Abstract
The skull base is made of flat bones that separate the intracranial compartment from the extracranial head and neck. Owing to this unique location, it can be affected both by intrinsic lesions originating from the bony elements of the skull base and by lesions originating outside the skull base proper or from trapped embryonic remnants. This chapter specifically focuses on the imaging techniques, developmental anomalies, skull base lesions originating from embryonic remnants, skull base infection, and other diffuse skull base lesions. Diagnostic imaging may require the use of different techniques, most often CT, MRI, bone scintigraphy, and PET-CT and is crucial in patient’s management, as the skull base is hidden from clinical inspection. Awareness of anatomic variants is crucial in surgical planning and to avoid potential confusion with skull base lesions. Moreover, radiologists should also be familiar with embryonic abnormalities affecting the skull base. Often, their particular location and other associated imaging features can be diagnostic. Proximity with sinus cavities (sinonasal and otomastoid) and with the nasopharynx makes the skull base prone to spread of infectious processes when appropriate treatment is delayed. Skull base osteomyelitis from bacterial or fungal agents remains a diagnostic challenge, particularly in the absence of relevant clinical data, and requires a high degree of suspicion. Diffuse bone lesions affecting the skull base result from defects of endochondral ossification, which may also affect the remaining skeleton. Overall, diagnostic imaging of these conditions is similar to that of bone lesions outside the skull base and the same rules for differential diagnosis apply.
Keywords
developmental skull base anomalies, embryonic skull base lesions, endochondral ossification defects, imaging bone lesions, skull base osteomyelitis, skull base variants
Introduction
The skull base is a bony-cartilaginous structure, oriented in the axial plane, providing a barrier between the intracranial compartment and the extracranial head and neck. It is pierced by several neurovascular foramina, which provide a crossroad for disease to spread between these two compartments. Most tumors affecting the skull base have their origin outside the skull base proper, mainly from the suprahyoid neck, and secondarily affect the skull base by direct or perineural extent. Primary and secondary bone tumors tend to be forgotten. The skull base division into anterior, central, and posterior, so useful in the differential diagnosis of extrinsic skull base lesions, does not apply when lesions originate from the fibrocartilaginous elements of the skull base proper as they are common to all skull base compartments. Therefore, when facing a lesion arising from bone the same rules for the differential diagnosis of bone lesions seen elsewhere in the body should be applied.
Except for metastases, bone tumors of the skull base are overall rare and often a diagnostic dilemma. Benign tumors and bone dysplasias can behave aggressively, and some malignant tumors can look misleadingly benign. Although imaging features can be quite helpful in the differential diagnosis, they are not very familiar to radiologists who do not routinely deal with skull base lesions and only a scant specific literature is available on this subject. Moreover, secondary/metastatic tumors account for the majority of bony skull base lesions, with primary skull base bone tumors being rather rare. To simplify the approach, it is useful to divide bone lesions of the skull base into three major groups: those related to developmental anomalies and embryonic remnants, primary and secondary bone tumors, and diffuse or multifocal bone lesions ( Table 15.1 ).
Developmental/Embryonic Remnants | |
Developmental | Arrested pneumatization of the sphenoid sinus |
Giant arachnoid granulations/arachnoidoceles | |
Sphenoid wing dysplasia | |
Petrous apex cephaloceles | |
Basal cephaloceles | |
Embryonic remnants | |
Ectodermal | Epidermoid/dermoid cysts |
Meningothelial | Arachnoid granulations |
Intradiploic arachnoid cysts | |
Intradiploic/en plaque meningiomas | |
Notochord | Ecchordosis physalifora |
Chordoma | |
Pharyngohypophyseal canal | Persistent craniopharyngeal canal |
Rhatke pouch cyst | |
Craniopharyngioma | |
Intraosseous pituitary adenoma | |
Bone Tumors | |
Primary | |
Benign | Osteoma |
Osteoid osteoma | |
Osteoblastoma | |
Ossifying fibroma | |
Enchondroma/osteochondroma | |
Chondroblastoma | |
Chondromyxoid fibroma | |
Intraosseous hemangioma | |
Aneurysmal bone cyst | |
Giant cell tumor/osteoclastoma | |
Brown tumor | |
Eosinophilic granuloma | |
Malignant | Metastasis |
Plasmacytoma/multiple myeloma | |
Lymphoma | |
Chloroma/granulocytic sarcoma | |
Ewing sarcoma | |
Osteosarcoma | |
Chondrosarcoma | |
Secondary | |
Metastasis | |
Lymphoma | |
Leukemia (chloroma) | |
Diffuse Bone Lesions | |
Fibrous dysplasia | |
Paget disease | |
Osteopetrosis | |
Osteopoikilosis | |
Melorheostosis | |
Infection (skull base osteomyelitis) | |
Multifocal Bone Lesions | |
Metastases | |
Multiple myeloma | |
Brown tumors | |
Eosinophilic granulomas |
This chapter reviews the imaging technique and focuses on developmental lesions originating from embryonic remnants trapped within the skull base during embryonic development and diffuse bone lesions, including bone dysplasias and infection; the following chapter addresses primary and secondary bone tumors.
Imaging Technique
Imaging of bone lesions in the skull base obeys the same principles applied to bone lesions elsewhere. To the exception of plain radiographs, which, in most centers, are no longer performed to investigate the skull base, and ultrasound imaging, with obvious limitations in the assessment of this anatomic region, the same imaging techniques (CT, MRI, bone scintigraphy, and fludeoxyglucose [FDG]-PET CT) are routinely used for diagnosing, staging, and follow-up of patients with skull base bone lesions. Volumetric CT acquisition parallel to the skull base with axial, coronal, and sagittal thin, millimetric, reconstructions on both soft tissue and high-resolution bone algorithm should be obtained. Contrast-enhanced imaging is performed to assess the pattern of vascularization of a given lesion, as well as to determine its relationship with major adjacent vessels and assess their patency. It provides a map of the bony anatomy, required for surgical planning, and allows three-dimensional (3D) reconstructions to help planning skull base reconstruction or grafting on the basis of 3D modeling and 3D printing. CT is the imaging technique with the highest sensitivity to depict calcification and ossification, critical in the assessment of bone or cartilage matrix-producing tumors and in depicting different types of periosteal reaction. The pattern of bone involvement and the evaluation of the transition zone, between tumor and normal bone, are also best depicted on CT. Still, for some authors, CT remains the most accurate technique in the diagnosis of fibroosseous lesions, which may show misleading imaging findings on other techniques, such as MR and bone scintigraphy. Moreover, as most bone lesions heal by ossification and sclerosis, CT is very useful to assess tumor response to treatment.
MRI is the modality of choice to evaluate the full extent of a bone tumor, including the extent of bone marrow involvement, presence of soft tissue components, meningeal and intracranial extent, involvement of cranial nerves and vessels, and relationship with adjacent soft tissues of the supra-hyoid neck. T1-weighted (T1W) images show clearly abnormal replacement of the fatty bone marrow, whereas T2-weighted (T2W) and short-tau inversion recovery (STIR) images clearly depict tumor matrix and any associated bone marrow edema. Contrast-enhanced fat-suppressed T1W images evaluate tumor enhancement and can clearly differentiate enhancing lesions from the surrounding fatty marrow. MR is also used to assess tumor response to chemo and radiation treatment by differentiating necrosis from viable tumor. This information can be derived from contrast-enhanced fat-suppressed T1W images, diffusion-weighted images (DWI), and dynamic contrast-enhanced perfusion-weighted images (DCE-PWI). DWI is very useful to support the diagnosis of small round cell tumors that tend to show low mean apparent diffusion coefficient (ADC) values and to differentiate bone malignancies from infection. Moreover, in the case of skull base osteomyelitis, it is routinely used to depict complications such as abscesses or subdural empyema, also featured by restricted diffusion. Whole-body DWI can depict diffuse and multifocal abnormalities and is being increasingly used for staging multiple myeloma and metastatic bone disease. MR angiography (MRA) and MR venography (MRV) may be required to assess the patency of any adjacent arteries and rule out dural sinus invasion or thrombosis, respectively. Bone scintigraphy using 99m Tc-MDP (methylene diphosphonate) and 18 F-NaF PET-CT are very sensitive techniques in the detection of osteoblastic activity, whereas 18 FDG-PET depicts tissues with high glucose metabolic rates. These functional techniques have the advantage of imaging the entire skeleton and therefore are useful to detect diffuse and multiple lesions. 67 Ga-single-photon emission CT (SPECT) and 99m Tc MDP-triphasic SPECT should be the choice for the early diagnosis and to monitor treatment response in patients with skull base osteomyelitis.
Bone scans can be used to depict bone-forming tumors, including osteogenic primary tumors and sclerotic metastasis, as well as any bone healing process. Tumors causing rapid bone destruction with no compensatory bone formation are missed by this technique, with the most striking examples being multiple myeloma and bone metastases from thyroid cancer. The flare phenomenon, a diffuse increase in 99m Tc-MDP uptake after treatment of bone metastases persisting until 6 months after treatment, is a well-known limitation in the assessment of tumor response and should not be mistaken for disease progression.
Imaging is also widely used to direct biopsies to relevant tumor areas avoiding cystic/necrotic regions. In skull base lesions, most biopsies are performed endoscopically.
General Imaging Features of Bone Lesions Helpful in the Differential Diagnosis
Although, in most cases, the final diagnosis of a bone lesion requires a biopsy specimen, there are several lesions in which a biopsy may be misleading without appropriate radiologic-pathologic correlation. Well-known examples are fibroosseous lesions, such as fibrous dysplasia, often mistaken on pathology for a sarcoma. Therefore, imaging plays an essential role in the diagnosis and further management of patients, particularly in an anatomic area occult to clinical inspection. Most general imaging features of bone tumors in the remainder of the skeletal system apply to the skull base, and one should bear in mind that the skull base is composed of flat bones originating from endochondral ossification.
One of the most important epidemiologic factors affecting the differential diagnosis of a bone tumor is age, with several tumors occurring only before the closure of growth plates and others showing specific incidence patterns. Clinical presentation and setting can also be helpful: in a patient with a known primary cancer and a skull base lesion, a bone metastasis should be high on the differential list, whereas in an immunocompromised patient with a sinonasal or temporal bone infection, skull base osteomyelitis should be highly considered. Symptoms from bone lesions of the skull base are nonspecific and include headache, cranial nerve’ dysfunction, sinonasal obstruction, and proptosis, just to mention the most common.
The general patterns of bone lesions apply to the skull base and help limit the differential diagnosis. Bone lesions can be lytic, characterized by bone destruction or replacement; sclerotic, featured mainly by bone production with ossified or calcified matrix; or mixed, showing elements of both bone destruction and bone formation. Although single, focal lesions suggest a primary benign or malignant bone tumor, diffuse bone involvement is most often seen in fibroosseous dysplasias, Paget disease, renal osteodystrophy, metastatic bone involvement, infection, and lymphoma. The presence of multiple focal bone lesions shortens the differential diagnosis to metastatic bone disease, multiple myeloma, Langerhans cell histiocytosis in pediatric age, brown tumors associated with hyperparathyroidism, and developmental/syndromic lesions such as multiple osteomas or enchondromas associated with osteopoikilosis, melorheostosis, and Maffuci and Ollier syndromes. Patterns of tumor growth and their transition to normal adjacent bone correlate with tumor aggressiveness, with most benign lesions leading to bone expansion and remodeling with a sharp, geographic transition to normal adjacent bone and most malignant lesions showing a moth-eaten, permeative, or destructive pattern with cortical disruption, ill-defined borders, and a wide transition zone. The pattern of periosteal reaction is also useful to differentiate benign slow-growing tumors from aggressive, rapidly growing tumors. The former usually show a thick, laminated, onion-skin pattern of periosteal reaction, whereas the latter show periosteal rupture (Codman triangle), air-on-end, or a spiculated, sunburst pattern. Tumors are further classified into bone forming, containing more or less mineralized osteoid matrix, which can show a ground-glass pattern in the case of fibroosseous lesions; cartilage producing, which often show a calcified matrix, containing chondroid-like calcifications; or neither bone nor cartilage forming, such as hematopoietic, vascular, fibrous, or giant-cell-related tumors.
Developmental Bone Lesions of the Skull Base
Developmental skull base lesions include arrested pneumatization of the skull base, giant and aberrant arachnoid granulations, sphenoid wing dysplasia, and basal cephaloceles.
Arrested pneumatization of the skull base is a common developmental variant. Right before the pneumatization of craniofacial bones, during the development of the sinonasal and otomastoid cavities, there is a conversion of the hematopoietic red bone marrow into fatty yellow marrow. If pneumatization arrests, a lytic, nonexpansive area with fat density/signal intensity, with lobulated sclerotic borders and occasional calcifications, ensues and can be easily mistaken for a pathologic lesion. Frequent locations in the skull base are the clivus and greater sphenoid wing ( Fig. 15.1 ).
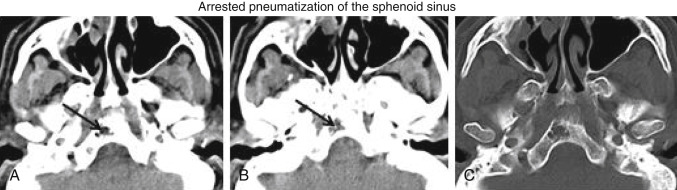
Giant, aberrant arachnoid granulations or arachnoidoceles are another common developmental anomaly seen in the skull base. Arachnoid or Pacchioni granulations are normal projections of arachnoid villi through the dura mater into the dural sinuses that allow cerebrospinal fluid (CSF) flow into the venous system. When over 10 mm in size, they are called giant and are responsible for irregularly shaped lytic lesions, often seen in and around dural venous sinuses, close to penetrating cortical veins. They show as filling defects on postcontrast CT and/or MR images and can mimic dural sinus thrombosis. When these granulations fail to migrate into the venous sinuses, they are called aberrant arachnoid granulations or arachnoidoceles and are most often seen in the floor of the middle cranial fossa and posterior aspect of the temporal bone. These granulations contain trapped CSF, which does not communicate with the venous system, and may enlarge over time as a result of chronic transmission of CSF pulsations, leading to slowly growing lytic defects with sclerotic margins that follow CSF density/signal intensity on CT/MRI and may show faint enhancement of the adjacent dura ( Fig. 15.2 ). Adjacent bone erosion may ensue with secondary herniation of these giant granulations into the paranasal sinuses, temporal bone, or orbit, shown on imaging as meningeal outpouchings or pseudomeningoceles ( Fig. 15.3 ). Although they are usually asymptomatic incidental findings, CSF leaks are a potential complication of these developmental lesions. Aberrant arachnoid granulations can potentially be confused with other lytic skull base lesions on CT. On MRI, however, the diagnosis is usually straightforward, as the lesion follows the signal intensity of CSF in all pulse sequences and does not enhance. A signal intensity slightly above that of CSF on proton density weighted (PDW) and fluid-attenuated inversion recovery (FLAIR) images can be seen and reflects a slight increase in protein concentration inside the trapped fluid.
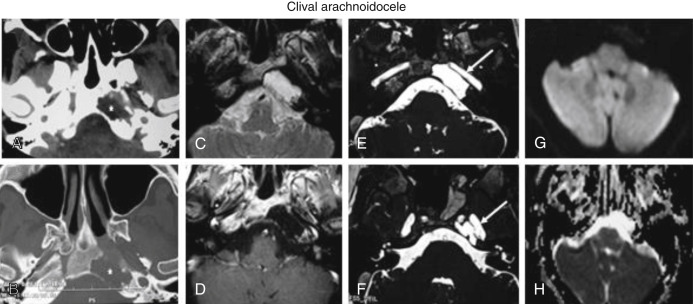
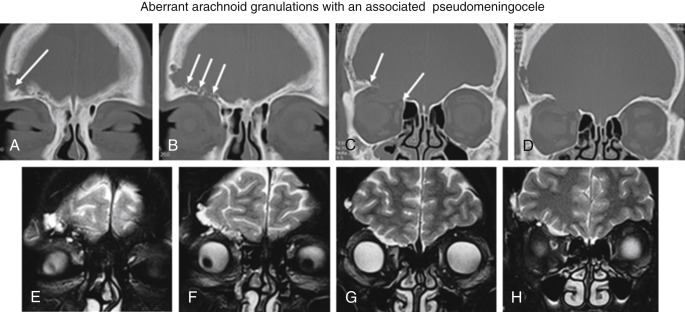
Sphenoid wing dysplasia is a developmental condition that can occur as an isolated finding or as a feature of neurofibromatosis type 1 (NF1) and is one of the six diagnostic criteria for this disease. In isolated cases, it seems to result from defective ossification of the sphenoid bone, whereas in patients with NF1 it may be progressive and secondary to an underlying plexiform neurofibroma. It can be asymptomatic or present clinically as pulsatile exophthalmos, enophthalmos, visual impairment, diplopia, or conjunctivitis. On imaging, it is featured by partial or total absence of the greater sphenoid wing and elevation of the lesser sphenoid wing, leading to widening of the superior orbital fissure, increased anteroposterior diameter of the middle cranial fossa, orbital enlargement, and the appearance of an empty orbit (bare orbit sign). Other associated findings include a middle cranial fossa arachnoid cyst and potential meningeal or temporal pole herniation through the bony defect into the orbit causing secondary proptosis.
Petrous apex cephalocele is rare and represents a congenital or acquired cystic expansion and herniation of the posterolateral Meckel cave into the petrous apex secondary to the chronic transmission of CSF pulsations through a patent porus trigeminus combined with a congenitally thinned or dehiscent bone at the roof of the petrous apex ( Fig. 15.4 ). Acquired cases seem to be secondary to increased intracranial pressure, as there is a known association with an empty sella, pseudotumor cerebri, and expansive intracranial lesions ( Fig. 15.5 ). On imaging, they are featured by a cystic enlargement of Meckel cave with smooth remodeling of the anterior aspect of the superior petrous ridge and, occasionally, containing prolapsed fibers of the trigeminal nerve. Although most cases are incidental and asymptomatic, some have been associated with trigeminal neuropathy, CSF otorrhea, recurrent meningitis, and pulsatile tinnitus, as they may erode posteriorly into the otic capsule and/or pneumatized petrous air cells.
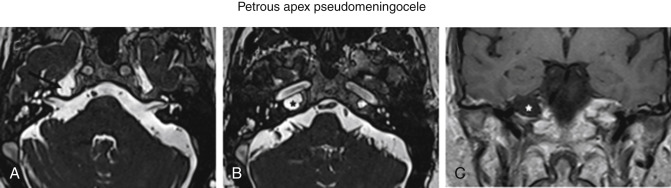
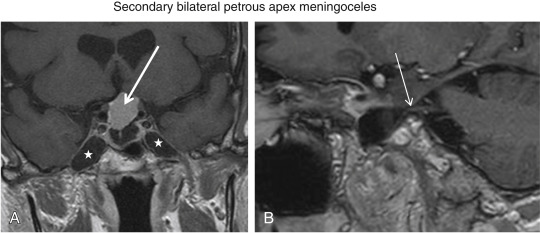
Basal cephaloceles represent herniation of intracranial contents through congenital or acquired skull base defects. There are two different pathophysiologic mechanisms to explain this developmental anomaly: failure of neural tube closure and developmental failure of ossification centers of the skull base. Basal cephaloceles are named according to the anatomic location of the skull base defect as sphenopharyngeal (through the basisphenoid into the pharynx), sphenoorbital (through the superior orbital fissure), sphenoethmoidal (through the sphenoethmoid synchondrosis), transethmoidal (through the cribiform plate), and sphenomaxillary (into the maxillary sinus). Transphenoidal cephaloceles are rare and may occur between the basisphenoid and the greater sphenoid wing, most often resulting from incomplete fusion of their ossification centers. This developmental anomaly, known as the lateral craniopharyngeal or Stenberg canal, provides a weak spot for the herniation of meninges and/or overlying temporal lobe into the lateral recess of the sphenoid sinus ( Fig. 15.6 ). A complete Stenberg canal is seen in 4% of adults, whereas persistent vestiges of this canal are much more common, seen in 30% of individuals. The oval foramen is another potential weak spot for the herniation of intracranial contents into the masticator space ( Fig. 15.7 ).
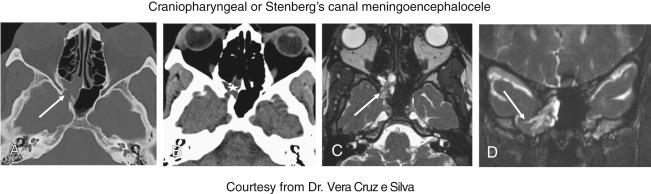
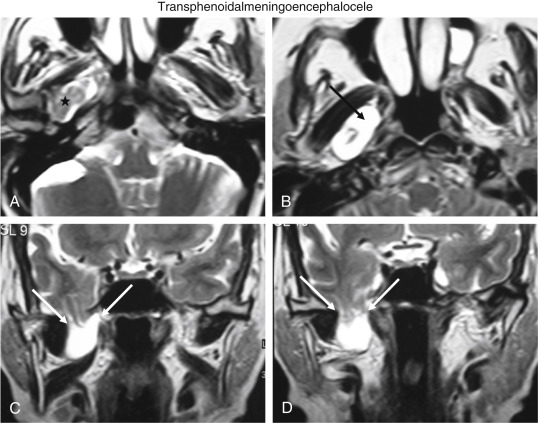
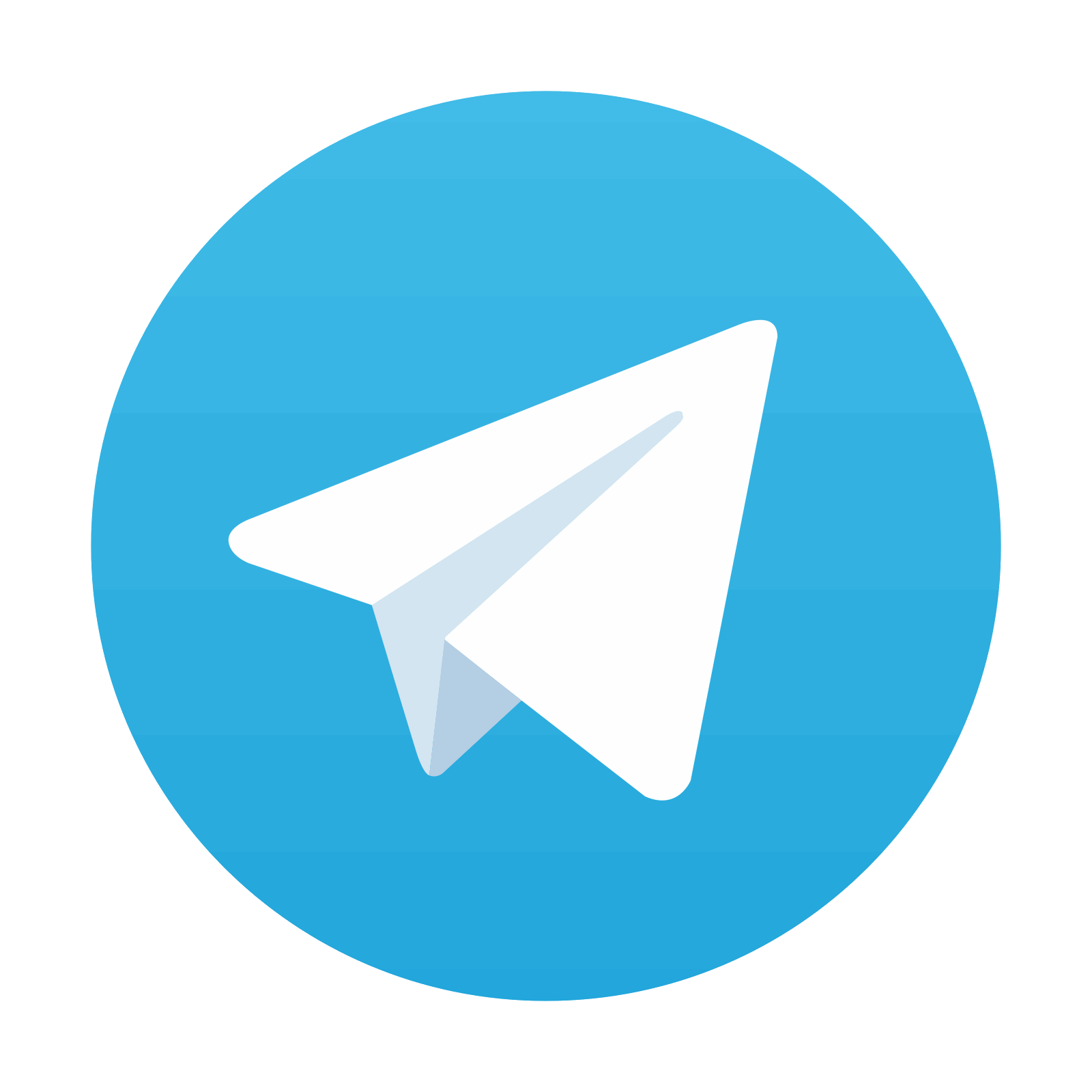
Stay updated, free articles. Join our Telegram channel

Full access? Get Clinical Tree
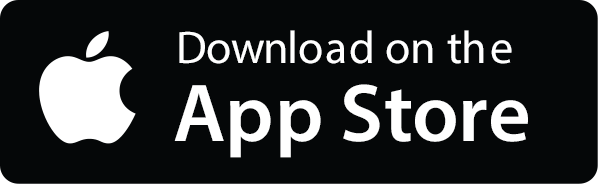
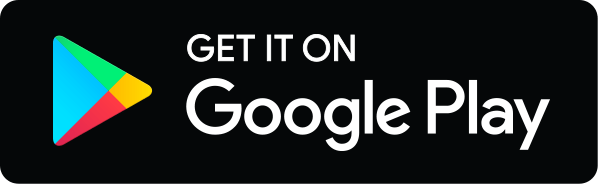
