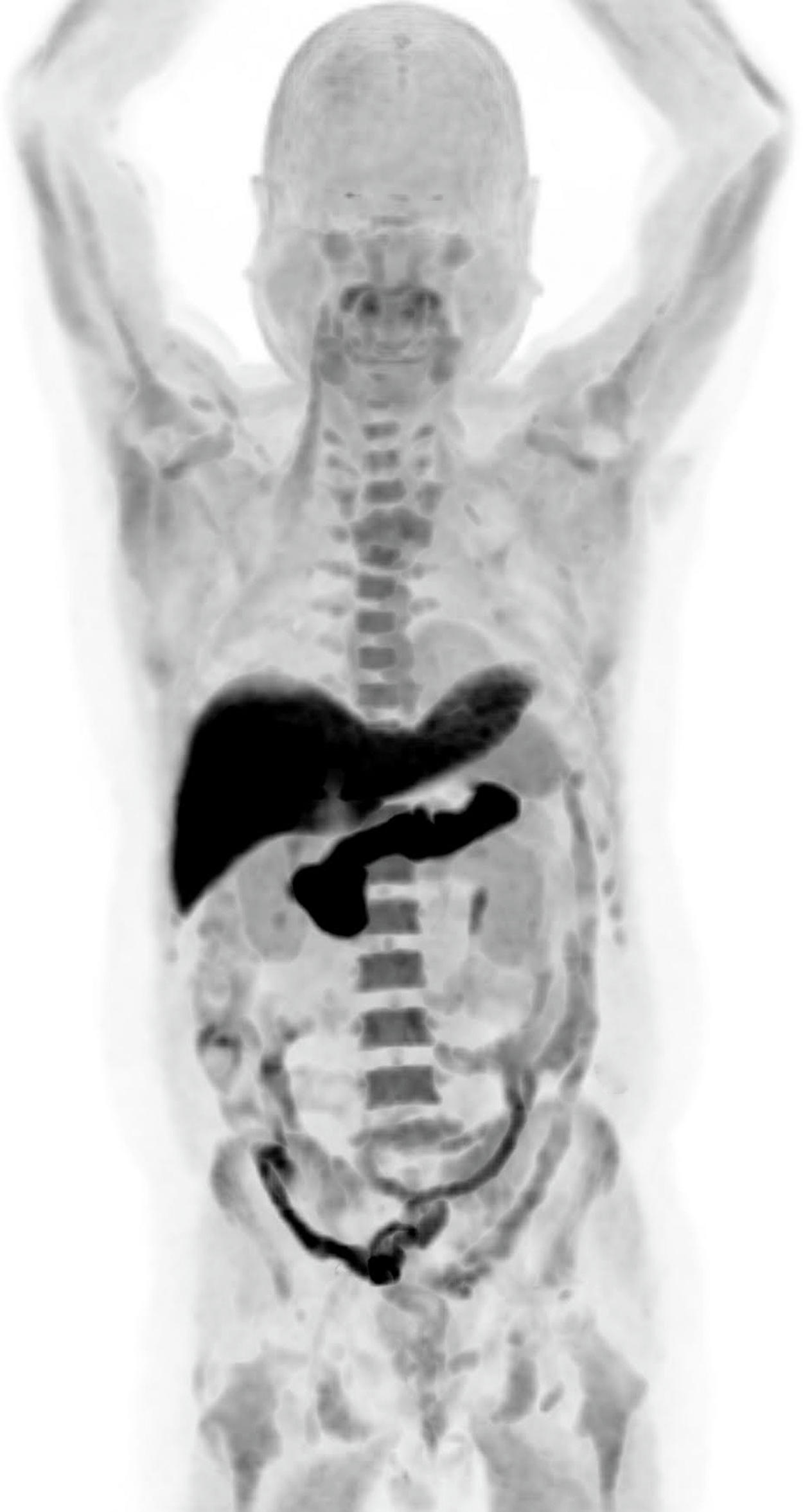
Head/neck: low to moderate uptake is typically seen in the salivary glands, lymphoid tissue, thyroid, and pituitary gland.
Thorax: breast parenchyma demonstrates mild diffuse uptake. Myocardial uptake is variable and tends to become more prominent in later phases of image acquisition. Mild diffuse physiologic esophageal uptake can also be seen in some patients.
Abdomen/pelvis: The liver and pancreas demonstrate the most intense physiologic uptake with the pancreatic uptake slightly higher than the liver ( Fig. 2.5 ). Mild to moderate uptake is usually seen in the spleen and renal parenchyma, adrenal glands, stomach and bowel. The normal prostate gland shows mild diffuse uptake. Linear or focal uptake along the urethra could also be seen due to physiologic excretion of the radiopharmaceutical in the urine.
Skeleton and soft tissues: Heterogeneous bone marrow uptake is commonly seen. Skeletal muscle uptake is variable and tends to increase over time after radiopharmaceutical administration and with exercise.
PET interpretation
Prostatectomy bed: Focal uptake level in the prostatectomy bed (SUVmax) at or greater than that of bone marrow, preferably at the L3 vertebrae (SUVmean), is considered suspicious for malignancy. Uptake between blood pool and bone marrow does not meet diagnostic criteria but requires close follow-up or correlation with dedicated prostate MRI. Symmetric uptake to seminal vesicles similar to blood pool is likely physiologic while asymmetric intense uptake may represent malignancy ( ).
Prostate gland: diffuse, focal, or multifocal uptake greater than that of the bone marrow is likely malignant; meanwhile, uptake between blood pool and bone marrow does not meet diagnostic criteria for malignancy and requires close follow-up or prostate MRI evaluation ( ).
Lymph nodes: Uptake equal to or greater than bone marrow in a lymph node in a distribution typical for recurrence is considered suspicious for malignancy while uptake between blood pool and bone marrow requires close follow-up. Lymph nodes in an atypical location for recurrence such as inguinal, axillary, and thoracic hilar regions are most often inflammatory unless seen in patients with extensive metastatic disease ( ).
Bones: Intense focal uptake is considered suspicious for malignancy. Lytic osseous lesions tend to show greater uptake than sclerotic lesions on 18F-fluciclovine. Sclerotic lesions could be false-negative; additional imaging evaluation should be considered ( ).
Liver: focal uptake greater than that of normal liver parenchyma or less than liver but greater than bone marrow is suspicious for malignancy ( ).
Pitfalls
Benign prostatic hyperplasia and postradiation inflammation and fibrosis are the most common reasons for false-positive uptake in the prostate gland ( ). Similarly, false-positive uptake in nodes could be due to reactive changes or due to other malignancies, especially if found in atypical locations for prostate carcinoma recurrence/metastatic disease. Other cancers that could demonstrate 18F-flucinvolive uptake include lymphoma, breast, lung, and brain cancers. Any degree of 18F-fluciclovine uptake in a renal lesion should prompt further evaluation as it might represent malignancy. Nonmalignant entities could also demonstrate uptakes such as meningiomas and adrenal adenomas.
68Ga and 18F labeled Prostate-Specific Membrane Antigen (PSMA)-targeted radiopharmaceuticals
Introduction and mechanism of uptake
Prostate-Specific Membrane Antigen (PSMA) is a cell-surface transmembrane protein that is overexpressed in the majority of prostate adenocarcinomas. The expression is further increased in poorly differentiated and metastatic prostate cancer ( ). Several radiolabeled probes have been developed, which bind with high affinity to PSMA’s active extracellular domain, including 68Ga-PSMA-11, 18F-PSMA-1007, and 18F-DCFPyL. The use of these radiopharmaceuticals is especially helpful in patients with relatively low PSA values (between 0.2 and 10 ng/mL). 68Ga-PSMA-targeted PET/CT has been shown to be superior for the detection of metastatic disease as compared to CT, MRI, and bone scintigraphy ( ; ). Pelvic MRI is still the modality of choice for local tumor delineation. Consequently, PSMA-targeted PET/MRI could provide a great tool for the precise evaluation of local and metastatic disease.
18F-DCFPyL PET/CT has an overall detection rate of approximately 85% in patients with biochemically recurrent prostate cancer. The rate increases with higher prostate specific antigen (PSA) levels (ng/mL): 50% (PSA <0.5), 69% (0.5 ≤ PSA <1), 100% (1 ≤ PSA <2), 91% (2 ≤ PSA <5), and 96% (PSA ≥5) ( ). When compared to 18F-fluciclovine, PSMA-targeted PET demonstrates higher cancer detection rates in patients with early biochemical recurrence after radical prostatectomy (PSA ≤2.0 ng/mL) ( ). 18F-radiolabeled PSMA targeting probes enable large-scale radiosynthesis as compared to the limited quantity of 68Ga ( ).
Indications/uses
Current most common uses include localization of prostate cancer in the setting of biochemical recurrence, primary staging in patients with high-risk disease (such as Gleason score >7, PSA >20 ng/mL), detection of nodal and/or osseous metastatic disease, and monitoring of treatment response ( ).
Patient preparation
Patients should be well-hydrated before the study and during the uptake period ( ). Voiding is encouraged before the scan to decrease high activity in the urinary system. Fasting is not required as a recent study utilizing 18F-DCFPyL showed that fasting does not significantly affect uptake in suspected malignant lesions ( ). Nonfasting results in significantly lower 18F-DCFPyL uptake in tissues with high physiologic uptake but the effect on diagnostic performance were thought to be negligible ( ).
Radiopharmaceutical administration and image acquisition
These radiopharmaceuticals are administered intravenously. An uptake period of 50–120 min is encouraged. Increased lesion detection has been shown with 3–4 h of delayed imaging ( ). However, delayed imaging is not always practical, especially in the case of 68Ga-PSMA-11, which has a relatively short half-life (68 min). Alternatively, initial PET acquisition could be performed at 60 min and additional delayed images could be acquired if there are any indeterminate findings on the initial scan.
Similar to 18F-Fluciclovine, PSMA-targeted PET acquisition is preferably done in the caudocranial direction from the midthighs toward the base of the skull base, in order to capture the pelvic organs while there is a low postvoid activity in the bladder.
Normal biodistribution
Studies have shown that normal biodistribution patterns of 68Ga-PSMA-11 and 18F-DCFPyL are similar ( ; ; ) ( Fig. 2.6 ).
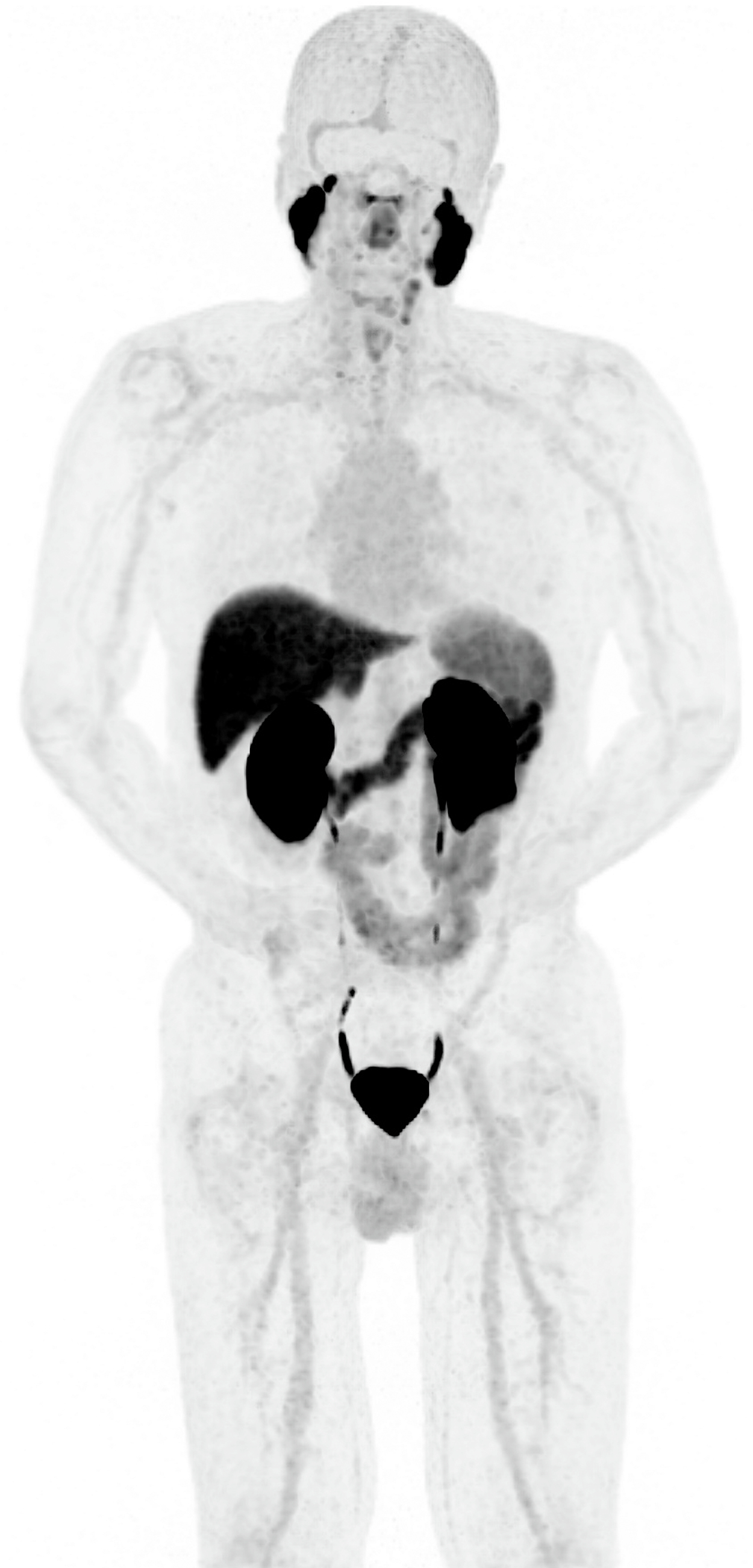
Head and neck: Salivary glands usually demonstrate intense uptake. Moderate uptake is also found in the lacrimal glands.
Thorax: low level mediastinal and blood pool uptake is typically seen.
Abdomen/pelvis: The kidneys demonstrate the most intense uptake in the body, followed by the salivary glands. Moderately intense uptake is seen in the duodenum and proximal jejunum. Moderate uptake is usually seen in the liver and spleen. Variable low-level uptake can be seen in the pancreas, adrenal glands, prostate gland, and normal lymph nodes. These agents are excreted predominantly by the urinary system and, therefore, bladder activity could be intense.
Skeleton: Nonfocal low-level bone marrow and muscular uptake can be seen.
PET interpretation
Intraprostatic primary or recurrent lesions and extraprostatic metastatic lesions typically demonstrate a high tumor to background uptake ratio ( ).
Pitfalls
PSMA expression can also be found in other types of cancers such as colon, esophageal, thyroid, lung, hepatocellular, renal, and brain cancers. Uptake could also be seen in multiple benign entities such as infectious and inflammatory processes, Paget’s disease, and benign neoplasms such as schwannomas, parathyroid adenomas, and many others ( ).
An important pitfall of PSMA-targeted imaging is radiopharmaceutical uptake in the sympathetic ganglia. In particular, uptake to the celiac ganglia is higher than cervical or sacral ganglia, which could lead to misinterpretation as retroperitoneal nodal metastasis ( ). However, the intensity of uptake, exact localization on anatomic imaging, and configuration should be assessed carefully to avoid such misinterpretations ( Fig. 2.7 ). In this regard, the superior anatomic layout of PET/MRI, as compared to that of PET/CT, might be advantageous.
