Renal Scintigraphy
Carlos Cunha Pereira Neto
Dominique Delbeke
![]() |
Renal scintigraphy has been used in clinical nephrourology since the early 1960s. It provides functional and anatomic information, both closely related. Structural information, however, is limited. Functional information is quite unique. For example, a radionuclide study can separately measure renal function on each side.
Common indications of renal scintigraphy are assessment of renal perfusion, renal function, renovascular hypertension (RVH), pyelonephritis, urinary tract obstruction, renal transplantation and acute renal failure. Also, radionuclide cystography (RNC) and scrotal scintigraphy have a well-established place in detecting vesicoureteral reflux (VUR) and torsion epididymoorchitis, respectively. In this chapter, renal radiopharmaceuticals and common protocols in nuclear nephrourology are discussed, followed by case presentations. A good review is available (1,2,3,4).
Radiopharmaceuticals in Renal Scintigraphy
There are many radiopharmaceuticals available for renal scintigraphy. Selection of the radiopharmaceutical depends upon the specific clinical question that is asked. Based on the mechanism of uptake, renal radiopharmaceuticals can be divided into three categories: glomerular, tubular, and cortical agents (5). A basic knowledge about the pharmacokinetics of renal agents is important for correct selection of an agent as well as image interpretation.
Glomerular Agents
These radiopharmaceuticals are filtered by the glomeruli. The two agents commonly used within this category are iodine-125 (125I)-iothalamate and technetium-99m (99mTc)-diethylenetriamine pentaacetic acid (DTPA).
125I-iothalamate is used only for measurement of 125I-glomerular filtration rate (GFR); it is not useful for imaging owing to the poor imaging quality of 125I.
99mTc-DTPA is promptly distributed throughout the extracellular fluid space after intravenous injection. It has only 2% to 6% binding to plasma proteins and does not enter the cells. 99mTc-DTPA has an extraction efficiency of about 20% with each pass through the kidneys, with peak renal activity 3 to 4 minutes postinjection. Overall, approximately 90% of the injected dose is excreted into urine by glomerular filtration in the first 2 hours. 99mTc-DTPA is used to assess renal blood flow, function, and drainage of the pelvicalyceal systems ureters. Having only glomerular filtration with no tubular reabsorption or secretion, 99mTc-DTPA is commonly used to measure GFR.
Tubular Agents
Radioiodinated orthoiodohippurate (OIH, hippuran) and 99mTc-mercaptylacetyltriglycine (99mTc-MAG3) are cleared from the kidneys mainly by tubular secretion.
99mTc-MAG3 has replaced 131I-OIH for renal imaging in most institutions. Having optimal imaging properties, 99mTc-MAG3 provides superior renal functional images with reasonable anatomic evaluation. It has 79% to 90% protein binding in plasma. Renal clearance of MAG3 is 89% by active tubular secretion and 11% glomerular filtration in animal studies (3). It has an extraction efficiency of 50% to 60% with each pass through the kidneys. Although the renal extraction efficiency of 99mTc-MAG3 is less than 131I-OIH, high blood concentrations of 99mTc-MAG3 due to significant protein binding compensate for low extraction, and both radiopharmaceuticals have almost identical renal uptake and excretion on time activity curves. 99mTc-MAG3 is used to measure effective renal plasma flow (ERPF) and assess renal perfusion and function. It is especially recommended for renal scintigraphy of patients with decreased renal function and of infants. The adult recommended dosage is 5 to 10 mCi, intravenously.
Cortical Agents
There are two renal cortical agents available: 99mTc-dimercaptosuccinic acid (DMSA) and 99mTc-glucoheptonate (GH). A fraction of injected radiopharmaceuticals are fixed into the normal renal parenchyma, allowing detection of cortical abnormalities such as acute pyelonephritis, scar, infarct, and congenital abnormalities; differential renal function can also be calculated.
99mTc-Dimercaptosuccinic Acid
Following intravenous administration, 99mTc-DMSA has 75% serum protein binding within the first 6 hours, with about 5% to 20% urinary excretion in 2 hours and 37% in 24 hours (5,6,7). Because of the slow renal excretion of radiotracer, pelvicalyceal systems are not visualized. 99mTc-DMSA has 40% to 50% renal cortical localization, and maximum activity is observed 3 to 6 hours postinjection. Cortical uptake is principally in the proximal convoluted tubules. The suggested adult dosage is 2 to 5 mCi administered intravenously. Renal planar and/or single photon emission tomography (SPECT) images are usually obtained 2 to 4 hours after injection.
99mTc-Glucoheptonate
Following intravenous injection, 99mTc-GH is excreted by glomerular filtration (80% to 90%) and tubular secretion (10% to 20%). About 25% to 40% of the injected dose is in urine within 1 hour and 70% within 24 hours. Up to 15% of the injected dose is retained in the kidneys by binding to the proximal convoluted tubules. Therefore this radiopharmaceutical is useful for early dynamic functional images (0 to 30 minutes) and delayed cortical imaging (2 to 3 hours). The suggested dose for adults is 10 to 15 mCi administered intravenously.
Protocols in Renal Scintigraphy
It is important to obtain renal images in a standard manner. The patient should be well hydrated prior to imaging to prevent false-positive studies. In adults, oral hydration with two glasses (500 mL) of water is adequate. Patients should void just prior to the procedure. A large field-of-view camera with a low-energy, high-resolution, parallel-hole collimator is used.
The patient lies supine on the imaging table with the camera under the table viewing the kidneys and bladder. In renal transplant patients, the camera will be over the transplant anteriorly.
The patient lies supine on the imaging table with the camera under the table viewing the kidneys and bladder. In renal transplant patients, the camera will be over the transplant anteriorly.
After tight bolus injection of radiopharmaceutical (99mTc-DTPA, 99mTc-MAG3, or 99mTc-GH), renal images are obtained in flow phase and sequential functional phase. The flow phase, also called the radionuclide angiogram, evaluates renal perfusion during the first pass of radiotracer through the kidneys. Flow images are acquired 2 to 4 seconds per frame for 60 seconds, starting immediately after injection. It is followed by the sequential functional phase for 30 minutes. Functional images show physiologic radiotracer uptake and excretion. In a normal study, flow to the kidneys is prompt and symmetrical. Maximum renal activity is seen 4 to 6 seconds after peak aortic activity on flow phase. Functional images reveal maximum parenchymal uptake 3 to 5 minutes into the study; excreted activity is in the renal pelves and bladder at the same time. There will be gradual radiotracer washout from renal parenchyma and collecting system as the study progresses. No significant cortical activity should be seen at 30 minutes.
Using scintigraphic data, computer analysis is performed to calculate differential (split) renal uptake and to generate time/ activity curves for each kidney. A region of interest (ROI) is drawn around each kidney. A background ROI is placed in curvilinear fashion along the inferolateral aspect or in semilunar fashion along the lateral aspect of each kidney. Background activity is subtracted from the renal activity to eliminate the contribution of overlying soft tissue activity from the total renal count. The renal uptake at 1 to 3 minutes (before activity is excreted into the collecting systems) is used to calculate split renal function. Normally, the relative contribution of each kidney to total renal activity is 45% to 55%.
A normal renal time/activity curve (renogram) has a characteristic configuration. An initial sharp upslope represents sudden appearance of activity within the renal vasculature in the first 30 seconds. The second portion of upslope is less steep and owing to radiotracer accumulation in the renal parenchyma, reaching a peak in 3 to 5 minutes, followed by a downslope due to excretion of radioactivity into the pelvicalyceal system. Using a renogram curve, many indices have been described. Three commonly used indices are the time to peak cortical activity, the ratio of cortical activity at 20 minutes over peak cortical activity, and the percentage of residual renal or cortical activity at 30 minutes. Normally, the ratio of cortical 20-minute activity/peak activity is less than 0.30 on a 99mTc- MAG3 renogram. Values above 0.30 suggest cortical retention.
Renal Cortical Imaging
99mTc-DMSA or 99mTc-GH is used for renal cortical imaging. Planar images with low-energy, high-resolution collimators are obtained 2 to 4 hours after intravenous injection of radiopharmaceutical. Image magnification (e.g., with pinhole collimators) may be needed in children. Standard images are posterior, right posterior oblique, and left posterior oblique views. SPECT with a multihead camera has a higher detection rate for renal lesions than conventional planar or pinhole collimator techniques (8,9). Therefore renal SPECT should be obtained, if possible, in patients with a clinical suspicion of acute pyelonephritis, especially in children under 3 years of age.
A normal study shows a smooth renal contour with homogeneous cortical activity. Less uptake in the medulla and no activity in the collecting system are expected on high-resolution images (10). With 99mTc-GH imaging, activity may be seen in the renal pelvis if images are obtained early or an obstruction is present. Flattening of the superolateral aspect of the left kidney due to splenic impression may be observed.
Diuretic Renography
Dilatation of the upper urinary tract (hydronephrosis) is usually detected on renal ultrasound or CT scan and needs further evaluation. Mechanical obstruction will cause gradual deterioration of renal function if it is not treated.
Diuretic radionuclide renography is used to evaluate the presence or absence of obstruction in a dilated system.
In order to standardize the techniques of diuretic renography, the members of the Society for Fetal Urology and the Pediatric Nuclear Medicine Council of the Society of Nuclear Medicine have recommended the “Well-Tempered Diuretic Renogram” (WTDR) protocol for neonates (infants) with hydronephrosis (11). With some modification, this technique can be applied to children. Recently, a panel of experts from the scientific committee of the 9th International Symposium on Radionuclides in Nephrourology published an article entitled “Consensus on Diuresis Renography for Investigating the Dilated Upper Urinary Tract” (12).
The technique is as follows:
Adequate hydration: In the WTDR protocol, infants get oral hydration with formula or water ad libitum beginning 2 hours prior to the study. Intravenous hydration with diluted normal saline (NS) solution (D5.3NS or D5.25NS) at a rate of 15 mL/kg over a 30-minute period is started 15 minutes prior to radiopharmaceutical injection and is continued at a rate of 200 mL/kg/24 hour for the duration of the examination. In adults, oral hydration with 500 mL of water or orange juice about 30 to 60 minutes prior to radiopharmaceutical injection is necessary.
Bladder catheterization: A full bladder may prevent adequate drainage of upper collecting systems and can cause a false-positive study for obstruction. The bladder is catheterized routinely in the WTDR protocol. In adults and older children, bladder catheterization may not be necessary if there is no evidence of vesicoureteric reflux, neurogenic bladder, or lower tract obstruction.
Radiopharmaceuticals: 99mTc-DTPA and 99mTc-MAG3 have been commonly used for diuretic renography. Currently, the agent of choice is 99mTc-MAG3, which provides superior images, especially in neonates, infants, and patients with diminished renal function.
Diuretic dosage and timing: Furosemide is given intravenously to induce forced diuresis. The high urinary flow rate induced with furosemide washes out radiopharmaceutical from dilated collecting systems if there is no significant obstruction. A prerequisite to a valid diuretic renogram is
reasonable renal function that can respond to furosemide. The recommended furosemide dosage is 1 mg/kg in infants, 0.5 mg/kg in children, and 40 mg in adults, given intravenously (11,12). It is injected 20 to 30 minutes after radiotracer injection or when the dilated renal pelvis/ureter is full. Then imaging is continued for another 20 to 30 minutes. Functional images, renogram time/activity curves (before and after furosemide injection), and furosemide washout half-time (T½) are used for interpretation.
Angiotensin-Converting Enzyme Inhibitor Renography (Captopril Renography)
Renovascular hypertension (RVH) affects less than 1% of general hypertensive patients and up to 35% of patients with risk factors for RVH. It is defined as high blood pressure caused by renal hypoperfusion, usually due to renal artery stenosis and activation of the renin-angiotensin-aldosterone system (13,14).
Currently, angiotensin-converting enzyme (ACE) inhibitor renography plays an important role in the noninvasive diagnosis of RVH. The principle of this test is scintigraphic demonstration of physiologic changes due to transient blockage of the activated renin angiotensin system with an ACE inhibitor, such as captopril. Protocols for performance and criteria for interpretation of captopril renography may vary among different nuclear medicine departments. Two protocol/interpretation guidelines are available: (a) a report of the working party group for patient selection and preparation in conjunction with diagnostic criteria of renovascular hypertension with captopril renography, a consensus statement (15,16), and (b) a consensus report on ACE inhibitor renography for detecting RVH by an international panel of experts (17).
In our institution, the following protocol, which is very close to the guidelines of the consensus report on ACE inhibitor renography for detecting RVH, is employed. 99mTc-MAG3 is routinely used. Patients should be off any ACE inhibitors for 48 hours, and no solid meal is allowed 4 hours prior to the procedure. First, a captopril renogram is obtained. The patient is hydrated with 10 mL/kg of water, orally, if possible. Then an intravenous line is inserted and hydration is continued with normal saline at a rate of 4 mL/min during the entire procedure. Baseline blood pressure (BP) and pulse rate (PR) are recorded. Captopril 50 mg is given orally. Then BP and PR are recorded every 15 minutes for 1 hour. The intravenous infusion rate is increased if there is a significant drop in BP, and a physician is immediately notified. One hour after captopril administration, the patient voids and then lies supine on the imaging table. Renal imaging is started immediately following intravenous administration of 99mTc-MAG3, 10 mCi, and furosemide, 40 mg (for adults). Flow and functional images are obtained for 30 minutes, and time/activity curves are generated.
If the captopril renogram is abnormal, a baseline (without captopril) study is obtained after 48 hours following the same protocol. Occasionally, ACE inhibitor renography is performed while the patient is on chronic administration of ACE inhibitor, and no additional dose of ACE inhibitor is given prior to the procedure. This procedure is known to be less sensitive than “a true captopril renogram.”
In renovascular hypertension, a captopril MAG renogram has a typical appearance consisting of delayed time to peak (Tmax >5 min) and cortical retention of radiotracer. These findings will markedly improve or normalize on the baseline study. Using 99mTc-DTPA, the most important finding is a decrease in differential renal function compared to baseline study (17).
Direct Radionuclide Cystography
This procedure is used for the detection and follow-up of vesicoureteral reflux. It is more sensitive and delivers about 100 times less radiation than contrast voiding urethrocystography (VCUG). However, anatomic resolution is poor with the radionuclide cystogram.
The technique is as follows:
99mTc-sulfur colloid (0.5 to 1.0 mCi) or 99mTc-pertechnetate (1.0 mCi) is used. The patient voids prior to the procedure. The patient lies supine on the imaging table and, under aseptic conditions, the bladder is catheterized using a Foley catheter (or an infant feeding tube in small children). A gamma camera equipped with a low-energy, high-resolution collimator is placed under the imaging table with the bladder and kidneys in the field of view. The radiotracer is administered through the catheter into the bladder and then flushed with sterile water. The bladder is gradually filled with normal saline by gravity (the bottle of normal saline is 70 to 90 cm above the bladder). Imaging is started at the time of normal saline infusion. The computer monitor is closely watched for vesicoureteral reflux, and the bladder volume at the time of reflux is recorded. Saline infusion is discontinued when the patient feels full with slight discomfort or estimated bladder volume is reached [estimated bladder volume in milliliters = (patient’s age + 2) × 30. Infants often urinate around the catheter when the bladder is full; otherwise the bladder is drained via the catheter. In older children, a bedpan is placed under the patient and images are obtained during and after voiding. Normally there is no reflux from the bladder into the ureter renal pelvis.
Scrotal Scintigraphy
Scrotal scintigraphy with a blood-flow and blood-pool imaging agent is performed to differentiate testicular torsion from epididymitis/epididymoorchitis. It should be performed urgently when the request is received, because testicular viability is endangered if the surgical treatment for torsion is delayed. Interpretation of nuclear scrotal images is based on expected normal symmetry in the hemiscrotum. Therefore proper positioning of the scrotum under the camera is important.
The patient may be pretreated with sodium or potassium perchlorate orally, 5 to 8 mg/kg, to reduce radiation to the thyroid gland if this does not delay imaging. The radiopharmaceutical agent of choice is 99mTc-pertechnetate, injected intravenously. The dosage is 15 to 20 mCi for an adult and is
adjusted for body weight in children, with the minimum dosage being 5 mCi (18).
adjusted for body weight in children, with the minimum dosage being 5 mCi (18).
The technique is as follows:
The patient lies supine with legs abducted on the imaging table. A scrotal sling or a rolled towel under the scrotum is used to provide support and symmetrical positioning of the scrotum in the center of the field of view. The penis is taped back onto the lower abdomen. A low-energy general-purpose parallel-hole collimator is placed as close as possible to the patient. Image magnification is necessary in children, using a converging collimator. Dynamic anterior flow images are acquired 2 to 5 seconds per frame for 60 seconds, starting immediately after intravenous bolus injection of the radiopharmaceutical, followed by 1- and 5-minute anterior static images, each for 500,000 counts. Another static image is obtained with a thin lead strip over the medial raphe to separate the right from the left hemiscrotum and a lead shield under the scrotum to block background thigh activity. This aids in identifying subtle asymmetrical perfusion within the scrotum.
Dynamic flow images clearly delineate the iliac–femoral arteries. However, small vessels of the scrotum and testes are not usually seen. Injected 99mTc-pertechnetate is loosely bound into the plasma protein and approximately 75% to 80% of radiopharmaceutical is rapidly diffused into the extravascular space. Therefore static images obtained at 1 and 5 minutes postinjection reveal the distribution of radiopharmaceutical in the extracellular space, which reflects tissue perfusion. This activity is normally symmetrical in the scrotum, with intensity similar or slightly greater than thigh uptake.
Although Doppler color-flow ultrasound becomes the primary imaging modality for evaluation of the scrotum, ultrasound may not differentiate acute epididymitis from early torsion (less than 7 hours after the onset of pain). Moreover, difficulties are often experienced in performing ultrasound on patients with acute scrotal pain. Radionuclide scintigraphy should be of great value in these special situations and may also be used as an adjunct to sonography.
Case 6.1
History: A 14-year-old boy was brought to the emergency room for acute left scrotal pain of 12 hours’ duration. Clinical assessment and laboratory findings were inconclusive. A testicular scintigram (Figs. 6.1 A to 6.1 D) was obtained to assess the vascular integrity of the testicles.
Findings: On the radionuclide angiogram (Fig. 6.1 A), symmetrical activity is demonstrated in the region of the scrotum. One-minute, 5-minute, and static lead-shielded images (Figs. 6.1 B, 6.1 C and 6.1 D) show homogeneous scrotal activity. No asymmetry or abnormal area of increased or decreased uptake is seen. Normal penile blood pool (arrow) and urinary bladder activity (arrowhead) are easily identified.
Discussion: Symmetrical scrotal activity on the flow study and homogeneous tracer distribution on delayed static images with no area of decreased uptake indicate an intact vascular supply and exclude a left hemiscrotal flow abnorm- ality.
Scrotal scintigraphy, consisting of dynamic blood flow and static blood pool images, is a useful procedure for evaluation of vascularity and perfusion of the scrotum and its contents.
It is to be noted that a normal scrotal scintigram in a patient with acute hemiscrotal pain may represent manual or spontaneous detorsion, torsion of the testicular appendage, referred pain, or even an emotional disorder (19).
Diagnosis: Normal scrotal scintigram.
Case 6.2
History: A 24-year-old healthy male presented with acute right scrotal pain of approximately 4 hours’ duration. There was no history of trauma. Although the clinical impression was that of probable epididymoorchitis, a testicular scintigram was requested to evaluate for acute testicular torsion (Figs. 6.2 A, 6.2 B and 6.2 C)
Findings: One-minute, 5-minute, and raphe marker/thigh lead shielded static images (Figs. 6.2 A, 6.2 B and 6.2 C) demonstrate a large area of diminished activity within the right hemiscrotum.
Discussion: The testicular scintigram reveals diminished radiopharmaceutical activity in the right hemiscrotum on both flow (not shown) and static images. Common causes of diminished hemiscrotal activity include early torsion, hematocele, hydrocele, and hernia. In the above clinical setting, findings are most consistent with early torsion.
Acute scrotal pain demands prompt evaluation. The main differential considerations are testicular torsion, which needs immediate surgical intervention to salvage the testis, and epididymitis epididymoorchitis, which can be managed medically. The usual clinical presentation is acute testicular pain in a male in his early teens. These patients may also have a low-grade fever, nausea, or vomiting. Urinary symptoms are uncommon and more likely to be encountered in cases of epididymitis or abscess. When the clinical diagnosis is unclear, scrotal scintigraphy has proven to be a safe and sensitive method for differentiating testicular torsion from epididymitis epididymoorchitis and should be performed immediately, if available.
Testicular torsion occurs in 1 in 4,000 males, commonly in adolescents and young adults. Usually an underlying anatomic abnormality predisposes the patient to torsion of the testis, with subsequent testicular ischemia/infarction. The most common anatomic variation occurring in testicular torsion is complete covering of the testes and epididymis by the tunica vaginalis, with absence of the normal uncovered area participating in the scrotal attachment. This leads to the “bell clapper” deformity, where the testicle and epididymis are freely movable on a vascular pedicle. This anatomic variant is frequently bilateral.
The arterial supply to the testis, epididymis, and tunica vaginalis (scrotal contents) is through the testicular artery (which arises from the aorta just below the renal arteries), the deferential artery (a branch of the internal iliac or vesical artery), and the cremasteric artery (a branch of the inferior epigastric artery). These vessels course throughout the spermatic cord. Although anastomosis is present among these arteries, the testicular artery is the major blood supply to the testis. The venous drainage of the scrotal contents is via the paminiform plexus to the internal spermatic veins. The right spermatic vein empties into the inferior vena cava, and the left joins with the left renal vein. Blood supply to the scrotum (scrotal skin and dartos) is from the superficial external pudendal artery (a branch of the femoral artery), the anterior scrotal artery (a branch of the deep external pudendal artery), and the posterior scrotal artery (a branch of the internal pudendal artery).
It is noted that the testis epididymis and the scrotum have separate blood supplies, and there is no anastomosis between these two. Therefore, in testicular torsion, a twist in the spermatic cord disrupts blood flow to and from the testis and endangers the viability of the testis. Scintigraphically, this is seen as an area of decreased activity in the hemiscrotum on flow and static tissue images. Decreased activity may be subtle, especially in the early stage of torsion; therefore a comparison of both the right and left hemiscrotum is very important in order to detect asymmetry, suggesting altered supply on one side. On the other hand, an inflammatory process such as epididymitis causes local hyperemia and is recognized on scintigraphy as an area of increased activity.
Diagnosis: Early right testicular torsion.
Case 6.3
History: A 41-year-old male with prior left orchialgia presented with a 4-day history of acute left scrotal pain and swelling; he was admitted with a tentative diagnosis of epididymoorchitis. A radionuclide scrotal scintigraphy was obtained (Figs. 6.3 A and 6.3 B).
Findings: The symptomatic left hemiscrotum demonstrates a rim of increased activity with a photopenic center on the dynamic flow study (Fig. 6.3 A). Similar findings are also present on the static blood pool image (Fig. 6.3 B).
Discussion: The pattern of a photopenic center with a surrounding rim of activity is not specific and may be seen in late testicular torsion, abscess, hematoma, or tumor. Clinical correlation narrows the differential.
In testicular torsion, the viability of the testis depends on the degree of torsion and the duration of symptoms. It is apparent that an incomplete twist (180 degrees) in the spermatic cord jeopardizes the blood flow to the testis less than a complete twist (360 degrees). Initially, venous flow is obstructed, leading to gradual congestion and edema. This is followed by cessation of arterial flow, and testicular infarction ultimately occurs. The prompt diagnosis of testicular torsion is imperative for salvaging of the involved testicle. Surgical intervention has been successful in approximately 100% of patients with torsion lasting less than 4 hours, as compared with 20% at 10 hours. Hormonal and spermatic function has been preserved in most patients with a duration of torsion between 6 and 12 hours; after 24 hours, viability is rare. Time-dependence of testicular survival dictates that scrotal scintigraphy be performed immediately upon request.
Late testicular torsion (missed torsion) indicates nonviability of the testis. Scintigraphically, this is seen as a photopenic area with a surrounding rim of intense activity (bull’s-eye sign) in the symptomatic side of the scrotum (Figs. 6.3 A and 6.3 B).
The photopenic center is the affected testis, and the surrounding rim of increased intensity represents reactive hyperemia in the dartos. Low-grade reactive hyperemia activity in the dartos may be present within 24 hours of torsion, but viability is still possible.
Diagnosis: Late left testicular torsion with acute hemorrhagic necrosis.
Case 6.4
History: A 22-year-old male presented with acute right scrotal pain; an emergency nuclear scrotal scintigram was obtained (Figs. 6.4 A and 6.4 B).
Findings: The dynamic flow images (Fig. 6.4 A) demonstrate asymmetrical activity in the scrotum, with the symptomatic right hemiscrotum having more activity than the left. Static images also reveal diffuse increased uptake on the right side (Fig. 6.4 B).
Discussion: The increased activity in the right hemiscrotum represents hypervascularity, which is commonly caused by an inflammatory process such as epididymitis/epididymoorchitis. Inflammatory conditions are the most common cause of acute scrotal pain, as they are more frequent than testicular torsion even in patients under 20 years of age. Epididymitis/epididymoorchitis is usually an extension of infection from the lower urinary tract.
An inflammatory process is associated with increased vascularity and hyperperfusion. Inflammation of the epididymis is seen as an area of increased radiopharmaceutical activity in the lateral and superior aspects of the expected location of the testis on blood flow and blood pool images. When inflammation involves the testis (so-called epididymoorchitis), the entire hemiscrotum will show increased activity. The spermatic cord of the symptomatic hemiscrotum may also reveal increased uptake secondary to increased blood flow and vascularity.
Occasionally, spontaneous or manual detorsion causes diffuse hemiscrotal activity and may mimic epididymoorchitis. If epididymal inflammation incites hydrocele formation, it will cause a photopenic area medial to epididymal activity. This pattern may be confused with missed torsion.
Diagnosis: Epididymoorchitis.
Case 6.5
History: A 3-year-old female presented with a 1-year history of urinary tract infection (UTI). On initial workup, renal ultrasound was normal, and contrast VCUG showed grade III vesicoureteral reflux (VUR) on the right side. She was started on prophylactic antibiotic with no new episode of UTI. One year later, a follow-up radionuclide cystogram (RNC) was obtained (Fig. 6.5 A).
Findings: Images reveal vesicoureteral reflux on the right side (Fig. 6.5 A), which appears during the middle filling phase at a bladder volume of approximately 115 mL. The reflux reaches into the intrarenal collecting system (arrow) with no evidence of gross dilatation of the ureter or renal pelvis. Low-pressure reflux is seen during bladder filling, and high-pressure reflux is seen during voiding. No residual activity is present in the ureter or renal pelvis on postvoid image. There is no reflux on the left side in this case.
Discussion: After the initial episode of a documented UTI, a child younger than 3 to 5 years of age should have assessment of the upper and lower urinary tract to evaluate for developmental and acquired abnormalities. Renal/bladder ultrasound is the most commonly used modality for evaluation of the upper urinary tract and bladder.
The lower urinary tract is evaluated with contrast VCUG or RNC. A contrast VCUG provides anatomic detail of the lower urinary tract and will disclose the presence or absence of vesicoureteral reflux, paraureteral/bladder diverticulum, or posterior urethral valve. It is recommended for the initial evaluation of the lower urinary tract in children with urinary tract infection, especially boys. Radionuclide cystography is recommended for follow-up of patients with reflux and evaluation of siblings of patients with reflux; it may also be used in initial assessment of girls with UTI.
RNC is more sensitive than contrast VCUG for the detection of reflux, and a volume as little as 0.25 mL can be detected. Continuous imaging during RNC allows detection of intermittent reflux that may be missed with contrast VCUG and delivers 50 to 200 times less radiation. However, diverticula may not be identified, and the urethra cannot be evaluated.
The most common abnormality detected in children with UTI is VUR, reported in 29% to 55% of cases. Primary VUR, the most common cause of reflux in children, is due to a short intramural segment of the ureter, causing vesicoureteral incompetence. As children grow, the intramural segment of the ureter lengthens, and reflux often resolves spontaneously by 5 years of age.
Grading VUR is important in the management of patients with reflux. The International Reflux Study (IRS) classification is commonly used for grading and is based on radiographic findings on contrast VCUG. It characterizes reflux as follows: grade I, reflux limited to the ureter; grade II, reflux extending into the ureter and pelvicalyceal system without dilatation; grade III, mild or moderate dilatation of the ureter and renal pelvis but without blunting of fornices; grade IV, moderate dilatation and/or tortuosity of the ureter with moderate dilatation of the pelvicalyceal system and obliteration of sharp angles of fornices but with preservation of papillary impression; and grade V, severe dilatation and tortuosity of the ureter and pelvicalyceal system with loss of papillary impression in most calyces.
The chance of spontaneous resolution of VUR depends upon the severity of reflux. Resolution occurs in 83% of grade I, 80% of grade II, and 46% of grade III reflux. Grades IV and V reflux require surgical intervention.
Because anatomic detail is suboptimal in radionuclide cystography, the IRS grading system cannot be applied accurately. A good method of conveying the significance of reflux is to describe the extent of reflux (ureter versus ureter and renal pelvis), the presence or absence of gross dilatation in the ureter pelvis if possible, the time of occurrence (filling versus voiding), bladder volume when reflux is first seen, and the presence or absence of residual activity in the collecting system after voiding.
On Figure 6.5 B, significant bilateral VUR is seen in a 4-year-old girl with a history of bilateral grade II VUR.
Another way of diagnosing VUR is with an indirect RNC, as shown in Figure 6.5 C.
The glucoheptonate renogram demonstrates essentially no function in the left kidney. Good function is seen in the right kidney; however, prominent activity in the region of the right renal pelvis raises the possibility of an extrarenal pelvis or nonobstructive hydronephrosis. The last three images show markedly decreased bladder activity with a sudden simultaneous appearance of activity in the left ureter and renal pelvis. Delayed and anterior abdominal/pelvic images (not shown) did not demonstrate an ectopic left kidney. The indirect RNC method does not require bladder catheterization, as bladder filling is physiologic; however, it does require good renal function for excretion of the radiopharmaceutical and the child’s cooperation to avoid voiding during bladder filling. Renal uptake and excretion of activity is associated with gradual filling of the bladder. When radioactivity is cleared from the upper collecting system, the patient is asked to void. Any reappearance of activity in the ureter or renal pelvis indicates VUR. Indirect RNC is not commonly used because it is less sensitive than direct RNC for the detection of reflux.
Diagnosis: Right-sided vesicoureteral reflux reaching into the intrarenal collecting system.
Case 6.6
History: A 10-year-old female with a history of recurrent UTI presented with left flank pain and fever. 99mTc-DMSA scintigraphy was performed to evaluate the possibility of pyelonephritis (Figs. 6.6 A and 6.6 B).
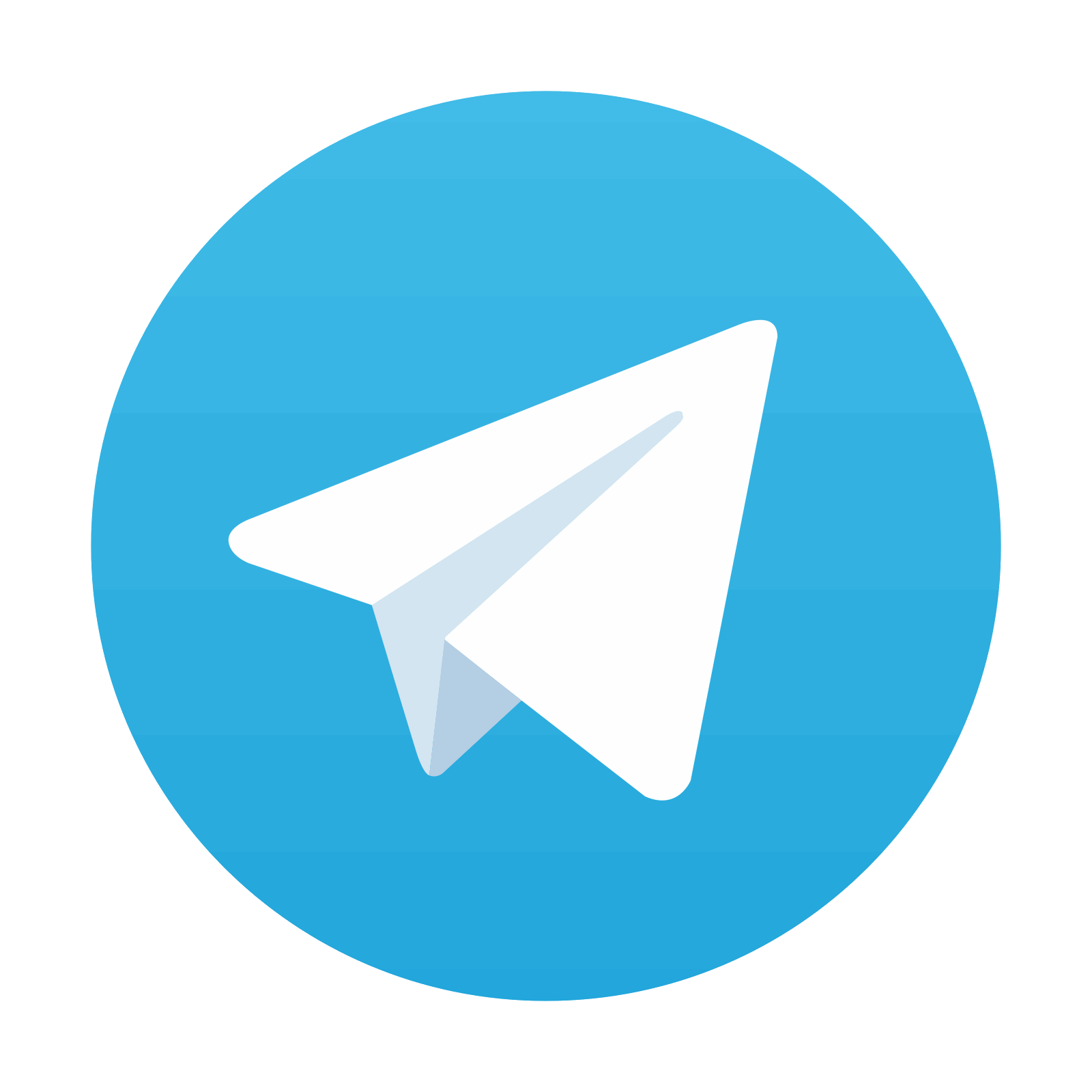
Stay updated, free articles. Join our Telegram channel

Full access? Get Clinical Tree
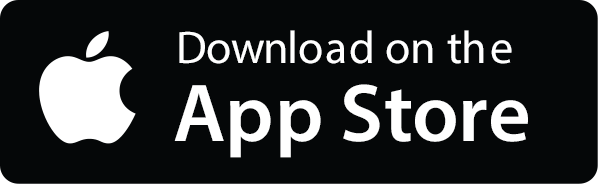
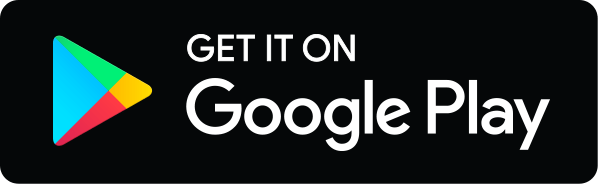