Fig. 1
Left panel Grand-averaged source localization of beta-ERD (15–30 Hz) with the main peak estimated to be in the left precentral gyrus (i.e., contralateral to the finger movement. Uncorrected baseline (a) and baseline corrected (b) beta (15–30 Hz) envelopes time-locked to the movement onset for the location with maximal beta-ERD as shown in the spatial map. Timecourse estimates obtained before (Pre) and 1, 3, and 5 h after administration of tiagabine or placebo are superimposed. Averaged values are plotted for the baseline period (c), active period (b) and the active–baseline difference (e). There was beta power increase in the baseline and a larger ERD (active–baseline) with tiagabine (Muthukumaraswamy et al. 2012, used with permission)
Even though the effects of benzodiazepines are particularly evident in increased beta power over sensorimotor cortices (Jensen et al. 2005; Hall et al. 2010, 2011), they modulate oscillatory changes in other frequency bands as well. Hall et al. (2010) reported distributed power increases in alpha (7–14 Hz) and gamma (30–80 Hz) bands, as well as theta power decrease (4–7 Hz) in frontal regions. Ahveninen et al. (2007) administered a benzodiazepine drug to healthy controls in a placebo-controlled design and recorded MEG during resting with eyes open or closed. Focusing on the alpha frequency which dominates the resting spectrum, they applied a distributed minimum norm inverse estimate (Lin et al. 2004). The estimates were anatomically constrained with the realistic shape of the cortical mantle obtained from MRI scans on the same subjects (Dale et al. 2000). Benzodiazepine administration reduced power in the alpha band which was estimated to originate in the medial occipital cortex. Indeed, it has been proposed that alpha oscillations are subserved by GABAergic currents and that they play an important role in modulating attentional processing (Mazaheri and Jensen 2010). Taken together, MEG studies manipulating GABA provide important insight into the neurochemistry underlying different functional states (e.g., motor activation and rest) in healthy individuals, and can illuminate how the GABA function is altered in disease when these paradigms are applied to patient cohorts.
3.2 Acetylcholine
Acetylcholine is a major neurotransmitter in both the central and peripheral nervous systems (Picciotto et al. 2012) with regulatory effects on vigilance, attention, learning, and memory functions (Everitt and Robbins 1997; Sarter et al. 2005; Hasselmo and Sarter 2011). Its contributions to cognition have recently begun to be explored with the MEG. Bauer and colleagues (2012) examined the effects of cholinergic modulation on oscillatory brain activity during a spatial visual attention task. They administered a cholinergic agonist (physostigmine) to healthy volunteers in a placebo-controlled design. The MEG signals were analyzed with a beamformer approach within the SPM environment (Van Veen et al. 1997). Oscillations in lower (alpha and beta) frequency bands were affected by physostigmine in the visual cortex only. In contrast, gamma-band power was selectively enhanced by physostigmine in the prefrontal cortex (Fig. 2). The results suggest that the cholinergic modulation may be expressed in a regionally- and functionally-specific manner across different frequency bands with particular relevance to top-down attentional control. Given the importance of acetylcholine for cognition (Klinkenberg et al. 2011), it is essential to expand and continue this line of research in order to further delineate its functional, anatomical, and neurotransmission specificity. This may be particularly relevant to the development of novel treatment options for dementia such as Alzheimer’s disease whose pathology is linked to cholinergic transmission but which has been rather minimally responsive to the available treatment including many of the currently available cholinergic neuromodulators (Sivaprakasam 2006; Leon et al. 2013). Degeneration of the cholinergic system has been shown to characterize Parkinson’s-related dementia as well (Bohnen and Albin 2011). Drugs enhancing the cholinergic function have been shown to ameliorate some of the cognitive and behavioral impairments in Parkinson’s patients (Rolinski et al. 2012). Given the increasing prevalence of neurodegenerative diseases and the severity of the accompanying deterioration of cognitive abilities (WHO 2006), it is essential to intensify search for successful biomarkers and treatments (Berg 2008; Caselli et al. 2006).
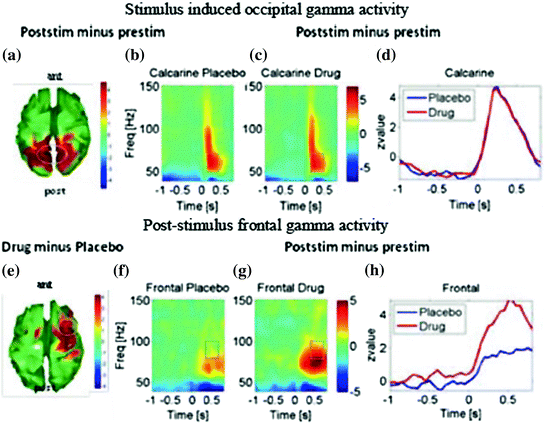
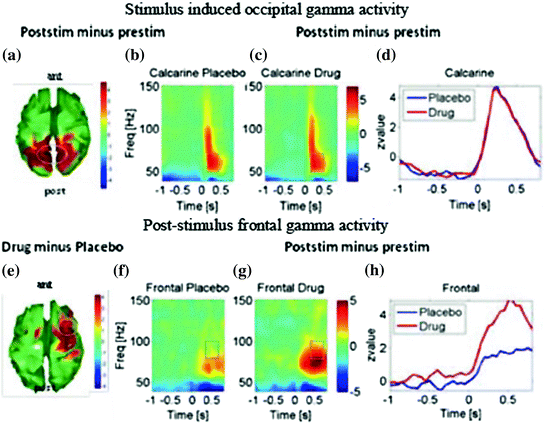
Fig. 2
a–d: Gamma activity induced by the onset of visual gratings and averaged across both hemispheres. Spatial attention was manipulated by cues indicating which hemifield to attend. a Stimulus-induced gamma increases for both drug and spatial attention conditions during the window marked in the time-frequency profiles are shown for the visual (peri-calcarine) cortex in b under placebo and in c under physostigmine. d Shows timecourses of induced 50–70 Hz gamma for the active drug and placebo conditions. e–h Induced gamma response for the frontal areas that show an enhancement by the cholinergic antagonist. e Topography for the statistical comparison between drug and placebo showing an increased gamma response over predominantly right frontal cortex. f Time-frequency profile of the response in area as marked in e under placebo and in g under physostigmine. b and c clearly show enhanced gamma-band response under physostigmine, which is confirmed with timecourses of induced 50–70 Hz gamma shown in (h). Values plotted are z-values for post- versus pre-stimulus power. Topography maps are thresholded at p < 0.01. The figure is used with permission (Bauer et al. 2012)
3.3 Dopamine
Dopamine is associated with memory and cognition functions (Goldman-Rakic 1998; Seamans and Yang 2004) and it plays a critical role in the neural circuitry of reward and addiction (Koob and Volkow 2010). The notion that DA imbalance underlies psychotic symptoms is the basis of the “dopamine hypothesis of schizophrenia” (Curran et al. 2004; Brunelin et al. 2013) lending additional importance to the neuroimaging investigations of DA function.
Modulatory effects of DA on memory have been examined with levodopa administration in a recent pharmacological MEG study (Moran et al. 2011). Levodopa is the catecholamine precursor resulting in increased dopamine availability (Olanow 2008). Moran et al. (2011) recorded MEG signals during a working memory task as healthy volunteers participated in a placebo-controlled acute levodopa (100 mg) challenge. They applied a dynamic causal modeling (DCM) approach in the context of the macrocolumnar architecture framework (Kiebel et al. 2009; Moran et al. 2009). The observed increased theta band activity under levodopa was estimated to the superior frontal gyrus and was related to behavioral performance within the DCM model of multidimensional synaptic signaling. In a study relying on time-domain analysis, Eckart and Bunzeck (2012) acquired MEG signals and administered levodopa (150 mg) or placebo to different groups of healthy volunteers as they were shown images that differed in the degree of novelty/familiarity. Sources of the ERF averages were estimated using the Linearly Constrained Minimum Variance (LCMV) beamformer approach (Van Veen et al. 1997) within the SPM8 environment. Increased levels of DA resulted in short latency (<100 ms) novelty differences that were estimated to originate in the medial temporal lobe. The results underscore prefrontal and temporal contributions to memory as a function of DA levels. Dopaminergic transmission is impaired in Parkinson’s disease, additionally giving high relevance to this type of study. MEG techniques can continue to provide insight into the basic mechanisms of the impairment as well as guidance for drug development when employed in healthy cohorts.
3.4 Parkinson’s Disease
PD is a degenerative disease characterized by motor deficits mainly resulting from the loss of DAergic neurons in substantia nigra (Bergman and Deuschl 2002). In addition, there is a progressive deterioration of non-motor abilities such as cognition which seems to be caused by other neurochemical (e.g., cholinergic) deficiencies (Coelho and Ferreira 2012; Bohnen and Albin 2011). The currently available treatment aims to restore DA levels and it commonly includes dopamine agonists and precursors (e.g., levodopa) in conjunction with agents targeting other neurotransmitter systems to mitigate cognitive dysfunction, psychotic symptoms, and treatment side-effects (Muller 2012).
MEG has been used in a series of “resting state” studies investigating oscillatory activity in PD patients across the span of the disease and as a function of dopaminomimetic and cholinomimetic therapy. Bosboom, Stoffers, and colleagues (Bosboom et al. 2006, 2009a, b; Stoffers et al. 2007, 2008a, b) recorded MEG signals during “eyes closed” resting state from groups of PD patients in their early or late disease stages as well as from healthy controls. The data were analyzed in sensor space with wideband spectral signal decomposition. Evidence from their group, as well as other groups consistently showed diffuse slowing of resting oscillatory activity in Parkinson’s patients with and without dementia symptoms (Bosboom et al. 2006; Stoffers et al. 2007; Kotini et al. 2005; Vardy et al. 2011). A longitudinal study revealed that this slowing worsens over time and is related to cognitive decline, but in a manner that is independent of aging effects (Olde Dubbelink et al. 2013). Furthermore, even untreated de novo PD patients showed significant slowing of the resting oscillatory activity that was expressed as a global power increase in the low frequency (<10 Hz) range and a loss of gamma power. These effects were not related to disease stage, duration, or other clinical indices and were only slightly affected by acute administration of dopaminomimetic medication (Stoffers et al. 2007). In contrast, cholinomimetic medication resulted in a shift towards faster frequencies, partially restoring the oscillatory deficit observed in PD patients (Bosboom et al. 2009a). Stoffers et al. (2007) interpreted these observations as evidence against a major role of the DA system in subserving the resting state brain oscillations in PD. Instead, they argue that other neurotransmitter systems including the cholinergic, noradrenergic, and serotonergic systems are involved in oscillatory alterations observed in PD (Bosboom et al. 2003; Brooks 2007). In another study, Stoffers et al. (2008a) examined functional connectivity in patients with PD and healthy controls by calculating temporal correlation between MEG epochs recorded during eyes-closed rest across pairs of sensors topographically grouped into regions of interest (Stam et al. 2002). Compared to healthy controls, PD patients exhibited increased levels of connectivity, which was related to motor symptoms (Stoffers et al. 2008a). Acute administration of dopaminomimetic medications increased the functional connectivity even further, which correlated with improved motor symptoms (Stoffers et al. 2008b).
A study by Pollok et al. (2009) investigated the effects of levodopa on functional connectivity during the parkinsonian resting tremor. They recorded MEG and EMG signals simultaneously from PD patients in their “off-medication” state (i.e., after overnight medication withdrawal) and immediately after an application of a fast-acting levodopa during rest. They examined cerebro-muscular and cerebro-cerebral coherence and applied the Dynamic Imaging of Coherent Sources (DICS) beamforming method (Gross et al. 2001) to estimate the MEG signal sources. The medication reduced the coupling strength within a thalamo-premotor/motor network at 8–12 Hz range, and was accompanied by a decrease in tremor and cerebro-muscular coherence. These results are taken as evidence of the drug-induced restoration of a normal functional interaction between the cortical and motor cortical regions.
In the clinical context of deep brain stimulation treatment for PD, Litvak and colleagues investigated the role of the basal ganglia and their functional connectivity with cortical areas in a series of multimodal imaging studies. They acquired MEG signals simultaneously with intracranial EEG (iEEG) recorded with depth electrodes implanted in the subthalamic nucleus (STN) (Litvak et al. 2011, 2012; Oswal et al. 2013). One study examined oscillatory synchronization between the signal in the basal ganglia and in cortical networks during resting with eyes open (Litvak et al. 2011). The coherence was estimated with Dynamic Imaging of Coherent Sources (DICS) beamforming method (Gross et al. 2001). A frontal network co-oscillated with the STN in the beta frequency range, whereas the network estimated to be in the temporoparietal area and the brainstem co-oscillated with the STN in alpha band. Acute effects of dopaminomimetic medications were examined by comparing the recording obtained after overnight medication withdrawal (OFF state) and after the usual dosage (ON state). The medication effects were expressed as an increase in beta coherence between the prefrontal cortex and STN. In another study, Litvak and colleagues used the same clinical setup and obtained simultaneous MEG and iEEG recordings during a finger movement task (Litvak et al. 2012). They examined movement-related oscillations estimated to originate in the motor cortex and those recorded from STN and their coherence in PD patients. Power and coherence in the gamma frequency range increased during movement and the increase was more pronounced during the ON state. Furthermore, the medication-induced increase in gamma co-oscillations at 60–90 Hz around the movement correlated with the improvement in motor symptoms, indicating their facilitatory modulation of motor activity. A companion study based on the same cohort and using the same paradigm reported effects in the alpha band that were complementary to the gamma power and coherence (Fig. 3) (Oswal et al. 2013). The coherence between the MEG-recorded alpha oscillations estimated to the right temporal cortex and the alpha in the STN was reduced after movement, particularly in the ON—medication state. Alpha suppression that preceded movement was unaffected by the medication state.
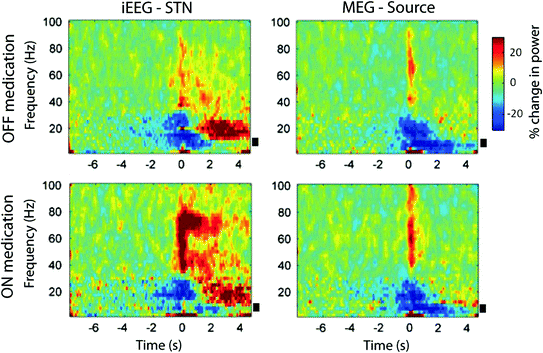
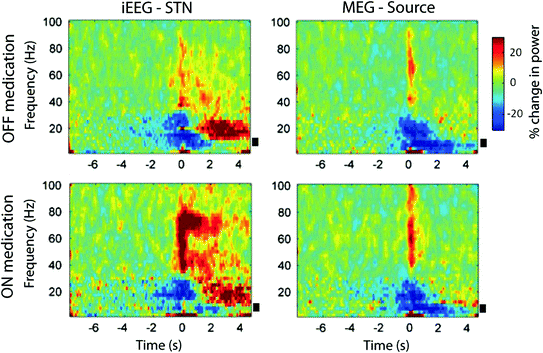
Fig. 3
Time-frequency images of power averaged across subjects for the STN (left column) and the right superior temporal MEG source (right column) during OFF medication (top row) and ON medication state (bottom row), recorded contralateral to movement. Power changes are expressed as percentage change calculated with respect to the baseline period from −8 to −5 s prior to movement. There is a beta desynchronization with onset prior to movement and gamma power increase upon movement. Gamma power increase is more marked ON medication. For the STN contacts, there was a significant reduction in alpha power from about 2 s before movement in both drug conditions. The black bars indicate alpha band frequencies between 7 and 13 Hz. Used with permission (Oswal et al. 2013)
Overall, this type of research can provide essential insight into the neurophysiology of neural disorders and can track the effects of different pharmacological treatments in a spatially- and temporally sensitive way. The rare opportunity to obtain combined MEG and iEEG data is particularly valuable for developing neurophysiologically realistic models of the basic mechanisms underlying motor and cognitive impairments and their sensitivity to pharmacological intervention. In this particular case, simultaneous recordings from the STN and the MEG estimates of cortical activity are especially advantageous for understanding the cortico-subcortical network and its sensitivity to pharmacological modulation in PD patients. In general, studies of patient populations are important for delineating biomarkers of the general and idiosyncratic features of the disease, for predicting treatment efficacy, and for guiding treatment development.
3.5 Attention Deficit Hyperactivity Disorder (ADHD)
ADHD is one of the most common neurobehavioral developmental disorders affecting ~5–7 % of children and persisting into adulthood (Willcutt 2012). It is characterized by hyperactivity which is particularly prevalent in children, whereas inattention and executive impairments are observed across the life span (Seidman 2006). Evidence from studies using MRI and EEG methods indicates structural and functional aberrations in individuals with ADHD (Cubillo et al. 2012; Cherkasova and Hechtman 2009; Barry et al. 2003). ADHD is successfully treated with stimulants such as methylphenidate (MPH) and amphetamine (AMP) which are particularly beneficial for immediate symptom relief (Bitter et al. 2012; Wilens et al. 2011), but nonstimulants and antidepressants are also prescribed (Wilens 2006). Both AMP and MPH increase DA synaptic availability but act at different points of the DA release and reuptake sequence (Heal et al. 2012; Challman and Lipsky 2000). They also modulate norepinephrine though to a lesser degree.
In an early MEG study investigating the effects of MPH treatment on resting state activity, Wienbruch et al. (2005) recorded MEG signals from a group of children diagnosed with ADHD. They performed a spectral analysis in sensor space before and after administering MPH and observed an increase in theta power over the left frontal region which correlated with improved scores on a test of attention. The authors suggested that the MPH renders its behavioral effects by increasing motor inhibition in ADHD patients.
In a recent series of studies, Wilson and colleagues (Franzen and Wilson 2012; Wilson et al. 2012, 2013) have explored the neural basis of ADHD, as well as the mechanisms underlying AMP treatment. They recorded MEG signals from adult individuals diagnosed with ADHD in the OFF-medication state (i.e., ~24 h after the last dose), and again after their regular stimulant medication intake (i.e., ON state). This paradigm allowed them to compare neural activity between the ADHD patients and healthy controls in addition to examining effects of AMP. They analyzed the MEG data in the frequency domain and estimated signal sources with a beamformer approach (Van Veen et al. 1997). One study (Wilson et al. 2013) examined broadband oscillations within the Default Mode Network (DMN) (Raichle et al. 2001) during rest. The principal finding was a globally-reduced wide-band power in unmedicated ADHD patients compared to controls in a higher frequency range (i.e., 14–228 Hz) that was estimated to originate in the medial prefrontal region. The only effect of medication was increased alpha power in the medial prefrontal area (Wilson et al. 2013). Another study (Wilson et al. 2012) investigated the neural basis of gamma activity induced by auditory stimuli in adults with ADHD before and after medication administration and in a control cohort. Binaural click trains presented at 40 Hz induced 40-Hz gamma activity estimated to bilateral auditory cortices. The gamma power was significantly attenuated in ADHD patients compared to control participants. However, administration of a regular dose of the AMP-based medication resulted in a significant increase in gamma activity in ADHD patients (Fig. 4). These results suggest that the commonly prescribed stimulant medication normalizes neural activity in response to auditory 40 Hz stimulation. The authors speculated that abnormalities in GABAergic transmission may underlie abnormally low responsivity in ADHD patients in the off-medication state. By the same token, they propose that the beneficial effects of the amphetamine-based medication derive from its modulation of GABAergic circuitry (Wilson et al. 2012).
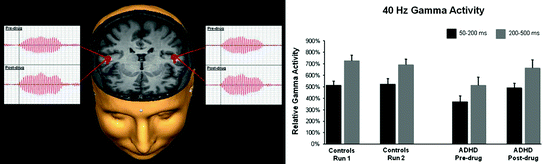
Get Clinical Tree app for offline access
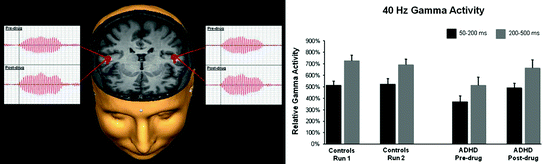
Fig. 4
Left panel Generators of the 40 Hz gamma activity were estimated to the auditory cortices and overlaid onto a 3D rendition of a representative ADHD subject. The source time series (nAm) from each session (pre-drug and post-drug) show the stimulus onset (vertical line) and the 40-Hz gamma response that is stronger after stimulant administration. Right panel
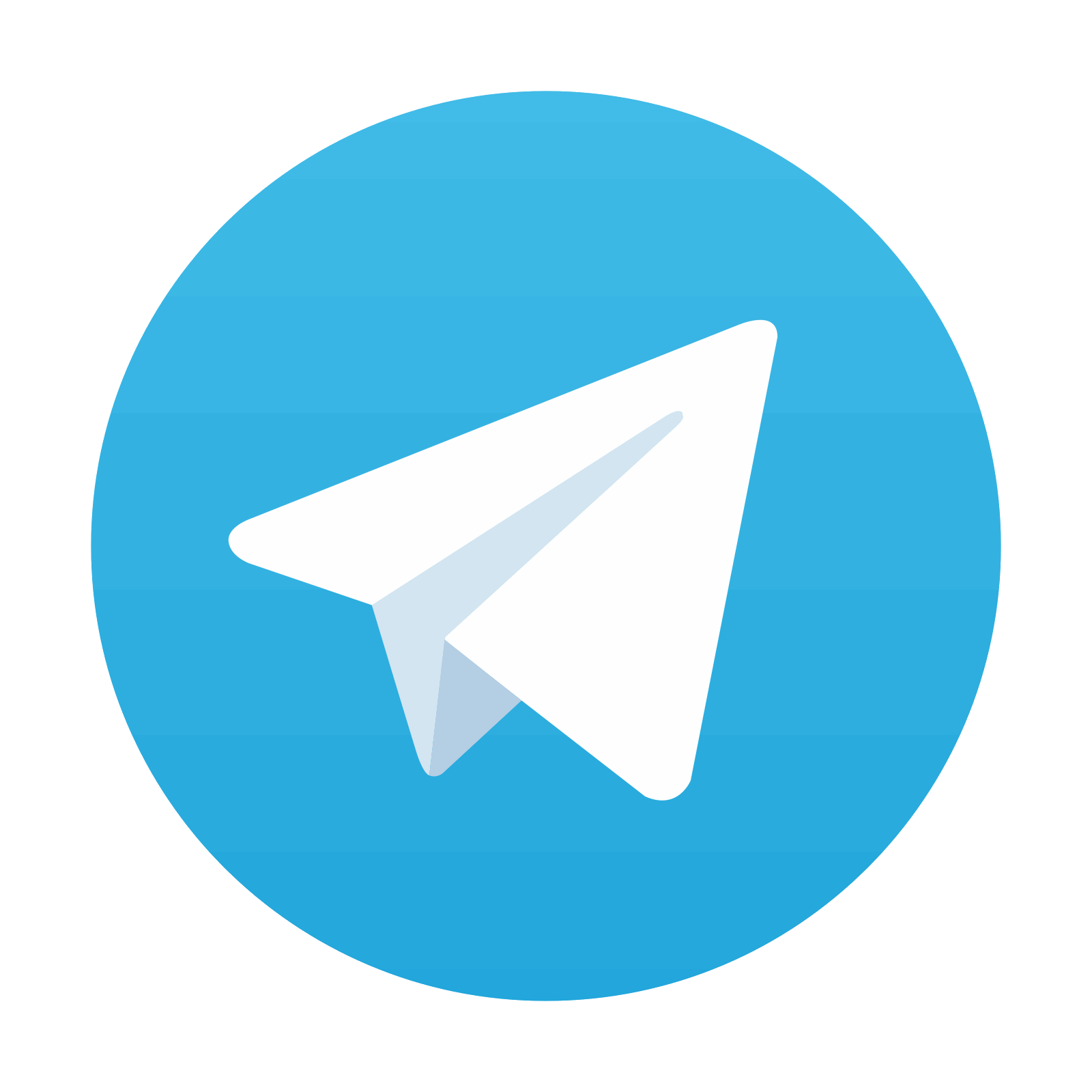
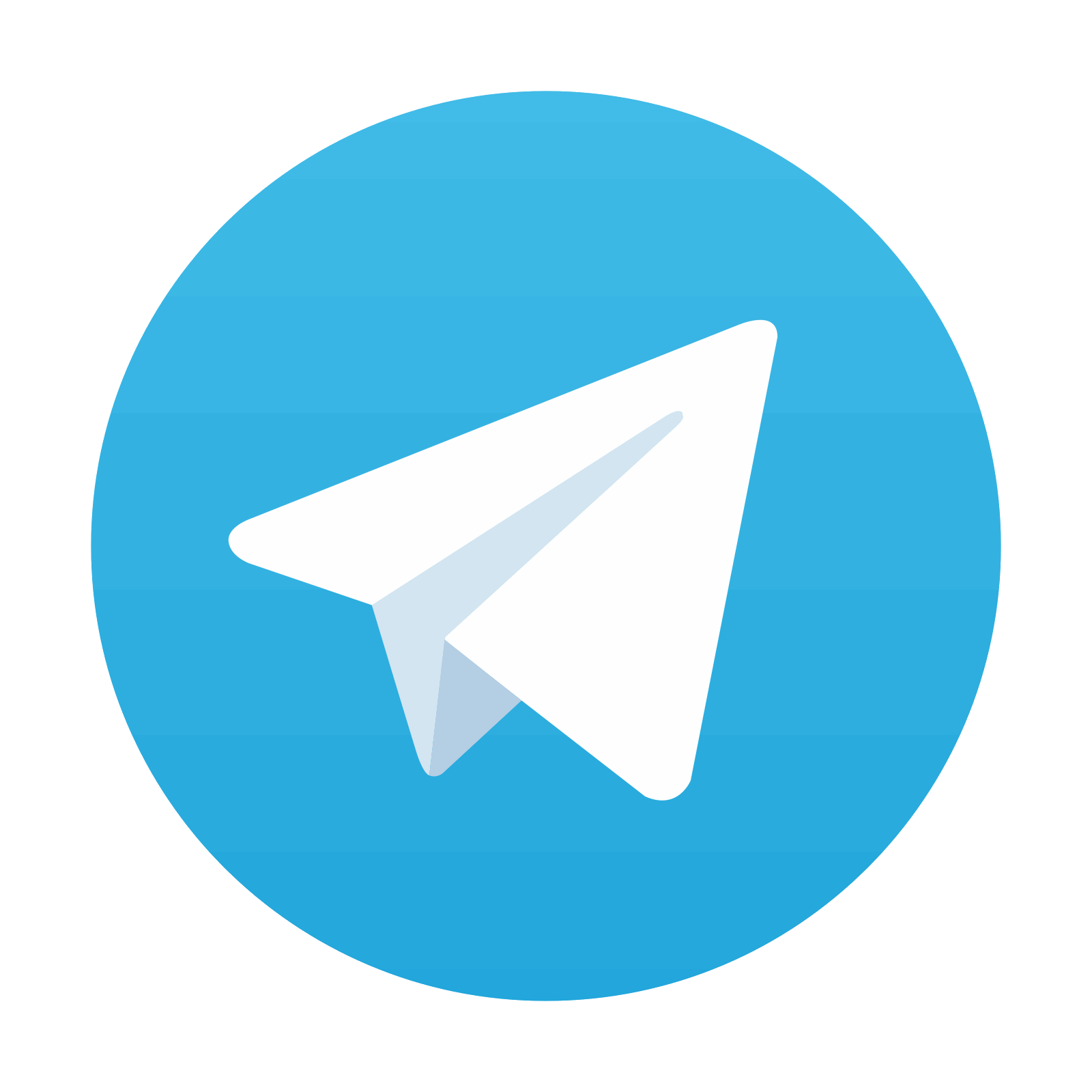
Stay updated, free articles. Join our Telegram channel

Full access? Get Clinical Tree
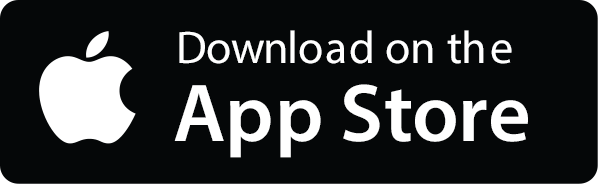
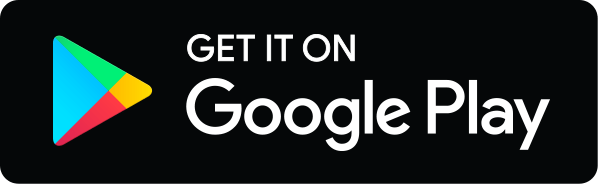
