Fig. 17.1
Supracondylar fracture involving the metaphysis of the distal humerus. (a, b) This is the most common fracture (arrows) in the pediatric elbow. Note joint effusion
1 Pediatric Fracture Patterns and Mechanisms of Injury
The pediatric bone is mainly composed of woven bone, which has less mechanical strength than mature trabeculated adult bone [4]. The woven bone is highly cellular and porous and contains a large amount of collagen. This results in decreased tensile and compressive strength and predisposes children to fractures rather than ligament injuries [3].
Buckle (torus) fractures result from compressive forces and constitute one of the most common pediatric fractures. They consist of cortical buckling, typically at the metadiaphysis, usually extending only partway around the bone circumference. Buckle fractures most frequently occur in the distal radius, typically after a fall on an outstretched hand. Displacement and angulation are usually minimal (Fig. 17.2) [4]. These stable fractures require only symptomatic treatment with a splint [5]. Greenstick fractures occur when a bone bends before beginning to break, and the fracture does not extend through the entire bone [6]. The cortical break is seen on the convex or tension side, and the fracture line propagates vertically within the medulla without breaking the cortex on the concave or compression side. Greenstick fractures most commonly involve the diaphysis of the radius or ulna, and radiographs typically show an incomplete fracture with angulation deformity (Fig. 17.3). Bowing injury is a plastic deformation, with the bone bent just short of the fracture or failure point [4, 7]. This may result from low-energy bending forces, and the adjacent bone often fractures [5]. Bowing injury most frequently occurs in the ulnar diaphysis and sometimes in the radial or fibular diaphysis. Radiographs show excessive bowing without cortical disruption (Fig. 17.4) [4, 6].
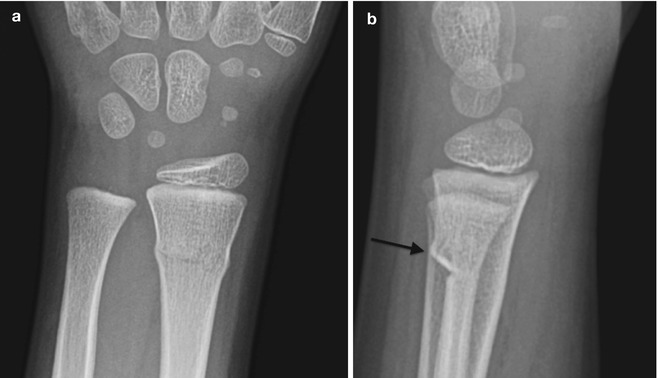
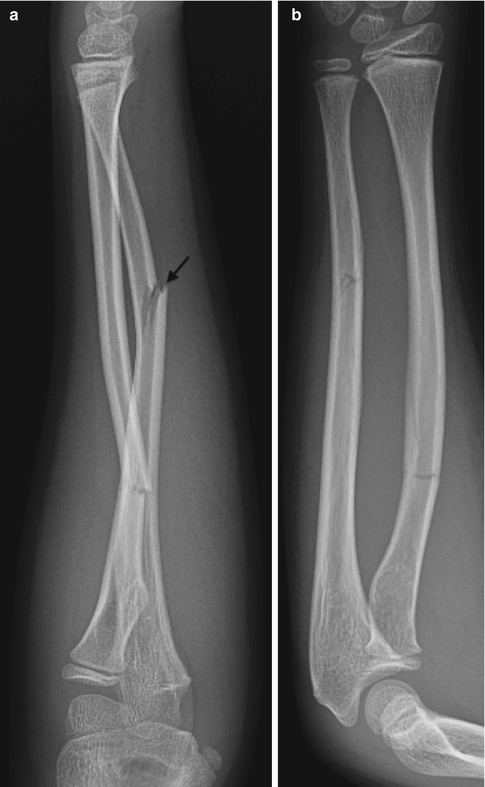
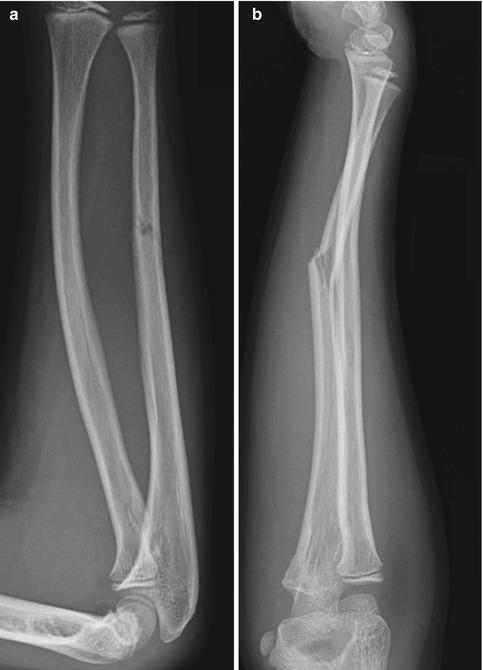
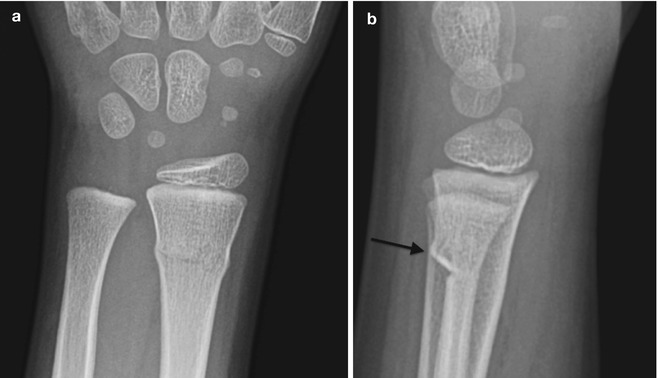
Fig. 17.2
Buckle fracture (arrow) of the distal radial metadiaphysis (a, b)
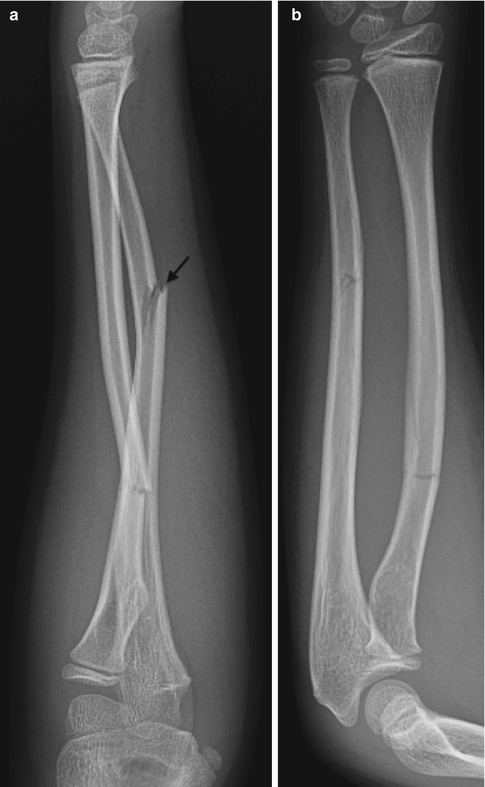
Fig. 17.3
Greenstick fracture of the ulnar diaphysis with vertical extension in the medullary space (arrow). There is also an incomplete transverse fracture of the radial shaft (a, b)
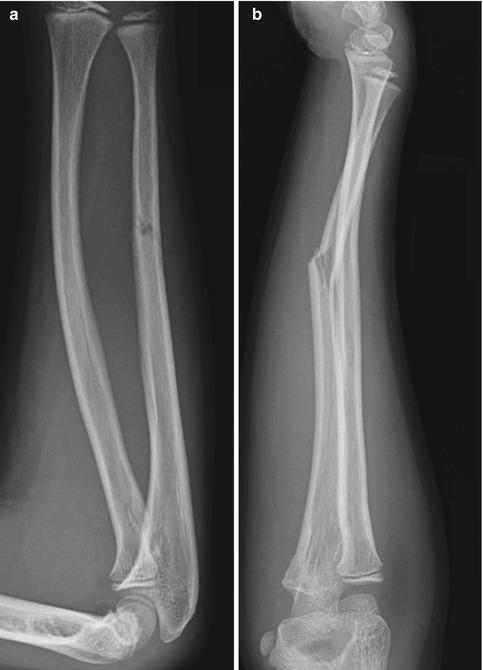
Fig. 17.4
Bowing injury of the radius and greenstick fracture of the ulnar diaphysis (a, b)
Abundant growth cartilage in the physes, epiphyses, and apophyses makes these areas vulnerable to specific injuries. The physis, the major contributor to longitudinal bone growth, is usually injured due to a shearing or avulsive force (Fig. 17.5). The epiphysis, responsible for spherical growth at the ends of the long bones, is primarily subject to compressive forces, which may cause osteochondral injuries (Fig. 17.6). The apophyses, secondary ossification centers at the attachment sites of major tendons, contribute to bone shape and may be injured by tensile forces (Fig. 17.7) [4]. The incidence of growth cartilage injury is highest during periods of maximal growth, such as the pubertal growth spurt. This may be because mineralization lags behind linear growth in rapidly growing bone, resulting in a thicker and more fragile physis. In addition, the increased rate of longitudinal bone growth may cause a temporary disparity with muscle-tendon elongation, resulting in decreased flexibility and increased susceptibility to growth cartilage injury [4].
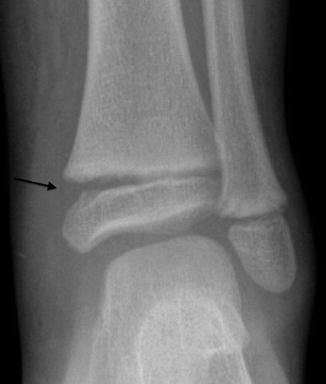
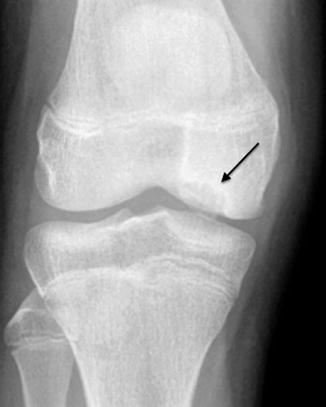
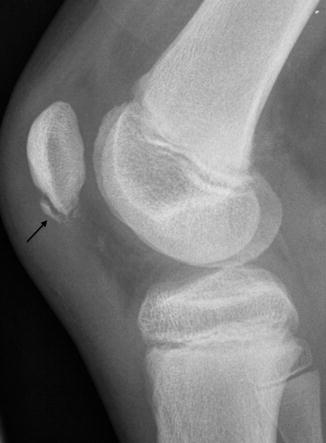
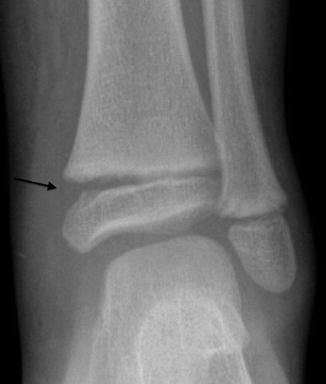
Fig. 17.5
Type I fracture of the distal tibial growth plate with widening of the physis (arrow) and adjacent soft tissue swelling
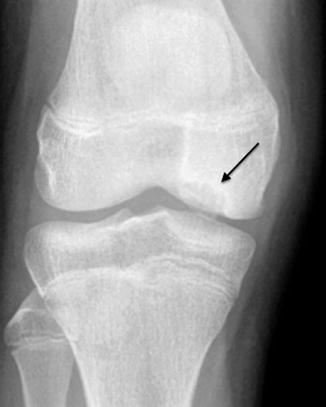
Fig. 17.6
Osteochondral injury of the distal femoral epiphysis (arrow)
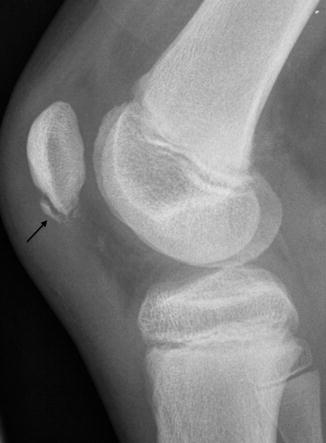
Fig. 17.7
Acute avulsion fracture of the inferior pole of the patella (arrow) at the origin of the patellar tendon
2 Imaging of Pediatric Fractures
Orthogonal radiographs are usually sufficient to diagnose pediatric fractures. Oblique views may provide further information about subtle fractures, particularly at the elbow, knee, and ankle. For example, internal oblique and external oblique views of the pediatric elbow are essential for accurate diagnosis of lateral condyle and medial condyle or epicondyle fractures. Tunnel views of the knee are important for imaging osteochondral fractures of the condyles, and mortise views of the ankle assist in characterizing the extent of ligamentous injury in ankle fractures. Axillary views of the shoulder are essential for evaluating glenohumeral dislocation.
Cross-sectional imaging is sometimes indicated to evaluate equivocal fractures and growth cartilage injuries or to distinguish trauma from other pathology such as infection or tumor. Ultrasound (US) is currently underutilized but can delineate cartilaginous structures and further assess osseous and physeal fractures. It is particularly helpful in evaluating radiographically occult epiphyseal avulsion fractures in infants. Sonographic findings include cortical discontinuity and growth plate widening; subperiosteal fluid or hematoma may be evident as well (Fig. 17.8) [8].
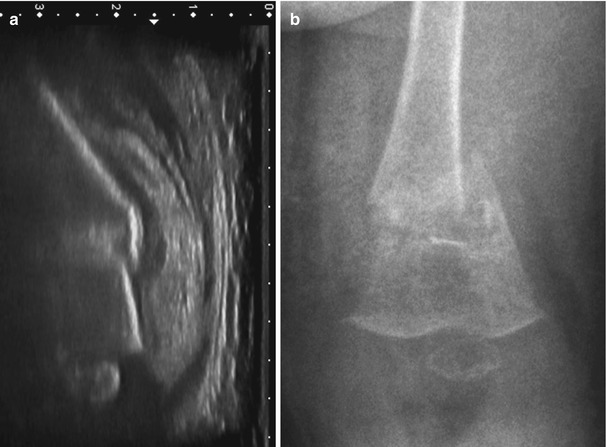
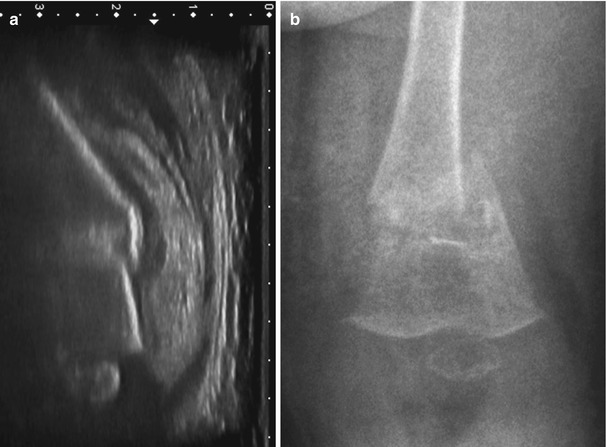
Fig. 17.8
Pathologic fracture in a 6-month-old boy with congenital renal insufficiency, who developed pain and swelling after routine vaccinations. (a) Ultrasound (US) shows a displaced fracture, with overlying hematoma (the US was performed to evaluate for deep venous thrombosis and has been rotated to correspond to the radiograph). (b) Osteopenia and displaced fracture (Courtesy of Willemijn M. Klein, MD, PhD, radiologist, Radboud University Medical Center, Nijmegen, the Netherlands)
The high-resolution cortical detail of computed tomography (CT) makes this modality excellent for delineating and confirming subtle fractures [6]. However, CT is most often used for presurgical assessment of complex intra-articular and growth plate fractures. CT can be performed in a cast with a single acquisition. The isotropic volume data of 64-detector technology can then be manipulated into multiplanar high-resolution two-dimensional (2D) and three-dimensional (3D) images (Fig. 17.9). Rapid acquisition speed nearly eliminates motion artifact, greatly decreasing the need for sedation [9].
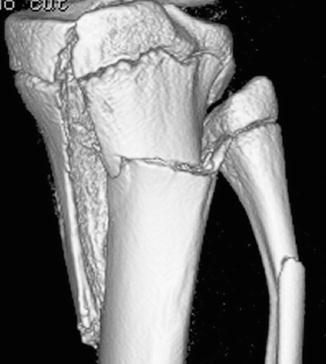
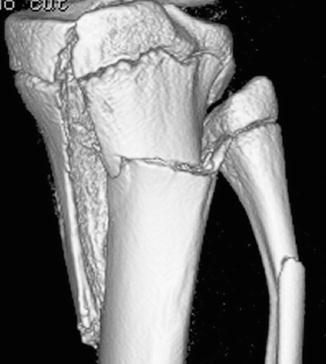
Fig. 17.9
Intra-articular Type IV proximal tibial fracture shown on reconstructed three-dimensional (3D) CT. There is also a fracture of the proximal fibular diaphysis
Magnetic resonance imaging (MRI) depicts occult osseous fractures, which are usually hypointense on T1-weighted (T1-W) images and hyperintense on fluid-sensitive sequences, including T2-weighted (T2-W) and short tau inversion recovery (STIR) (Fig. 17.10). MRI is particularly useful for assessing epiphyseal and physeal injuries, due to its multiplanar capability and superb soft tissue contrast [10]. MRI findings that suggest growth plate fracture include increased physeal thickness and T2 hyperintensity as well as disruption of the perichondrium. Gas within a physeal fracture cleft may be visible as a hypointensity, contrasted with the hyperintense physis on water-sensitive and multiplanar gradient echo (MPGR) images [6]. Fluid within a fracture cleft is hyperintense on water-sensitive sequences; it may be obscured, since the growth plate is also hyperintense. However, on gadolinium-enhanced 3D spoiled gradient-recalled echo (SPGR) images, growth plate fracture clefts that contain fluid are hypointense, compared to the enhancing physeal cartilage [11].
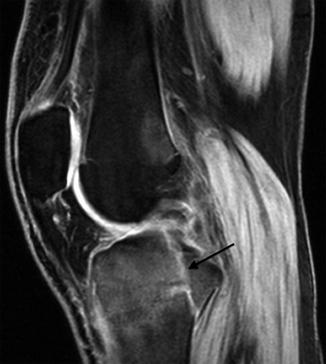
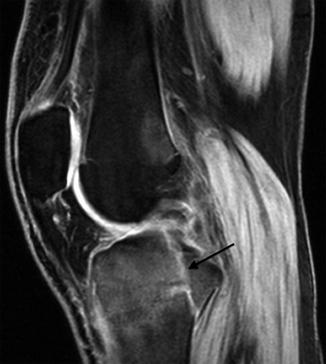
Fig. 17.10
Intra-articular proximal tibial fracture. Sagittal T2-weighted (T2-W) fat-suppressed (FS) image shows the vertical linear hyperintense fracture (arrow) with diffuse surrounding ill-defined hyperintense marrow edema (Courtesy of J. Herman Kan)
3 Normal Development and Imaging of Growth Cartilage
Knowledge of bone growth facilitates understanding and imaging interpretation of fractures and other osseous injuries. However, growth plate architecture and cartilage imaging are addressed only briefly here, as they are discussed in depth in Chap. 2.
Of the four distinct zones of the growth plate, the zone of provisional calcification (ZPC) is most vulnerable to trauma. This zone, adjacent to the metaphysis and the site of mineralization from cartilage to bone, is the weakest part of the growth plate. The thickness of the physis is relatively constant throughout growth due to a balance between the rate of cartilage production in the proliferative zone and cartilage removal and mineralization in the hypertrophic zone [12]. Although the physis is initially smooth and flat, differential growth rates lead to an undulating contour. Circumferential growth of the epiphyses, apophyses, and small bones proceeds by a similar mechanism. There is a fibrocartilaginous perichondrial ring, at the periphery of the physis, which provides structural support to the chondro-osseous junction [6, 13].
Unique characteristics of blood supply to the epiphysis and metaphysis affect the likelihood of complications after fracture or chronic repetitive cartilage injury. The epiphyseal cartilage is initially perfused by vessels within cartilage canals, which decrease in number and size during childhood. Cartilage canals also traverse the growth plate during infancy, but these disappear after about 18 months [14]. The physis thus has a dual vascular supply, with the epiphyseal artery supporting cartilage growth and the metaphyseal nutrient arteries supporting ossification (Fig. 17.11) [6]. The epiphyseal artery supplies the germinal and proliferative zones of the physis. The metaphyseal arteries supply the hypertrophic zone and zone of provisional calcification, but these nutrient arteries loop within the metaphysis before reaching the growth plate, and the hypertrophic zone is therefore relatively avascular. The tenuous nature of this blood supply makes the primary physis and epiphysis particularly prone to ischemic injury. Focal physeal ischemia may cause bone bridge formation, whereas epiphyseal ischemia may cause fragmentation and decreased size of the epiphyseal ossification center [13]. Fractures involving the germinal and proliferative zones have a higher incidence of complications than those involving the hypertrophic zone [10].
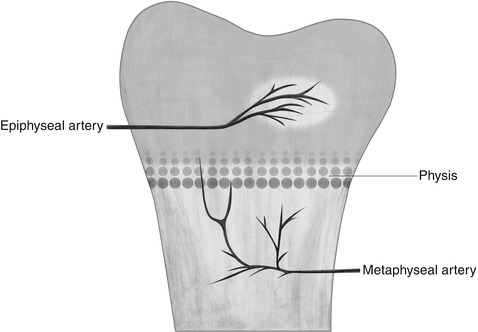
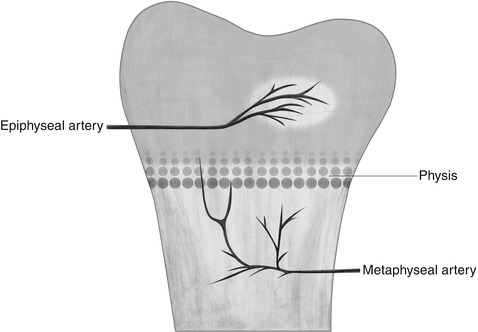
Fig. 17.11
After 18 months of age, the transphyseal vessels disappear, and the arterial supply of the metaphysis and epiphysis is separated by the growth plate
Several normal growth-related findings may mimic pathology. For example, although in infants and young children the growth plate is smooth and flat, uneven growth leads to physeal undulation. The thickness of the normal physis remains uniform. However, the wavy contour of the growth plate (Fig. 17.12) makes it difficult to obtain images perpendicular to the entire physis, and multiple planes and 3D sequences help evaluate this area [15]. As skeletal maturation approaches, the physis thins, and after it closes there is a residual very thin low signal physeal scar that could be misinterpreted as a fracture. This often persists into adulthood but eventually disappears [15]. The epiphyseal ossification center may develop from several ossification centers (Fig. 17.13) or may have an irregular contour in younger children (Fig. 17.14). However, the overlying cartilage is intact, which helps differentiate this appearance from pathology [16].
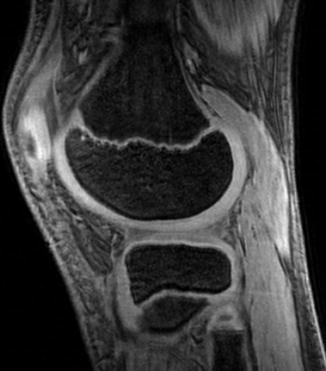
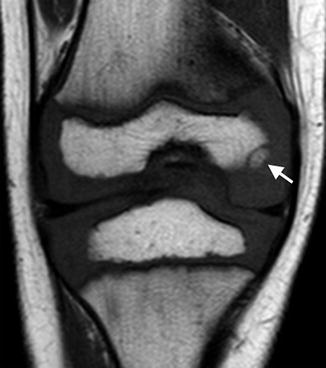
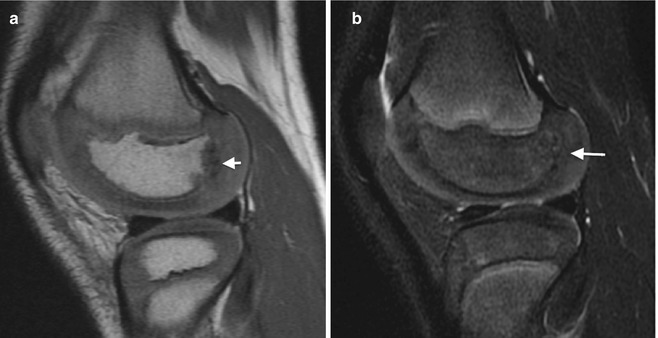
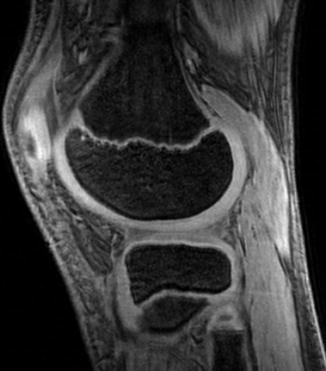
Fig. 17.12
Normal undulation of the distal femoral growth plate related to differential growth in a 12-year-old (sagittal fast spoiled gradient-recalled echo [FSPR])
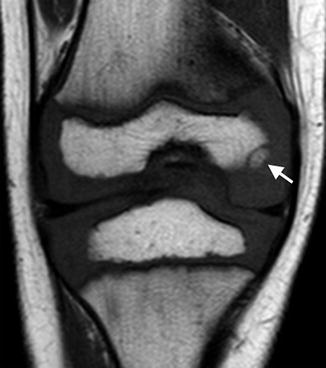
Fig. 17.13
Secondary ossification center (arrow) at distal femoral epiphysis has hyperintense signal due to marrow fat in this young child (coronal T1-weighted (T1-W) image)
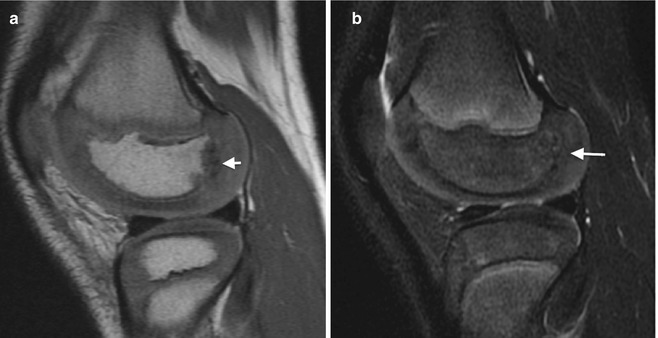
Fig. 17.14
Normal cortical irregularity in a 7-year-old. Sagittal proton density (PD) (a) and T2-W FS (b) images show irregularity of the posterior aspect of the lateral femoral condylar ossification center without edema or cartilage defect (arrows)
4 Classification of Growth Plate Injury
Several classification schemes have been developed since Poland’s systematic description of epiphyseal injuries in 1898. Salter and Harris (1963) devised the simplest and most widely used classification system, which describes five types of growth plate injury, according to radiographic findings in the epiphysis, physis, and metaphysis (Table 17.1) [17]. Ogden subsequently extended this system to include nine fracture types that affect bone growth at any site, including appositional growth of the diaphysis (Fig. 17.15) [18]. However, with the exception of Type VII, isolated epiphyseal fractures, the additional types only account for a small percentage of injuries [6].
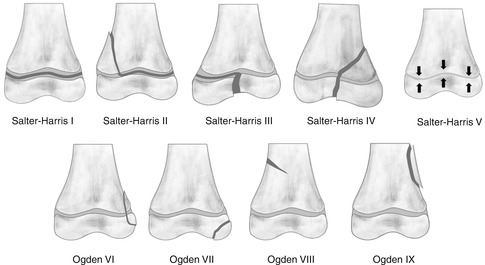
Table 17.1
Salter-Harris growth plate fractures
Incidence | Age/common location | Radiographic findings | Cross-sectional imaging |
---|---|---|---|
Type I | Usually <5 y/o | Soft tissue swelling | MRI: physeal widening |
6 % | Proximal/distal humerus | Fracture often unapparent | T2 hyperintense |
Proximal femur | Growth plate widening | ||
Older child | Epiphyseal displacement | ||
Distal radius | Blurring of distal metaphysis | ||
Proximal humerus | Tiny fragments within physis | ||
Proximal femur | |||
Type II 75 % | Usually >10 y/o Distal radius | Triangular metaphyseal fragment adjacent to physis | Infrequently performed Exceptions |
Distal tibia | May extend into diaphysis | Distal femur | |
Distal fibula | Growth plate widening | Proximal tibia | |
Phalanges | Epiphyseal displacement | ||
Type III 8 % | Usually adolescent Distal tibia | Intra-articular sagittal or oblique fracture through epiphysis | CT or MRI to assess alignment Low T1, low or high T2 fracture line through epiphysis Wide high T2 physis |
Proximal tibia | Growth plate widening | ||
Distal femur | |||
Phalanges | |||
Type IV | 5–10 y/o | Intra-articular fracture | CT or MRI to assess alignment |
10 % | Distal humerus (lateral condyle) | Through physis, epiphysis, and metaphysis | Low T1, low or high T2 fracture line through epiphysis and metaphysis |
Older child | Wide high T2 physis | ||
Distal radius | |||
Adolescent | |||
Distal tibia | |||
Type V | Severe trauma | May see physeal narrowing | MRI may show hemorrhage or hematoma within growth plate immediately after injury |
1 % | Usually diagnosed retrospectively | ||
Present with complications of growth arrest |
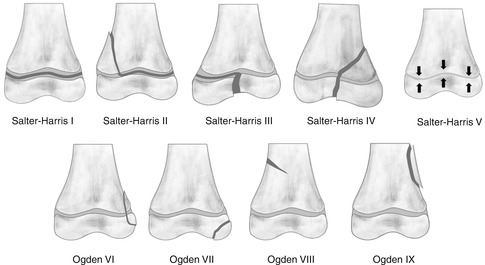
Fig. 17.15
The Salter-Harris I–V and extended Ogden VI–IX classification of fractures at or near the growth plate
4.1 Salter-Harris Fractures
Type I Fractures
These fractures are confined to the physis and usually result from a shearing or avulsion force that parallels the growth plate. Type I fractures occur most often in children less than 5 years old but are relatively uncommon, representing around 6 % of all growth plate injuries. In infancy, they usually occur in the proximal humerus, distal humerus, and proximal femur, resulting from birth trauma or non-accidental trauma [6]. In older children, Type I fractures are most common in the distal radius, proximal humerus, and proximal femur. “Little Leaguer’s shoulder,” a Type I fracture of the proximal humerus, occurs around age 10–12 in throwing athletes. Slipped capital femoral epiphysis (SCFE), also a Type I fracture, occurs around puberty, usually without specific antecedent trauma. Most Type I injuries have an excellent prognosis [6], but SCFE may be complicated by avascular necrosis of the femoral head, since the artery supplying the epiphysis courses along the physis and may be injured at epiphyseal separation.
The classic but relatively uncommon radiographic finding is displacement of the epiphysis without an osseous fracture. This may be seen in infants with traumatic separation of the distal humerus, which usually results in posteromedial displacement of the distal fragment [19] (Fig. 17.16) (see Chap. 8). However, in many cases the fracture reduces spontaneously after the initial slip, and radiological diagnosis may be difficult. Findings that suggest a Type I fracture include slight widening of the growth plate or poor definition of the metaphyseal margin adjacent to the growth plate, due to slight angulation. Very small, thin bone fragments may be seen along the physis, due to fracture propagation from the hypertrophic zone into the zone of provisional calcification [6] (Fig. 17.17). In infants whose epiphysis has not yet ossified, a slipped epiphysis may mimic a dislocation. Ultrasound may confirm a Type I fracture by showing malalignment between the metaphysis and cartilaginous epiphysis (Fig. 17.18). MRI may be used to assess for occult Type I injury, particularly in regions where growth plate damage could cause significant growth arrest, such as the knee. Water-sensitive sequences typically show a wide, hyperintense physis, with adjacent marrow and soft tissue edema (Fig. 17.19). Follow-up radiographs in 7–10 days may show periosteal new bone formation in the adjacent metaphysis, due to healing.
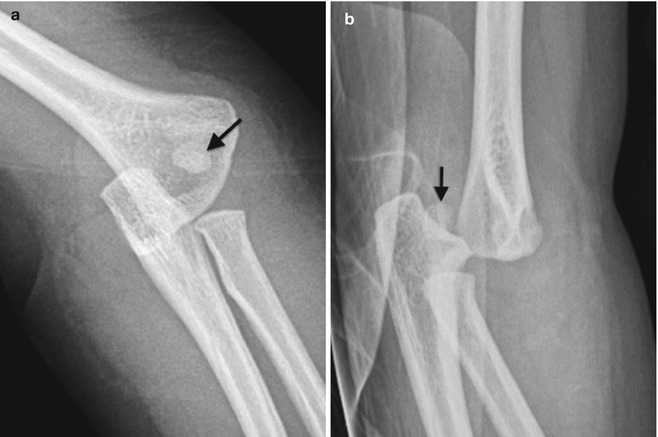
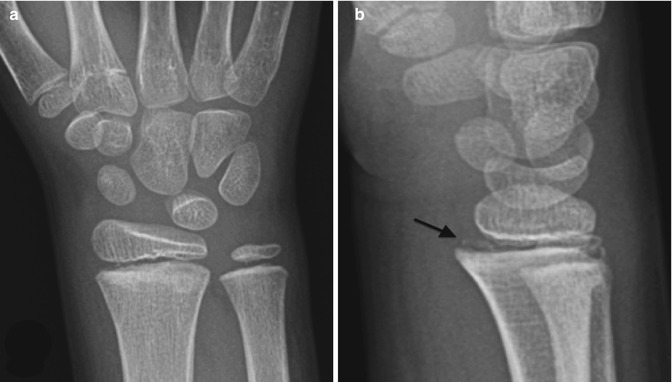
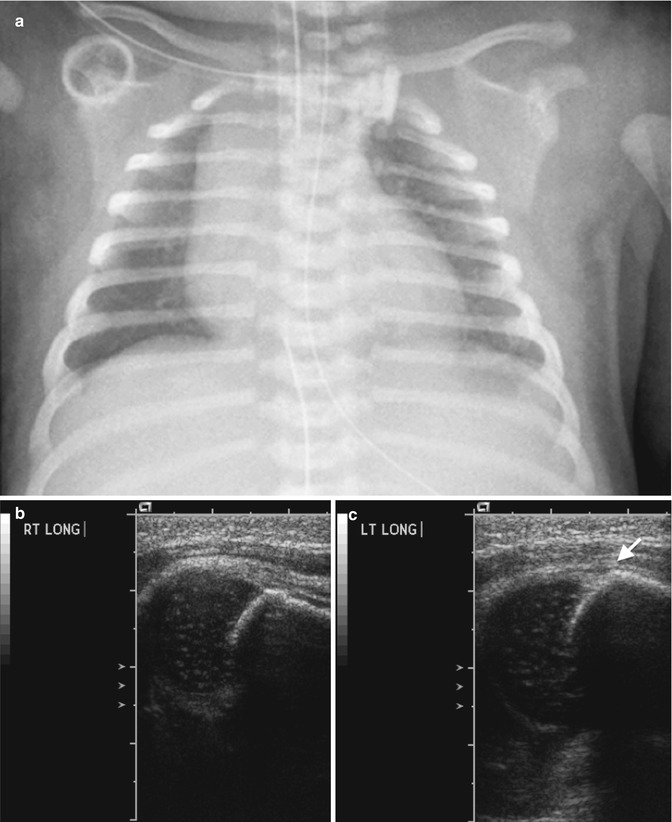
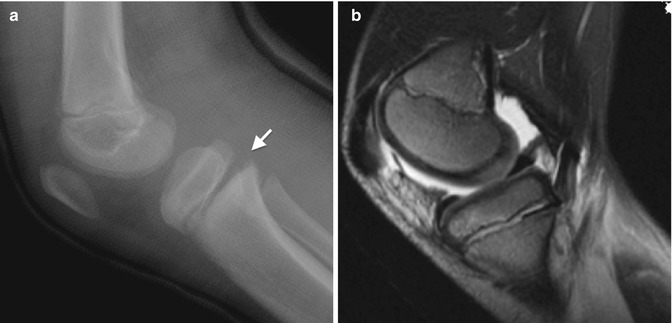
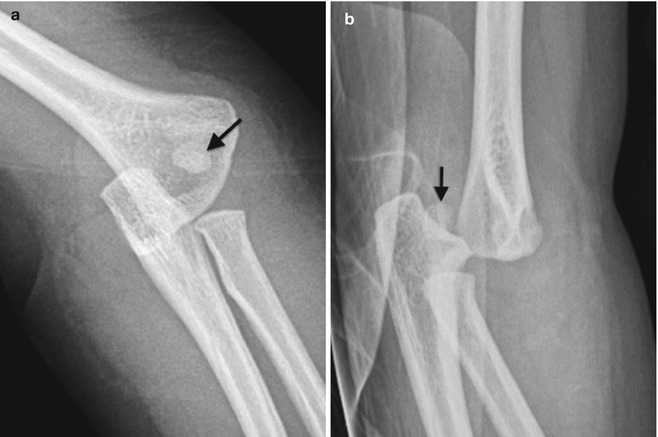
Fig. 17.16
Type I distal humeral fracture in a young child. (a, b) The capitellum (arrow) is displaced posteromedially with respect to the distal humeral metaphysis but maintains alignment with the radius and ulna
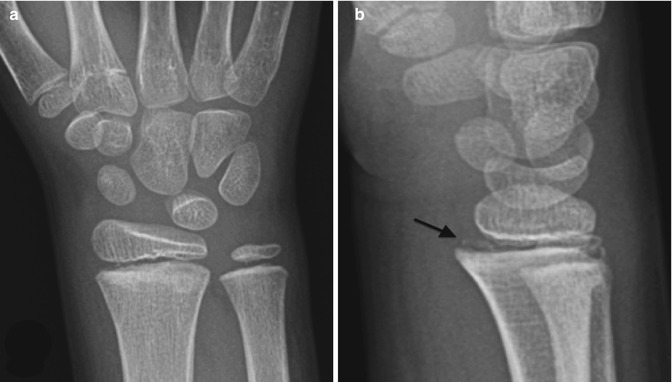
Fig. 17.17
Type I fracture with mild posterior displacement of the distal radial epiphysis. (a, b) Note tiny fragments along the physis (arrow)
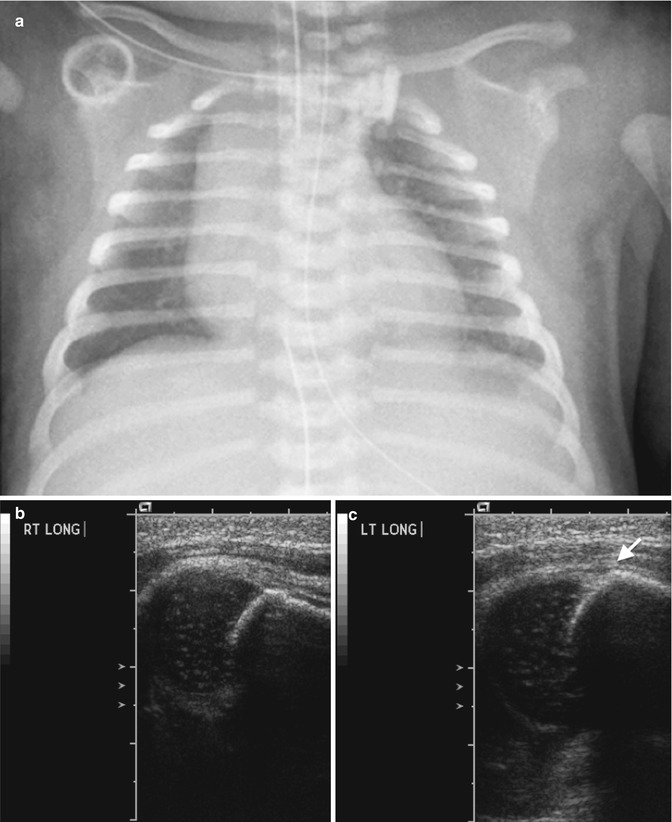
Fig. 17.18
Type I fracture in a newborn who did not move the left arm after a difficult delivery. (a) The distance between the left glenoid and proximal humeral metaphysis may be slightly wide, perhaps suggesting dislocation or Type I fracture. (b) Sagittal US on right side (for comparison) shows normal alignment. (c) Sagittal US on left shows malalignment of the hypoechoic cartilaginous epiphysis and echogenic cortical bone
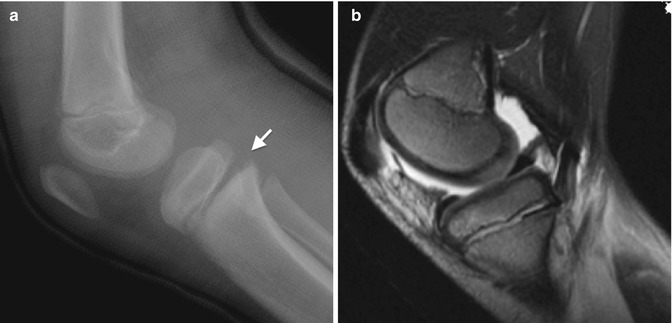
Fig. 17.19
Type I fracture of proximal tibial physis. (a) Widening of the posterior portion of proximal tibial physis (arrow). (b) Sagittal T2-W FS image shows increased signal and widening of the proximal tibial physis with adjacent marrow edema and a joint effusion
Type II Fractures
Type II fractures extend along the growth plate into the metaphysis and usually result from a shearing or avulsive force. They are the most common physeal injury, representing 75 % of growth plate fractures. Type II injuries are rare in infancy and usually occur in children older than age 10. The distal radius is most often affected, accounting for up to 50 % of Type II fractures [6]. Other common sites include the distal tibia, distal fibula, and phalanges. Growth usually continues undisturbed or with minimal shortening, as the germinal matrix is often preserved. However, Type II fractures at the knee, especially at the distal femur, have a guarded prognosis, as the force required to produce these injuries may crush the germinal cells [6]. Distal femoral growth plate fractures are usually caused by severe force, such as high-speed motor vehicle collisions, but these fractures also have an increased incidence in adolescents playing contact sports.
Imaging
The classic radiographic finding of a Type II fracture is a triangular metaphyseal fragment adjacent to the growth plate (the Thurston-Holland sign) (Fig. 17.20). Variations include a metaphyseal fracture parallel to the growth plate or extending into the diaphysis. The metaphyseal fragment is usually visible on radiographs, and there is often epiphyseal displacement and growth plate widening (Fig. 17.21). The periosteum is intact along the metaphyseal fracture but torn at the site of growth plate separation.
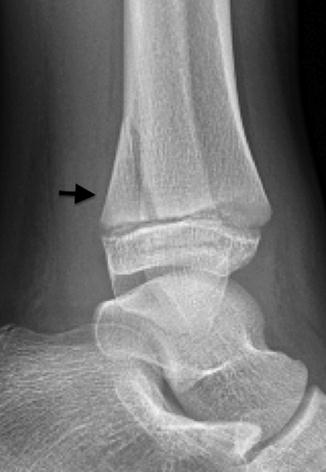
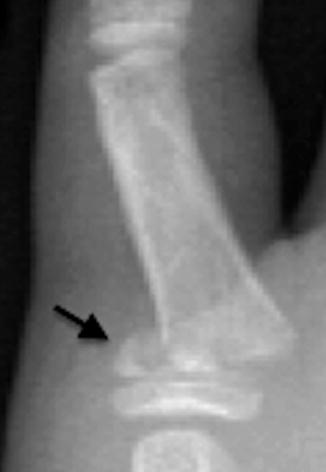
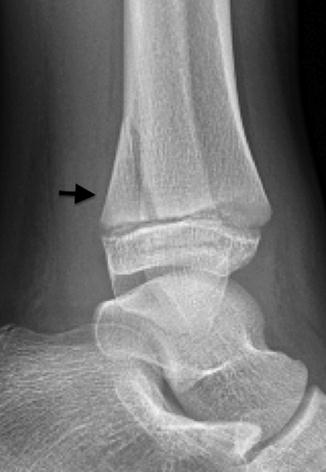
Fig. 17.20
Type II fracture of the distal tibia. Growth plate widening and a posterior triangular metaphyseal fragment (arrow)
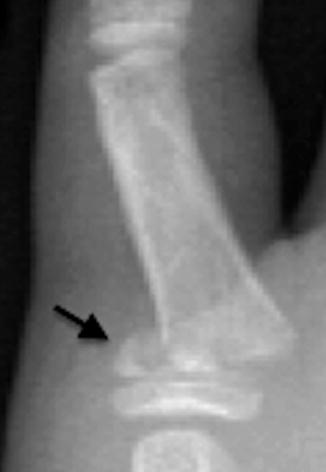
Fig. 17.21
Type II fracture with small metaphyseal fragment (arrow)
Type III Fractures
Type III fractures extend both vertically and obliquely through the epiphysis to the articular surface, and horizontally through the physis, resulting in separation of a portion of the epiphysis. They represent 8 % of growth plate fractures and most commonly occur during adolescence. These fractures usually result from vertical shearing force, and fragments are typically at most minimally displaced [6]. The prognosis is generally good, provided the fracture is not significantly displaced and the epiphyseal blood supply is intact. However, if a displaced fracture is incompletely reduced, bone bridging and/or articular incongruity may result. The most common locations for these fractures are the distal tibia, proximal tibia, distal femur, and phalanges [6].
Imaging
Type III fractures are usually visible on radiographs (Figs. 17.22 and 17.23), but additional oblique views may be needed for diagnosis. The juvenile Tillaux fracture, which affects the distal tibial epiphysis, occurs when physeal fusion is incomplete (see Chap. 16). Forced supination and external rotation of the ankle cause stress on the anterior tibiofibular ligament, with resultant avulsion of a small fragment of the bone from the anterolateral corner of the distal tibial epiphysis (Figs. 17.24 and 17.25). Type III fractures of the distal femur usually result from severe valgus stress, sometimes due to direct force against the lateral portion of the knee (as in a football “clip” or wrestling). CT or MRI may help delineate these fractures and assess alignment, particularly at the distal femur, proximal tibia, and distal tibia (Fig. 17.26).
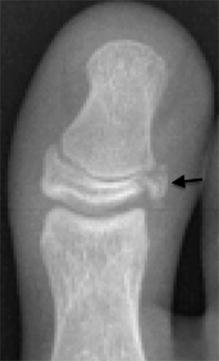
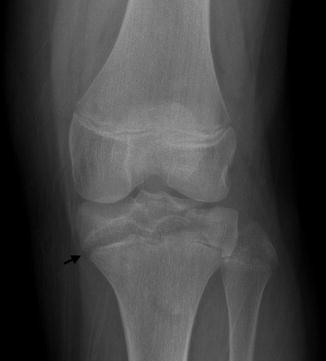
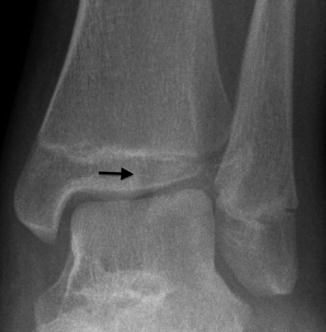
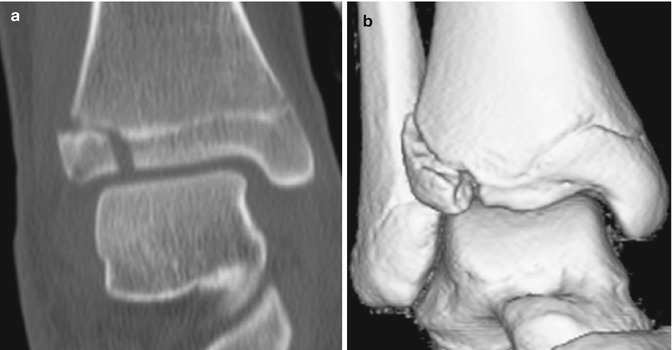
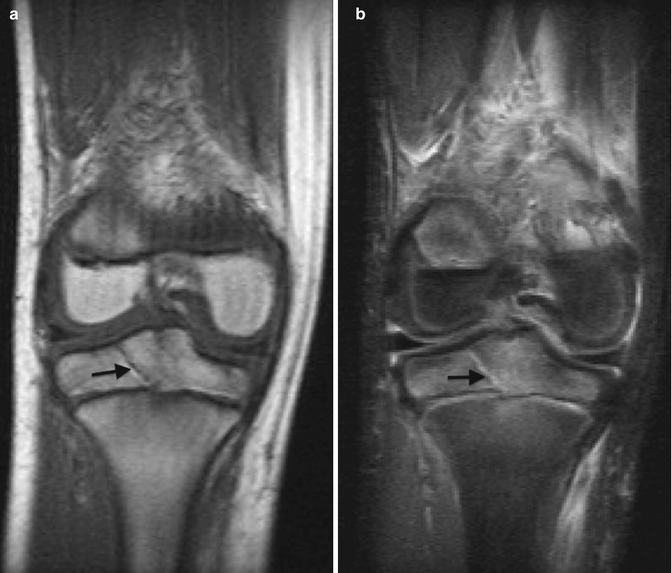
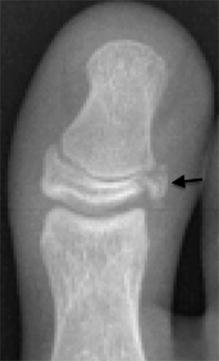
Fig. 17.22
Type III fracture. The epiphyseal fragment is rotated (arrow)
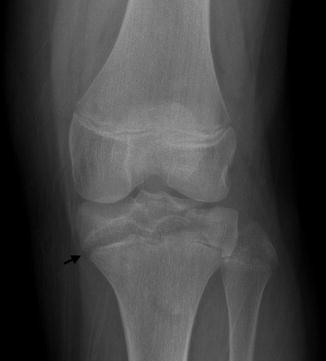
Fig. 17.23
Type III fracture of the proximal tibia. An oblique fracture line traverses the epiphysis, and the medial physis is widened (arrow)
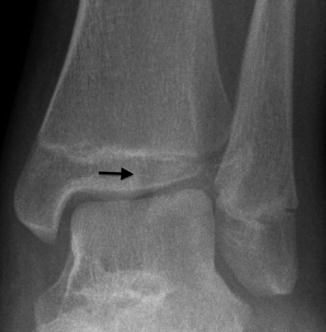
Fig. 17.24
Type II fracture (Tillaux) in a 13-year-old who fell while Rollerblading. Vertical fracture (arrow) through epiphysis
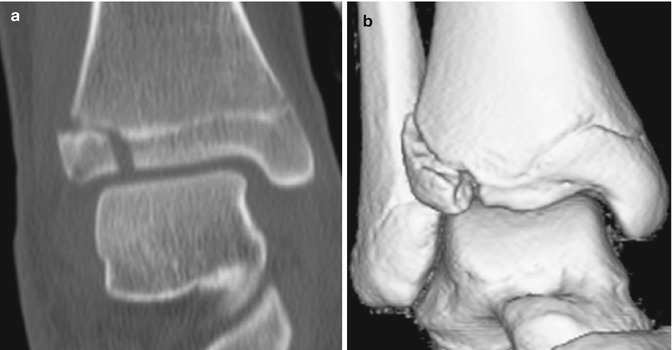
Fig. 17.25
Type III fracture (Tillaux). Coronal two-dimensional (2D) (a) and three-dimensional (3D) (b) computed tomography (CT) reformatted images
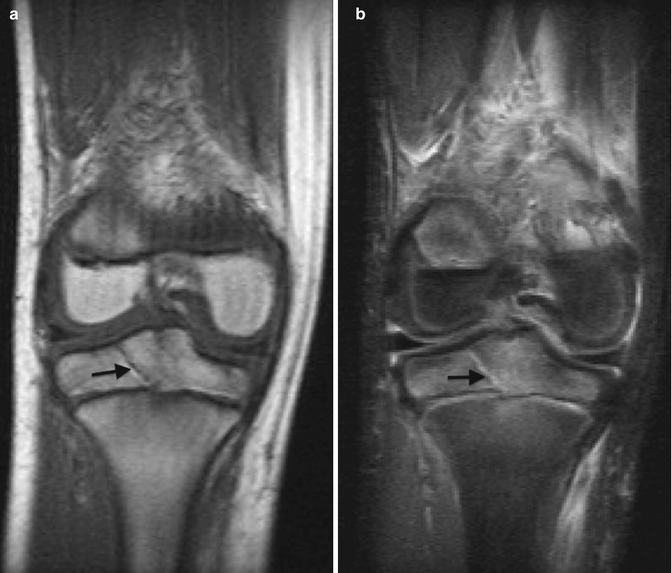
Fig. 17.26
Type III fracture of the proximal tibial epiphysis. Coronal T1-W (a) and T2-W FS (b) images show an oblique non-displaced fracture line extending to the growth plate, which is T1 hypointense and T2 hyperintense (arrows)
Type IV Fractures
These fractures usually result from a vertical compression or shearing force that extends through the metaphysis, epiphysis, and intervening physis. They represent 10 % of growth plate fractures and most commonly occur at the distal humerus (lateral condyle), distal tibia, and distal radius [20]. Because these fractures are intra-articular, they must be reduced to near-anatomic alignment to prevent long-term complications such as joint deformity, accelerated osteoarthritis, or bone bridge formation. Such complications are most likely at the elbow, knee, and ankle.
Imaging
Radiologic findings of Type IV fractures include a fracture line extending through the epiphysis, growth plate, and metaphysis (Fig. 17.27). Lateral condylar fractures of the distal humerus, which represent about 20 % of pediatric elbow fractures and which usually occur in children age 4–10 years, are often Type IV fractures (Figs. 17.28 and 17.29) [21] (see Chap. 8). Internal oblique views may demonstrate a subtle, minimally displaced lateral condylar fracture [22]. CT more accurately evaluates the degree of fracture displacement and can determine if the articular cartilage is intact [23, 24]. However, MRI, with its superior cartilage detail, is the best method for distinguishing between Type II and IV lateral condylar fractures (Fig. 17.30). Lateral condylar fractures have the highest incidence of nonunion of all pediatric fractures [22].
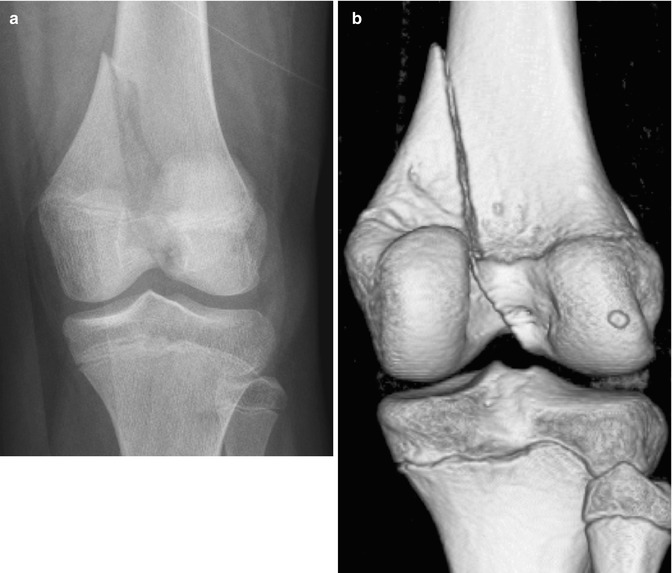
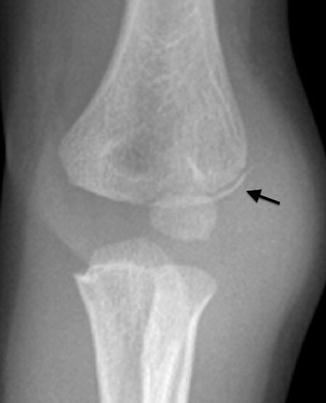
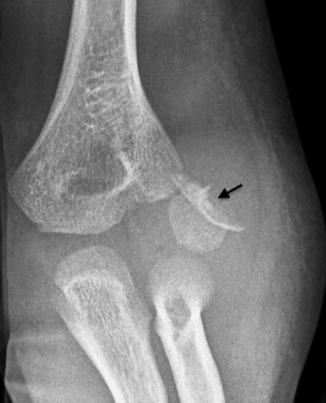
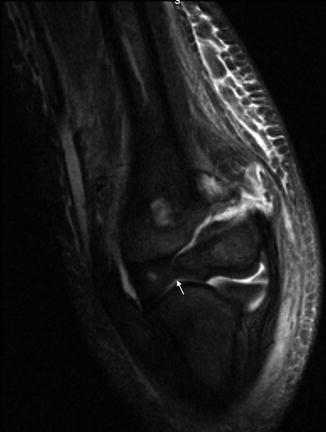
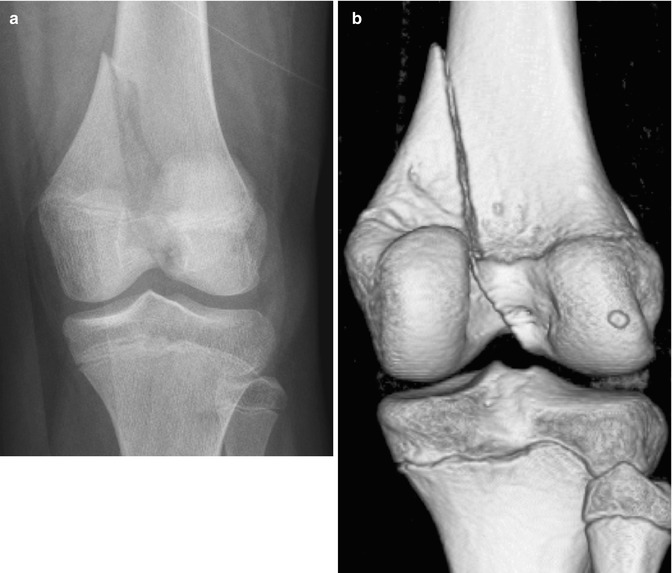
Fig. 17.27
Type IV fracture of the distal femur. (a) There is a vertical fracture of the distal femur, extending from the metadiaphysis, across the physis, and through the intercondylar region of the epiphysis. (b) 3D CT reconstruction
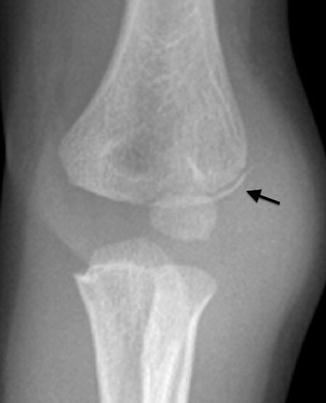
Fig. 17.28
Lateral condylar fracture. There is a thin, non-displaced metaphyseal fragment (arrow). Distinction between a Type II and IV fracture cannot be made radiographically as the epiphysis is largely cartilaginous
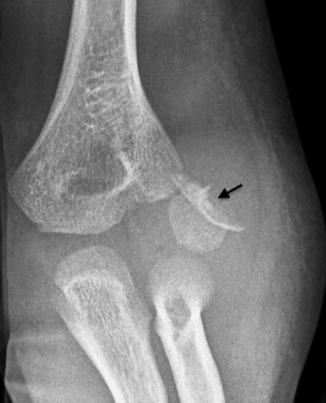
Fig. 17.29
Type IV lateral condylar fracture in a 5-year-old who fell from a play structure. There is striking displacement and rotation of the capitellum and metaphyseal fragment (arrow)
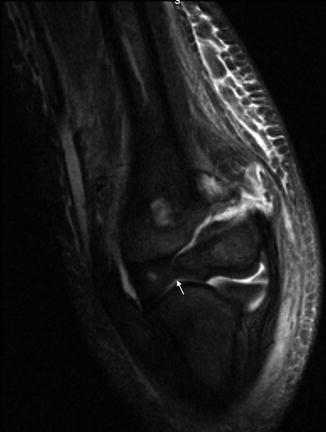
Fig. 17.30
Type IV fracture. Coronal T2-W FS image shows high T2 signal in the fracture, extending from the lateral metaphysis into the cartilaginous epiphysis, with an intact cartilage flap (arrow) (Courtesy of J. Herman Kan)
The triplane fracture is a Type IV injury of the distal tibia, occurring at the end of puberty, as the physis is fusing (see Chap. 16). Usually a result of eversion injury, it may result from a fall [25]. This fracture consists of a coronal fracture through the posterior metaphysis, a transverse fracture along the lateral physis and a sagittal fracture through the anterior epiphysis. This complex pattern is related to asymmetric fusion of the distal tibial growth plate, which begins medially at a physeal undulation, “Kump’s bump” [26]. Radiographs typically show widening of the lateral physis, with the epiphyseal fracture evident on the frontal or oblique view and the metaphyseal fracture evident on the lateral view (Fig. 17.31). CT with multiplanar reformats is indicated for presurgical assessment (Fig. 17.32). Since these fractures occur near skeletal maturity, limb shortening is uncommon; the prognosis is largely determined by the integrity of the tibial plafond [12, 20].
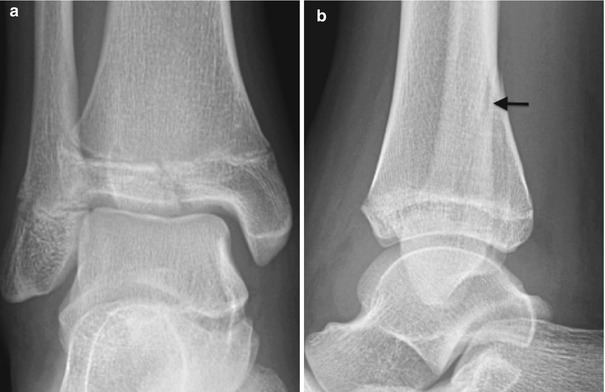
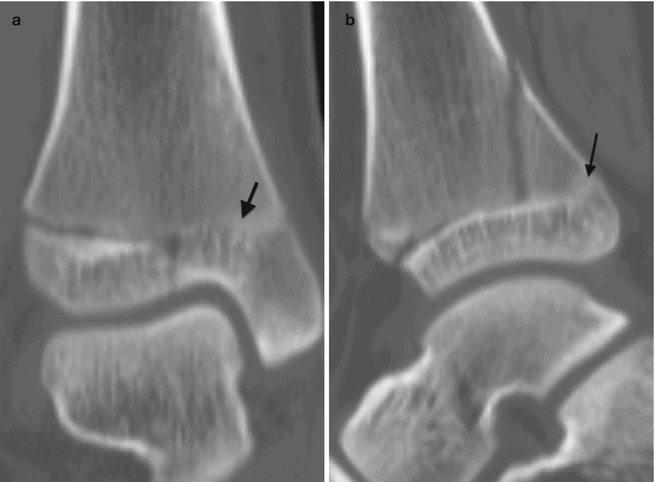
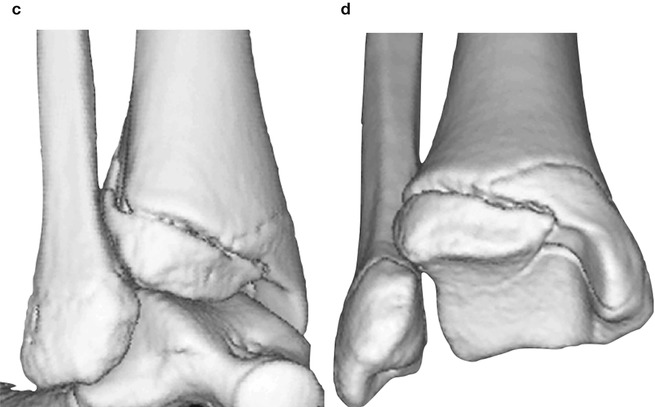
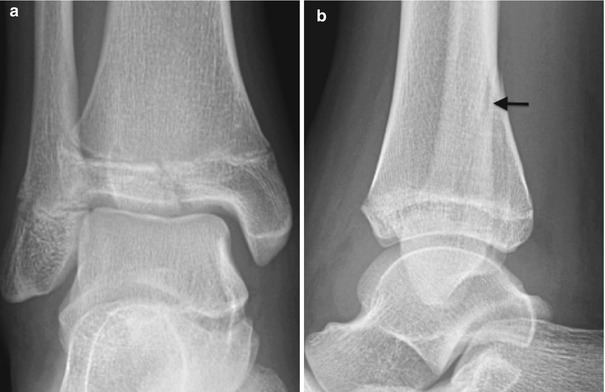
Fig. 17.31
Type IV triplane fracture. (a) Vertical fracture through the distal tibial epiphysis with widening of the lateral aspect of the growth plate. (b) Oblique fracture component through the posterior aspect of the distal tibial metaphysis (arrow)
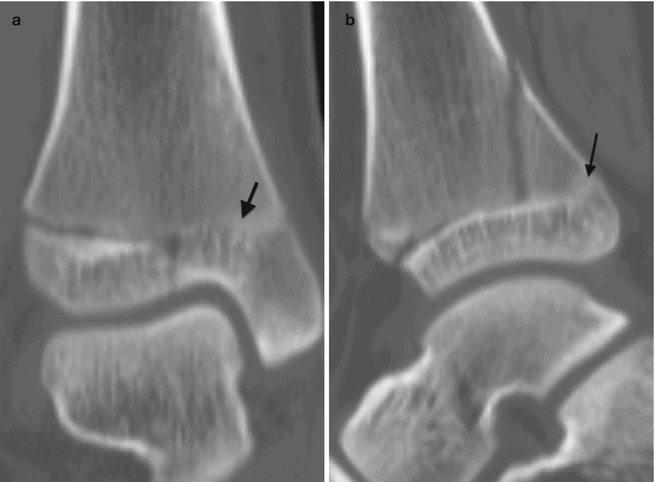
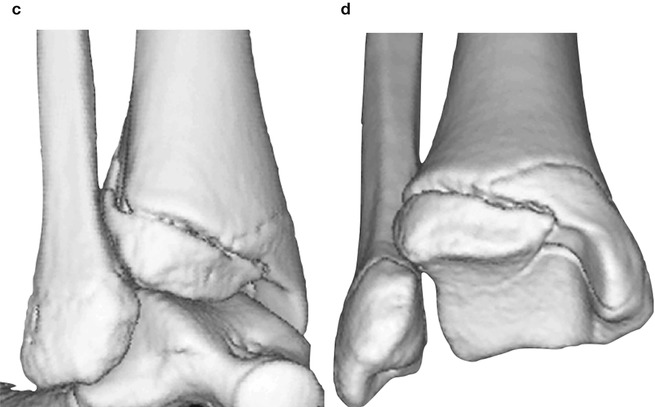
Fig. 17.32
Type IV triplane fracture. Coronal (a) and sagittal (b) CT reformats show partial fusion at the medial and posterior distal tibial growth plate (arrows). 3D CT reformats (c, d) show all three fracture planes
Type V Fractures
These injuries represent only 1 % of growth plate fractures and result from severe axial loading with physeal compression or crush injury. This damages the vascular supply and germinal cells of the physis, and subsequent growth arrest is therefore common and the prognosis poor [6]. Type V fractures are most common in the distal femur, proximal tibia, and distal tibia and may also coexist with other types of complex growth plate injuries [12]. Radiographic diagnosis is difficult and often made retrospectively, when premature physeal fusion is observed. Type V injuries may be suspected after significant axial loading injury of the knee or ankle, especially if associated with adjacent fractures. MRI can establish the diagnosis immediately after injury, if hemorrhage or hematoma is identified within the growth plate [6].
4.2 Ogden Extension of Salter-Harris Fractures
Type VI Fractures
This injury is very rare and damages the perichondrial ring. It frequently involves a lawn mower or farm machinery and is often associated with a compound or open fracture. With healing, a bone bridge forms, connecting the epiphysis to the metaphysis and leading to angular deformity. The distal femur and distal tibia are common locations. Radiographs may demonstrate a small, sliver-like fragment at the lateral margin of the epiphysis [6].
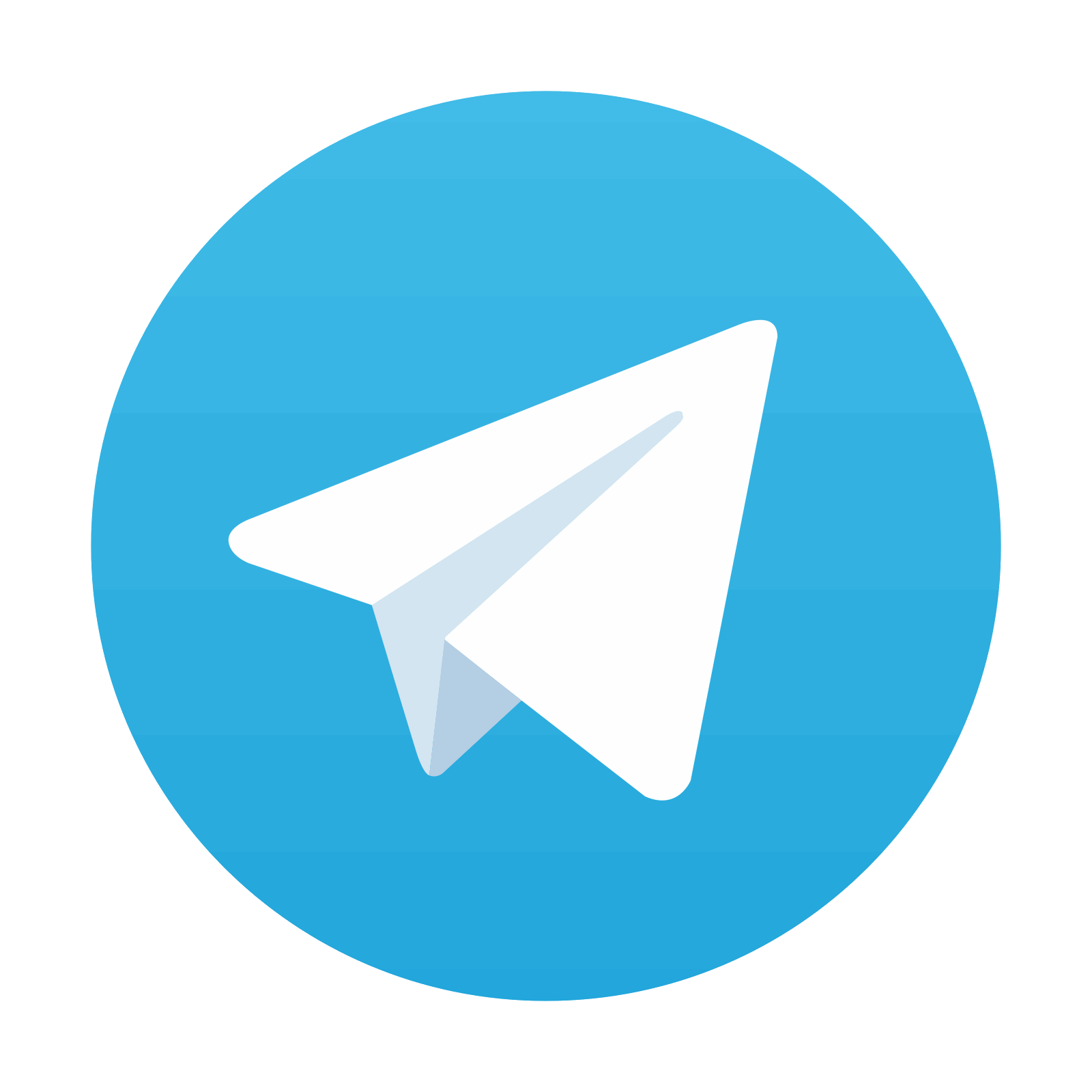
Stay updated, free articles. Join our Telegram channel

Full access? Get Clinical Tree
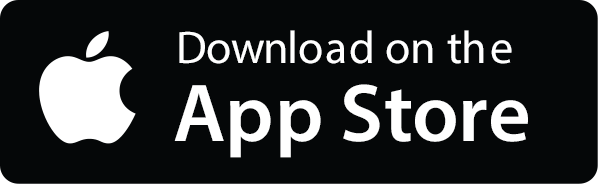
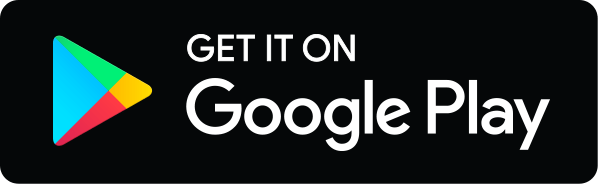