Fig. 1
(a–f) Normal kidney anatomy. Coronal TSE T2 (a). Normal kidneys have a relative long T2 time, and they appear homogeneously hyperintense compared to the liver. Coronal FFE T1 (b). The cortex is slightly higher in signal intensity than the medulla. (c–f) Axial dynamic contrast-enhanced GRE T1 before (c) and after administration of gadolinium (d– f). During the arterial phase (30 s after gadolinium injection), the cortex has a dramatically higher signal intensity than the medulla (d). The nephrographic phase (60 s after gadolinium injection) further demonstrates corticomedullary differentiation but less evident that in the arterial phase (e). The late phase (180 s after gadolinium injection) demonstrates a homogeneous signal intensity of the renal parenchyma with high signal intensity in the renal pelvis due to excretion of gadolinium (f)
A standard renal study protocol contains a T2W sequence to differentiate cysts from solid masses and to show the caliber and contour of excretory tracts. Renal cysts are homogenously hyperintense (Fig. 2), while the presence of hypointense nodularity, septations, or variable degrees of hypointense content are possible signs of malignancy (Figs. 3, 4 and 5). Conventional spin echo sequences have long acquisition time so motion artifacts may reduce image quality. To avoid this problem, echo-train imaging (e.g., turbo spin echo, fast spin echo according to different vendors) with fat suppression is usually used. In addition, in patients with limited breath-hold capacity, the use of respiratory triggering could reduce respiratory artifacts in the abdomen. Half-Fourier reconstruction (e.g., HASTE, SSFSE) could be added to echo-train sequences to further decrease acquisition time in patients without breathing control. These sequences last less than a second on a per-slice base, acquiring only one half of the k space, while other data are mathematically reconstructed. They offer a rapid survey of abdominal structures, particularly cysts and excretory system, but they are less sensitive for small, low-contrast lesions due to their low signal and contrast-to-noise ratio and their typical blurring effect (Zhang et al. 2003).
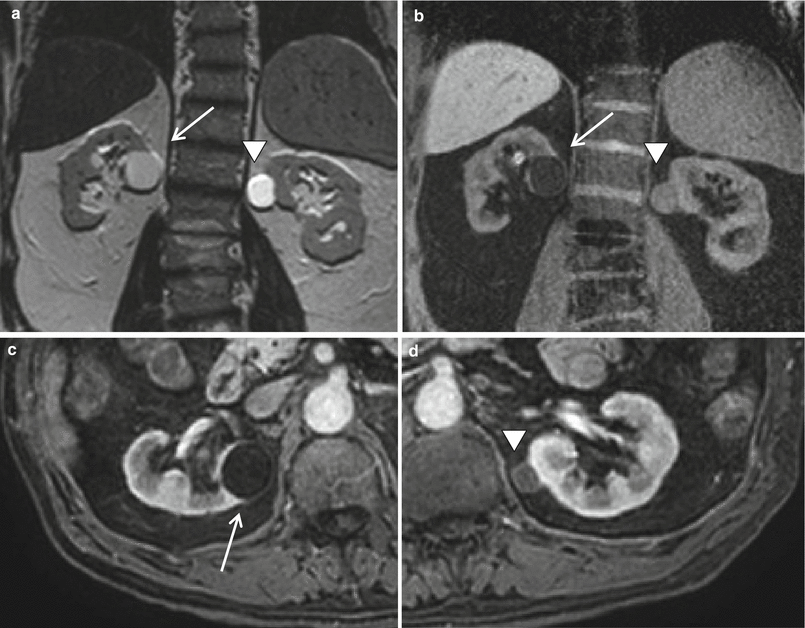
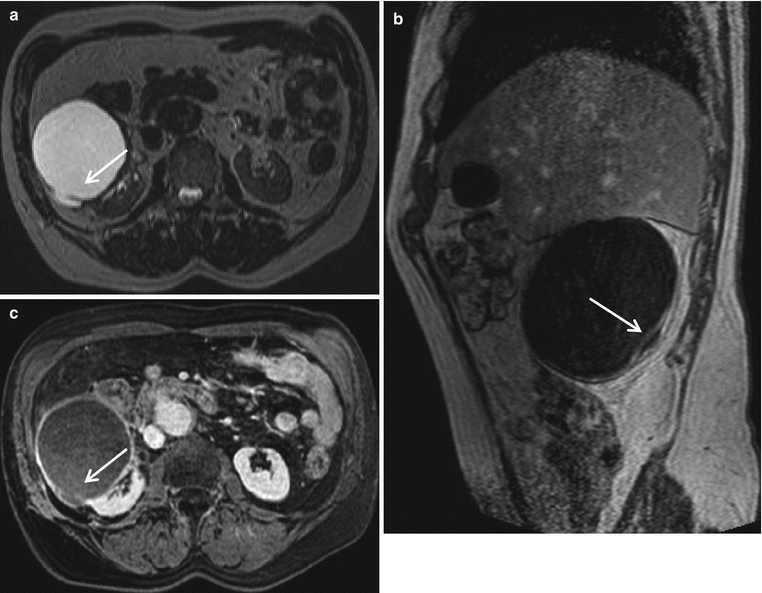
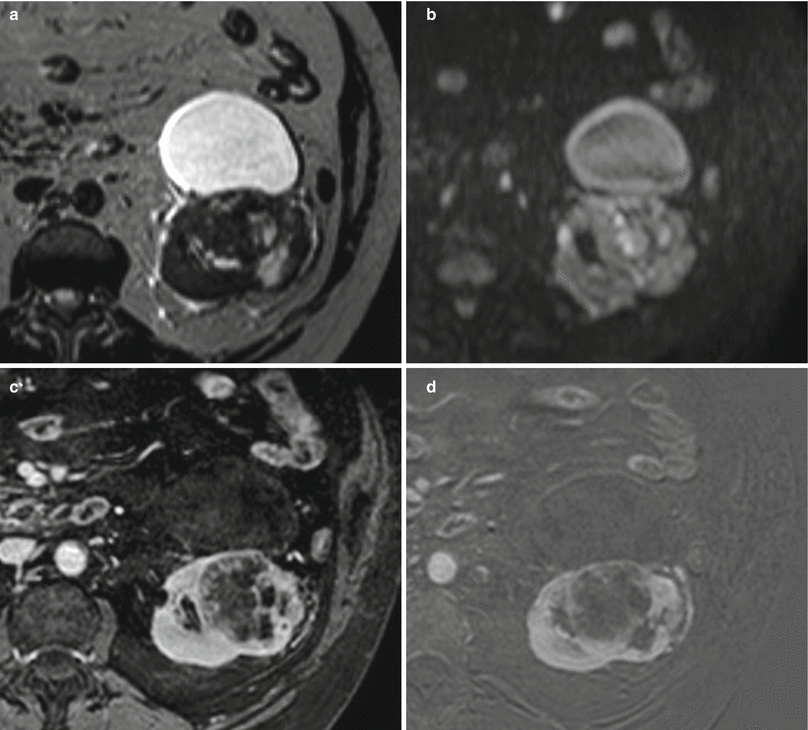
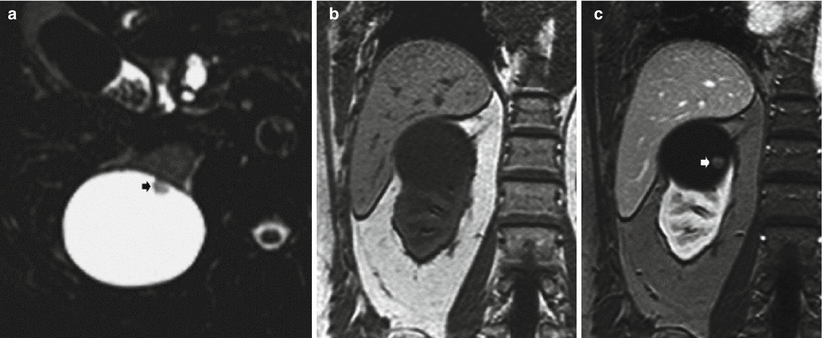
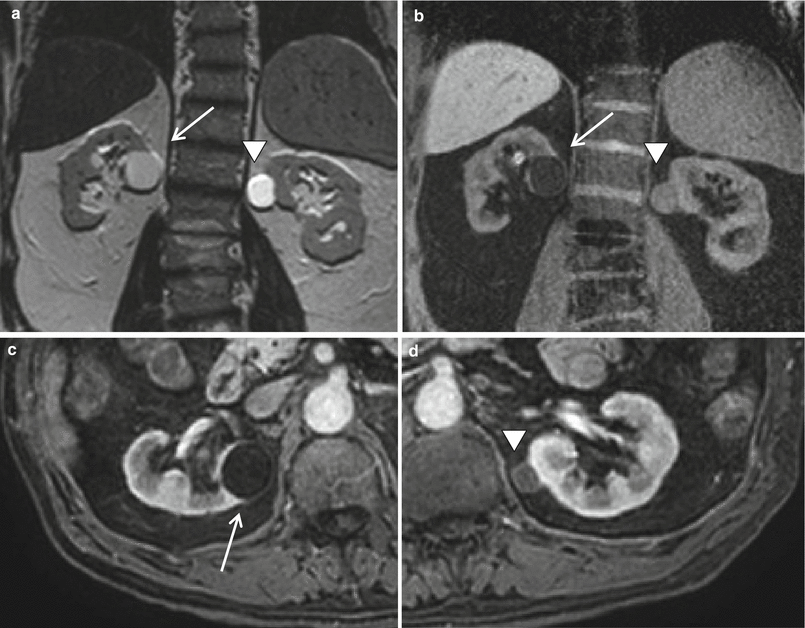
Fig. 2
(a–d) Simple cyst versus hemorrhagic cyst. Coronal T2-weighted (a) and fat suppression GRE T1-weighted (b) MR images show an exophytic simple cyst on the right (arrow) and an exophytic hemorrhagic cyst on the left kidney (arrowhead). The simple cyst shows a dark T1 and bright T2 signal intensity, while the hemorrhagic cyst has a strong hyperintensity on T2 weighted images and a slightly less hyperintensity on T1-weighted images. Both lesions do not have enhancement as shown on axial T1-weighted images after the administration of contrast material (c–d)
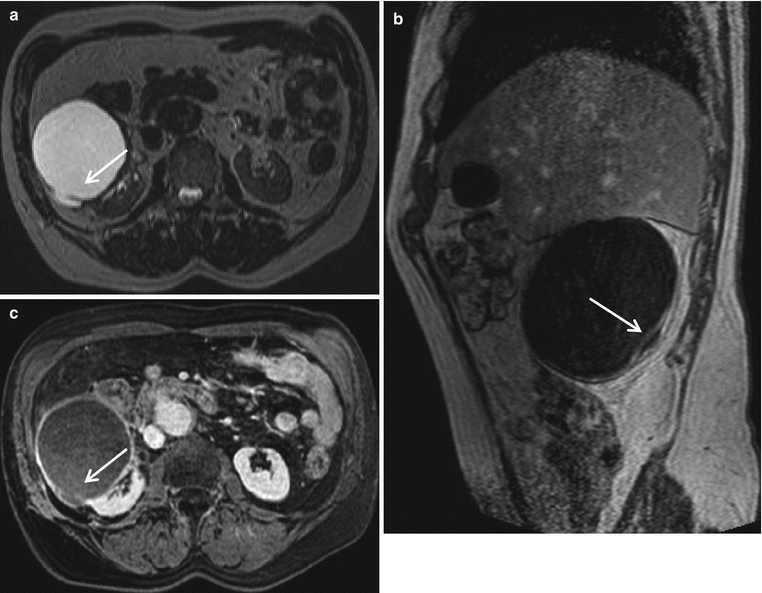
Fig. 3
(a–c) Complex cystic lesion (Bosniak IIF) in a 75-year-old man. Axial T2-weighted image (a) shows a hyperintense cystic lesion which contains hairline thin septa (arrow). The lesion appears hypointense on sagittal T1-weighted image (b). Axial contrast-enhanced T1-weighted image shows thin enhancing wall, corresponding to Bosniak IIF (c)
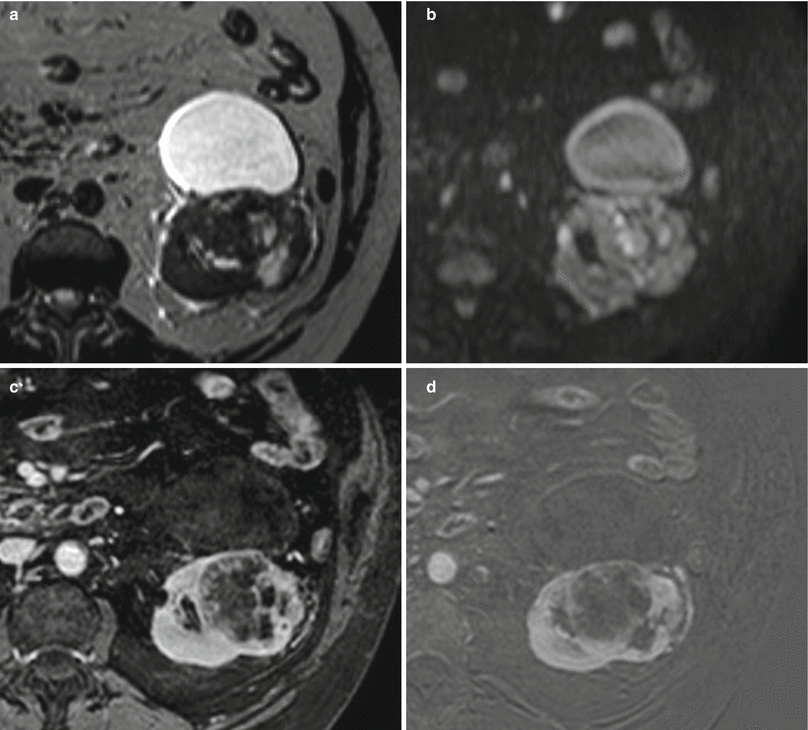
Fig. 4
(a–d) Complex cystic lesion (Bosniak IV) in a 71-year-old woman. Axial T2-weighted image (a) shows an inhomogeneous lesion in the lower pole of the left kidney. The lesion has restricted signal on DWI (b). Axial T1 contrast-enhanced image (c) and subtraction mask (d) show thick enhancing wall and septa with intralesional solid coins representing a malignant cystic lesion (Bosniak IV). A simple cystic lesion is also seen
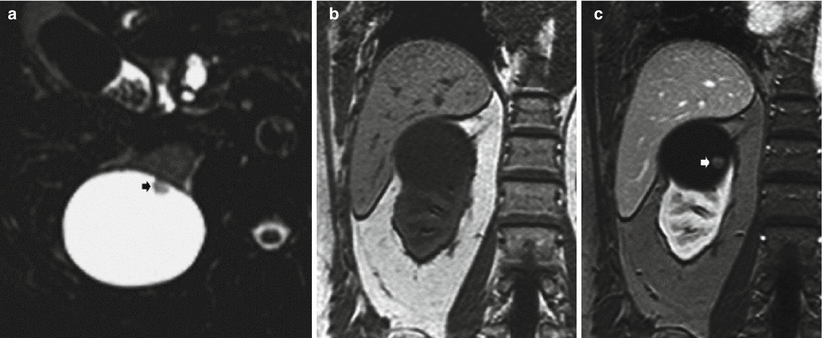
Fig. 5
(a–c) Malignant renal cyst (Bosniak IV) in a 41-year-old man. Axial TSE T2W (a) image shows a hypointense mural nodule (arrow). Coronal GRE T1W images, obtained before (b) and after (c) gadolinium-based contrast agent administration, demonstrate mural nodule enhancement
On T1-weighted (T1W) sequences, normal renal cortex is slightly higher in signal intensity than medulla, and medulla has a similar signal intensity compared to muscle (Fig. 1). T1W sequences are needed for a complete lesion characterization. Most cysts have a low signal intensity on T1W, appearing hypointense compared to renal parenchyma, while hemorrhagic, proteinaceous, melanin, or fat content ones have a high signal intensity on T1W, appearing hyperintense (Fig. 2). Multishot gradient-echo techniques are most commonly used. A repetition time (TR) of 120–200 ms can provide full coverage of the kidney in a single breath hold, allowing dynamic contrast-enhanced imaging when fat suppression is applied. T1W spoiled gradient-echo imaging performed before, during, and after intravenous gadolinium-based contrast agent administration is essential for contrast enhancement pattern demonstration. Comparison between pre- and postcontrast image, obtained in corticomedullary and nephrographic phases, permits differentiation of solid masses from complicated cystic lesions, while delayed postcontrast imaging depicts tumor extension in the perinephric fat and in venous structures. While with 2D gradient-echo sequences, smaller lesions can be missed or insufficiently characterized, the use of a three-dimensional (3D) acquisition allows the generation of multiplanar reformations, greatly enhancing the multiplanar assessment of renal lesions (Sun and Pedrosa 2009; Zhang et al. 2003).
Detection of lesion enhancement may be difficult when precontrast T1W signal intensity is high or intermediate; in this situation subtraction technique can be helpful. The non-enhanced image set can be subtracted from contrast-enhanced ones, using each contrast phase as a template. A misregistration of data set can result in areas of apparent enhancement as the appearance of a hyperintense rim in the direction of motion artifact (Sun and Pedrosa 2009).
Frequency-selected fat suppression technique is a useful tool to depict fat component within lesions, which is a typical feature of angiomyolipoma (AML). Gradient-echo sequences, performed with specific echo time (TE) values, can image water and fat either in-phase or opposed-phase within the same voxel. Opposed-phase sequences are useful for the characterization of diseased tissue when mixture of fat and water protons is present within the same voxel like in intracellular lipids of some clear cell renal carcinoma. The cancellation of fat and water signals in the same image voxel causes a loss of signal intensity on opposed-phase images (Outwater et al. 1998). On opposed-phase sequences, a chemical shift artifact (India ink artifact) appears as a black line between renal parenchyma and retroperitoneal fat and disappears when a mass is extending beyond renal capsule, even if it does not involve adjacent structures. The same artifact appears at the periphery of a bulk fat content area, highlighting the presence of gross intralesional fat as in angiomyolipoma (Zhang et al. 2003; Prasad et al. 2008a).
Diffusion-weighted imaging (DWI) measures the Brownian motion which represents the mobility of water molecules within tissues. DWI has been long used in neuroradiology, but abdominal applications were limited by physiological motion of intra-abdominal structures and the heterogeneous composition of abdominal organs. New fast imaging techniques, like Half-Fourier reconstruction and motion compensation, have improved the quality of abdominal DWI allowing DWI evaluation of the kidney. DWI uses a pulse sequence (T2-weighted spin echo sequence) and two equal size gradients, separated by a 180° radiofrequency (RF) pulse. The first gradient is the dephasing (diffusion sensitizing) gradient, while the second gradient, after the RF pulse, is the rephasing gradient. In stationary water molecules, as in tissues with restricted diffusion, the extra rotation induced by the second gradient will be identical to the first one, and all molecules will be in-phase at the end of the second gradient, generating a high signal. In contrast, in nonstationary water molecules, the second extra rotation will not be identical to the first, and the molecule will be out of phase, generating a lower signal. The most common sequence used for DWI is a single-shot echo-planar imaging. The diffusion weighting of the sequence is usually indicated by the b-value, expressed in s/mm2. Only higher b-values enable an estimate of true diffusion of water molecules (Petralia and Thoeny 2010). Visual assessment of DWI images alone may have some limitations. Because DWI is a T2W sequence, due to the T2 shine-through effect, normal tissues show high signal intensity even in higher b-values (Fig. 6). Indeed the signal intensity on high b-value images depends both on the diffusion of water molecules and on the intrinsic tissue T2 relaxation time. To avoid misinterpretation, the visual assessment of DWI images should be supported by the quantitative analysis provided by the apparent diffusion coefficient (ADC) map calculated by a workstation software from two images acquired with different gradient duration and b-values. A tissue with a significant T2 shine-through effect is hyperintense on DWI images at a high b-value appearing in color shades of light gray on the corresponding ADC map, with a high ADC value. Conversely, high signal intensity on the high b-value with a corresponding color shade of dark gray on ADC maps with a reduced ADC value indicates a cellular tissue (Petralia and Thoeny 2010).
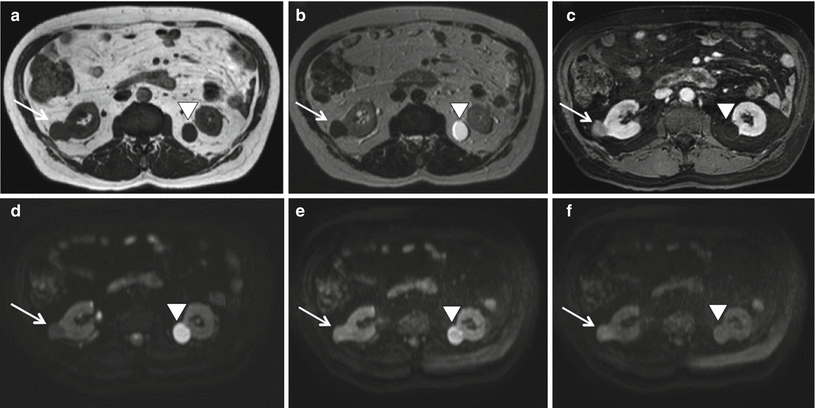
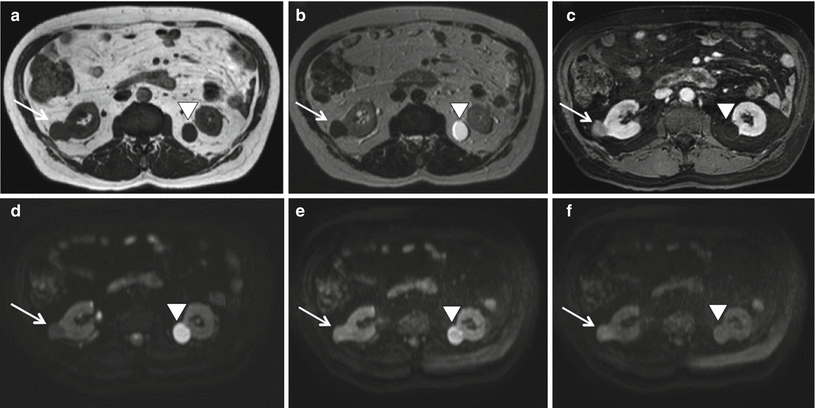
Fig. 6
(a–f) Mesenchymal renal neoplasm in a 70-year-old man. T1W images show a hypointense lesion (arrow) in the right kidney (a) which remains hypointense in T2W image (b) and has a strong enhancement after the administration of contrast material (c). The lesion restricts the signal on DWI images as demonstrated by the increasing signal with increasing b-values (d–f). At histologic examination after surgical excision, this mass was determined to be a mesenchymal neoplasm of the kidney. The same images demonstrate the presence of a simple cyst (arrowhead) on the left kidney which does not restrict the signal on DWI images: the lesion becomes hypointense with increasing values of b
DWI have shown considerable promise in several renal clinical applications: renal lesion detection and characterization as benign or malignant, assessment of renal cell cancer subtype and nuclear grade, and evaluation of treatment response in patients with advanced renal cell cancer undergoing targeted chemotherapy. DWI may have a role also in the assessment of malignant lymph nodes from urogenital tumors, which are expected to have restricted diffusion and low ADC values. In addition, ADC values correlate well with renal function both in native and transplanted kidney (Zangh et al. 2008; Palmucci et al. 2012).
The lack of standardization in imaging technique is the major drawback of DWI which slightly hampers its use in clinical practice.
Magnetic resonance angiography (MRA) is performed for renal vasculature evaluation. Today, 3D contrast-enhanced magnetic resonance angiography (3D CE MRA) is widely used, replacing invasive angiography in many circumstances. It is a 3D fast spoiled gradient-echo sequence performed in conjunction with intravenous bolus injection of contrast medium. Perfect bolus timing is essential to get maximum arterial gadolinium concentration during the center of k space. There are several techniques available to correctly coordinate the initiation of bolus injection with the initiation of scanning. The most widely used is an automatic pulse sequence, named bolus track, care bolus, and fluoroscopic triggering depending on vendors, which allows direct visualization of contrast arrival in the aorta with a real-time 2D acquisition, typically updated every second. The CE MRA acquisition can then be triggered manually or automatically with the visualization of contrast arrival.
The typical 3D CE MRA sequence also has the effect of suppressing the background tissue not exposed to gadolinium through relatively high flip angles and short repetition times. Additional background suppression can be achieved by adding fat suppression pulses to the sequence or by subtracting the contrast-enhanced sequence from a precontrast mask acquisition. The latter has the limit of misregistration artifacts in patients whose breath holding is different in the two acquisitions.
CE MRA produces 3D data set that can be reconstructed into an unlimited number of projections using different slab thickness, orientations, and rendering methods. Maximum intensity projection, multiplanar reconstructions, and 3D volume rendering are the most commonly used postprocessing techniques (Zhang and Prince 2004; Glockner and Vrtiska 2007).
The nephrogenic systemic fibrosis risk in patients with severely compromised renal function – estimated glomerular filtration rate <30 mL/min/1.73 m2 – has given a new boost in MRI without the use of gadolinium-based contrast agent (Perez-Rodriguez et al. 2009). Early studies emphasized noncontrast sequences, such as time of flight or phase contrast (Glockner and Vrtiska 2007). The image quality of these flow-sensitive techniques is limited by diminished flow in patients with vascular stenosis or parenchymal disease and by motion artifacts due to respiration during acquisition times that are too long for breath hold.
Alongside the two conventional unenhanced MR angiographic techniques, several novel techniques have been developed for the evaluation of renal arteries, including electrocardiograph steady-state free precession (SSFP) and arterial spin labeling. SSFP angiography, named as balanced FFE (fast field echo), TrueFISP (fast imaging with steady-state precession), FIESTA (fast imaging employing steady-state acquisition), and TrueSSFP according to the vendor, is a gradient-echo-based sequence which maintains steady-state longitudinal and transverse magnetization by applying a series of equidistant RF pulses. The image contrast is T2W/T1W. The main advantages of this sequence are the short acquisition time and its relative independence from flow. The major drawback is its susceptibility to field heterogeneities. For arterial spin labeling sequence, a slab-selective IR pulse is applied to the image plane, commonly in combination with ECG gating. Combining this with an appropriate inversion time, the background signals are suppressed, while only inflowing blood from outside the slab provides high signal intensity. It has been reported that this sequence has high sensitivity and high specificity in the detection of high-grade renal artery stenosis and in the detection of transplant renal artery stenosis (Satoru et al. 2011).
MR urography (MRU) allows urinary tract evaluation. It is recommended in pregnant, pediatric, and young patients, but some intrinsic limitations, such as insensitivity in renal calcification detection, long imaging time, motion sensitivity, and low spatial resolution, limit its clinical applications. MRU may be performed with a static fluid or an excretory technique.
Static-fluid MRU is performed using T2W sequences, exploiting the long T2 relaxation time of urine, without contrast material administration (Figs. 7 and 8). Thick-slab single-shot fast spin echo is optimal for obtaining heavily T2W images in a short time. For urinary tract peristalsis evaluation and stenosis confirmation, multiple images can be obtained and played as a cine-loop. Single acquisitions have to be separated by 5–10 s to prevent radiofrequency saturation of the tissue and to avoid progressive signal loss. The signal intensity of soft tissue can be adjusted by modifying the echo time or using fat suppression. Three-dimensional respiratory-triggered sequences can be used too, obtaining volume rendering (VR) and maximum intensity projection (MIP). Static-fluid MRU is helpful to evaluate the urinary tract course and diameter, even in cases of obstructed or poorly excreting kidney. Oral negative contrast agent administration could be requested to avoid signal intensity visualization of bowel contents.
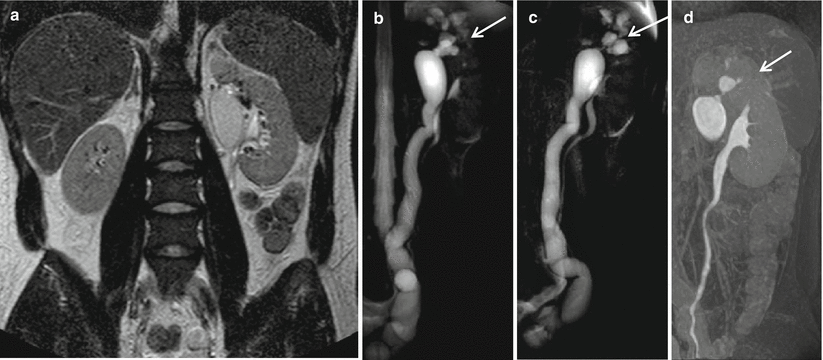
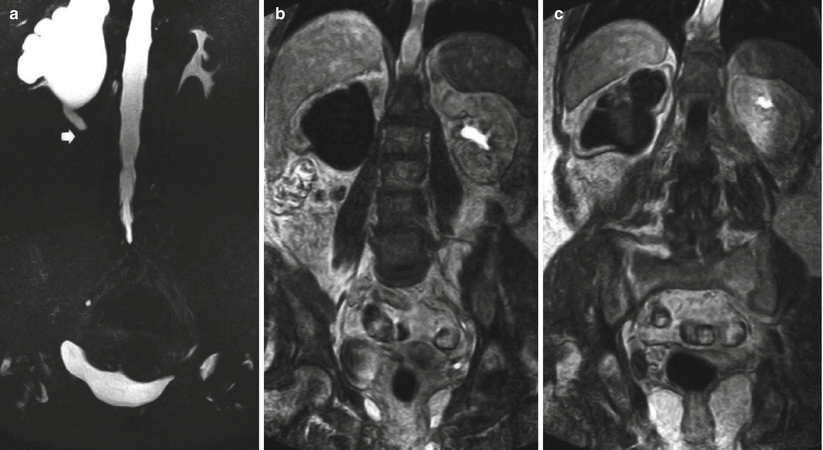
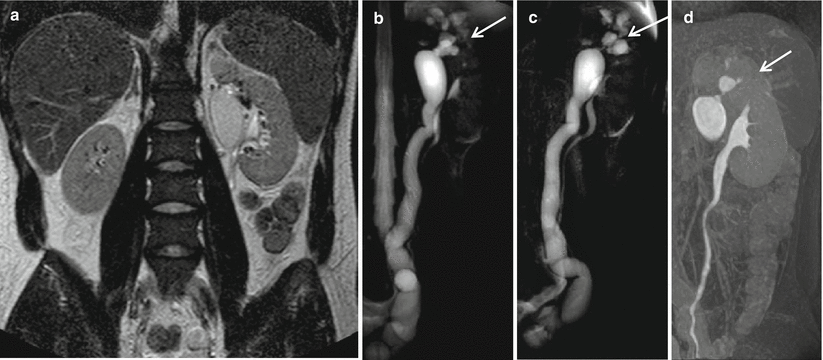
Fig. 7
(a–d) Static-fluid MRU and excretory MRU in a patient with left double renal pelvis and double left ureters. Coronal TSE T2W shows a left dilated upper renal pelvis but a regular lower renal pelvis (a). Static-fluid MRU shows a dilated and tortuous left ureter; the corresponding upper renal pelvis is dilated. At the upper pole of the left kidney, a hyperintense focal area, connected to the renal upper pelvis, is also visible (arrow) (b–c). Excretory MRU demonstrates the normal caliper of the lower renal pelvis and the corresponding ureter with regular contrast excretion. The dilated upper renal pelvis has a delayed contrast excretion (d)
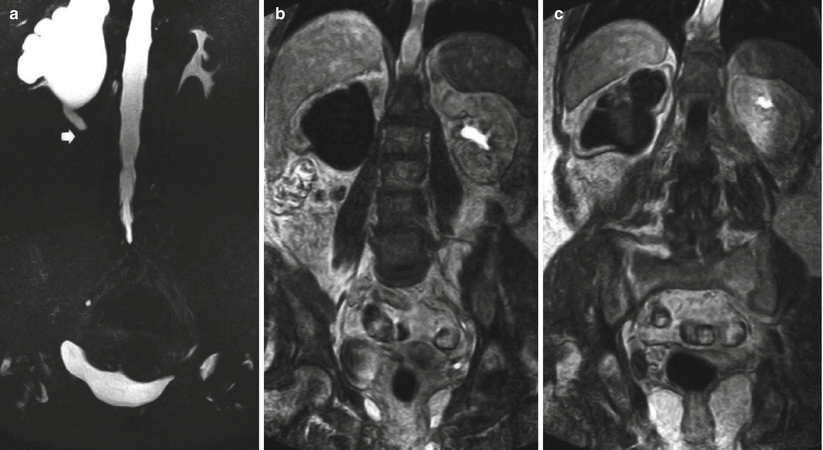
Fig. 8
(a–c) MR urography (MRU) in a patient with right ureteral carcinoma. Static-fluid MRU. Dilatation of the right upper urinary tract with the evidence of obstruction at the level of the upper right ureter (arrow) (a). Excretory MRU after Gd-based contrast agent injection. Absent contrast excretion on the right kidney which reveals third-grade hydronephrosis and regular contrast excretion on the left urinary tract (b, c)
Excretory MRU is performed using T1W sequences obtained after gadolinium-based contrast agent administration during the excretory phase (Figs. 7 and 8). For excretory MRU, the thinnest partitions that maintain acceptable SNR and anatomic coverage should be used allowing a through-plane resolution of 2–3 mm. Breath holding is essential for excretory phase imaging, as acquisition time lasts 20–30 s. The use of parallel imaging techniques reduces acquisition times with a modest penalty in SNR. For patients with limited breath-holding capacity, the urinary tract can be imaged in segments. Coronal 3D gradient-echo fat suppression sequences are employed. Using a standard dose of contrast material (0.1 mmol/kg), gadolinium quickly becomes concentrated in the urine and can give T2* effects, reducing the signal intensity of urine. To avoid this artifact, a low dose of gadolinium-based contrast material (0.05 mmol/kg) is advisable. Diuretic administration (0.1 mg/kg of furosemide) can improve the quality of excretory MRU by enhancing urine flow and giving a better dilution and uniform distribution of the contrast agent through urinary tract. Excretory MRU requires the excretion of contrast medium into the collecting system, and so it does not have a role in the evaluation of patients with obstructed or poorly excreting kidney (Nolte-Ernsting et al. 2001a, b). Furthermore, contrast medium administration is not possible in patients with severely compromised renal function for the risk of nephrogenic systemic fibrosis (Leyendecker et al. 2008).
Because of the excellent diagnostic performance of CT for the diagnosis of many diseases of the urinary tract (e.g., patients with flank pain or hematuria), MRU, to date, has been reserved for patients who cannot receive iodinated contrast material or for whom exposure to ionizing radiation is particularly undesirable. Even in this population, MRU has relatively limited applications due to limited availability of expertise and the technical rigors of producing a high-quality examination.
Currently, MRU is most commonly indicated in children and pregnant patients with dilated collecting systems. In the former group, indications for MRU include preoperative anatomic imaging for the assessment of vascular anatomy, evaluation of a wide range of congenital abnormalities, evaluation of duplex systems, and distinction between pelvicalyceal dilatation and cystic disease. Both anatomic and functional information of the urinary tract can be obtained in a single examination, potentially eliminating the need for other diagnostic examinations such as nuclear scintigraphy. Functional data that can be obtained from excretory MRU include renal transit time, differential renal function, and estimated GFR. Static-fluid and excretory MRU techniques have been shown to be complementary in the evaluation of children. In pregnant patients, physiological dilatation of the right ureter can be distinguished from an obstructive uropathy caused by a calculus without intravenous contrast material. Because most static-fluid MRU techniques are quick and relatively easy to perform, the examination is well tolerated, even in the advanced stages of pregnancy. To avoid potentially nephrotoxic iodinated contrast material, MRU can be used also to evaluate potential renal transplant donors and to assess urologic complications following renal transplantation.
A number of studies have shown that MRU is a useful examination for investigating the causes of urinary tract dilatation and diagnosing urinary tract obstruction (Darge et al. 2008; Silverman et al. 2009; Zielonko et al. 2003). Because MR is relatively insensitive to the detection of calcifications, the diagnosis of ureteral calculi often relies on detecting secondary signs of obstruction such as ureteral dilatation and perinephric fluid. Sometimes a persistent filling defect can be identified. The sensitivity of MRU for ureteral calculi is technique dependent, with higher sensitivities for excretory MRU than for static-fluid T2-weighted techniques. MRU is more sensitive for diagnosing the cause of urinary tract obstruction due to causes other than urolithiasis compared with unenhanced CT. Although the diagnostic confidence of CT urography in the detection of urothelial neoplasm is slightly higher, MRU may have a role when investigating young patients with microhematuria who are at low risk for malignancy (Martingano et al. 2013).
The comprehensive MR protocol used in our institution for kidney evaluation is reported in Table 1.
Table 1
MRI protocol for the evaluation of the kidney
Sequence | Respiratory compensation | Flip angle | TR | TE | Slice thickness | View | |
---|---|---|---|---|---|---|---|
T2W Half Fourier single shot TSE | Breath-hold | 90° | Shortest | 140 ms | 3 mm/ no gap | Axial | Localizer |
T2W information | |||||||
Low signal-to- noise ratio | |||||||
T2W TSE with fat suppression | Respiratory triggering | 90° | Shortest | 140 ms | 3 mm/ no gap | Coronal | More detailed T2 information |
Characterization of cysts, intraparenchymal abscesses, hydronephrosis | |||||||
Detection of solid lesions | |||||||
T1W GRE as a dual echo sequence | Breath-hold | 90° | 3 mm | Axial | Detection of fat | ||
In-phase | Shortest | 2.3 ms | |||||
Opposed-phase | Shortest | 4.6 ms | |||||
Ultrafast single shot EPI sequence with navigator | Breath-hold | 90° | Shortest | Shortest | Axial | Lesion detection | |
b-values (mm/s2): 0; 50; 100; 800; 1,000 | Differential diagnosis between solid and liquid lesions | ||||||
Limited role in lesion characterization | |||||||
Unenhanced T1W GRE sequence | Breath-hold | 10° | Shortest | Shortest | Axial | Used to create subtraction mask | |
T1W GRE sequence for dynamic imaging during the administration of gadolinium | Breath-hold |