1. Central feature (essential for a diagnosis of possible or probable DLB)
Dementia defined as progressive cognitive decline of sufficient magnitude to interfere with normal social or occupational function. Prominent or persistent memory impairment may not necessarily occur in the early stages but is usually evident with progression. Deficits on tests of attention, executive function and visuospatial ability may be especially prominent
2. Core features (two core features are sufficient for a diagnosis of probable DLB, one for possible DLB)
Fluctuating cognition with pronounced variations in attention and alertness
Recurrent visual hallucinations that are typically well formed and detailed
Spontaneous features of Parkinsonism
3. Suggestive features (if one or more of these are present in the presence of one or more core features, a diagnosis of probable DLB can be made. In the absence of any core features, one or more suggestive features are sufficient for possible DLB. Probable DLB should not be diagnosed on the basis of suggestive features alone.)
REM sleep behaviour disorder
Severe neuroleptic sensitivity
Low dopamine transporter uptake in basal ganglia demonstrated by SPECT or PET imaging
4. Supportive features (commonly present but not proven to have diagnostic specificity)
Repeated falls and syncope
Transient, unexplained loss of consciousness
Severe autonomic dysfunction, e.g. orthostatic hypotension, urinary incontinence
Hallucinations in other modalities
Systematised delusions
Depression
Relative preservation of medial temporal lobe structures on CT/MRI scan
Generalised low uptake on SPECT/PET perfusion scan with reduced occipital activity
Abnormal (low uptake) MIBG myocardial scintigraphy
Prominent slow wave activity on EEG with temporal lobe transient sharp waves
5. A diagnosis of DLB is less likely
In the presence of cerebrovascular disease evident as focal neurologic signs or on brain imaging
In the presence of any other physical illness or brain disorder sufficient to account in part or in total for the clinical picture
If Parkinsonism only appears for the first time at a stage of severe dementia
6. Temporal sequence of symptoms
DLB should be diagnosed when dementia occurs before or concurrently with Parkinsonism (if it is present). The term Parkinson disease dementia (PDD) should be used to describe dementia that occurs in the context of well-established Parkinson disease. In a practice setting, the term that is most appropriate to the clinical situation should be used and generic terms such as LB disease are often helpful. In research studies in which distinction needs to be made between DLB and PDD, the existing 1-year rule between the onset of dementia and Parkinsonism DLB continues to be recommended. Adoption of other time periods will simply confound data pooling or comparison between studies. In other research settings that may include clinicopathologic studies and clinical trials, both clinical phenotypes may be considered collectively under categories such as LB disease or alpha-synucleinopathy
However, some research indicates that the clinical diagnostic criteria have suboptimal accuracy when differentiating DLB from AD. For example, Verghese et al. (1999) found that the clinical criteria distinguished DLB from AD with a sensitivity of 78 % and a specificity of 64 % when measured against the ‘gold standard’ of autopsy. The difficulty in differentiating DLB from AD in life stems from the fact that both DLB and AD feature cognitive impairment as well as behavioural disturbance. The differential diagnosis is important because optimal treatments vary between DLB and other disorders. Neuroleptic medication may be used to treat neuropsychiatric symptoms in AD, but is contraindicated in DLB, as it can lead to worsening of parkinsonian symptoms and acute confusion. Cautious treatment with dopaminergic agents can improve motor symptoms in DLB. However, it can exacerbate psychiatric symptoms such as psychosis and impulsivity (Barbas 2006). Treatment options in DLB remain limited in general, and further research is needed in order to identify potential targets for intervention. There is also a need to address questions about the relevance of various neuropathological changes in DLB and how they relate to one another and clinical symptoms.
Nuclear and molecular imaging has been applied to address these problems. Nuclear imaging studies have improved diagnostic accuracy, identified potential disease mechanisms and followed the progression of medicated and unmedicated patients. These studies have involved the identification of ‘biomarkers’. Biomarkers can be defined as ‘a characteristic that is objectively measured and evaluated as an indicator of normal biological processes, pathogenic processes, or pharmacological responses to a therapeutic intervention’ (Atkinson et al. 2001; p. 91). The European Federation of Neurological Societies (EFNS) offers guidance that there are two types of biomarkers: biomarkers of disease state, i.e. indicators of diagnosis, and biomarkers of disease stage, i.e. the progression of the disease (Filippi et al. 2012).
This chapter reviews findings from PET and SPECT studies in DLB, including exploratory studies as well as those that attempted to identify biomarkers. We will first discuss metabolism and perfusion, followed by molecular pathology and deficits in neurotransmitter systems. Neuroimaging investigations using PET and SPECT have also allowed the relationship between brain pathology and symptoms to be studied, and this too will be outlined.
17.2 Metabolism in DLB
Brain metabolism in DLB has been studied extensively using PET and 18F-fluorodeoxyglucose (18F-FDG). This has allowed researchers to investigate the regional cerebral metabolic rate of glucose (rCMRglc) in the disease. Consistent patterns of metabolism have been identified, which are significantly different from those observed in both healthy control subjects and in subjects with other neurodegenerative diseases (see Table 17.2).
Table 17.2
PET studies of metabolism in DLB
Study | Subjects | Findings in DLB | |||||
---|---|---|---|---|---|---|---|
DLB | AD | PD | PDD | Other | Control | ||
Albin et al. (1996) | 6 | – | – | – | – | – | Marked metabolic reductions in many cortices compared to normal database |
Imamura et al. (1999) | 22 | 16 | – | – | – | – | DLB patients with visual hallucinations (VH) had milder hypometabolism compared to DLB patients without VH in right posterior temporal and parietal areas |
Ishii et al. (1998) | 12 | 12 | – | – | – | 12 | Metabolism in DLB and AD generally lower than in controls. DLB had lower metabolism than AD in occipital lobes |
Yong et al. (2007) | 7 | – | 16 | 13 | – | 15 | DLB and PDD exhibited similar metabolism, except patients with DLB had decreased metabolism in lateral temporal area |
Mosconi et al. (2008) | 27 | 199 | – | – | 212 | 110 | Standardised disease-specific PET patterns were developed that correctly classified 92 % of patients with DLB |
Teune et al. (2010) | 6 | 15 | 20 | – | 55 | – | Disease-specific patterns of relatively decreased metabolic activity were found in DLB (occipital and parietotemporal regions) |
17.2.1 Hypometabolism and Differential Diagnosis of DLB Versus AD
Albin et al. (1996) studied six subjects with a neuropathologically confirmed diagnosis of DLB or DLB plus AD. Using 18F-FDG, they identified diffuse cerebral hypometabolism in all subjects relative to a database of normal controls. It was found that metabolism in sensorimotor and subcortical regions was relatively spared. The authors noted that these results constituted a metabolic pattern previously associated with AD but also that all their participants had marked hypometabolism in the occipital cortex, a finding not previously observed in AD. A later study by Ishii et al. (1998) had similar results, finding that metabolism across the cerebral cortex was generally lower in patients with DLB (n = 12) and AD (n = 12) than in normal controls. When compared directly, the only difference between DLB and AD was a relative occipital hypometabolism in DLB (see Fig. 17.1). They noted that if the lowest absolute value for occipital metabolism from the control group was used as a cut-off, 8 of 12 subjects with DLB fell below it, whilst this was true for only 1 of 12 subjects with AD. This yields a sensitivity of 67 % and a specificity of 92 % for differentiating DLB from AD. Ishii et al. also distinguished that metabolism in the sensorimotor cortex did not differ between any of the groups and can thus be used as a reference region against which metabolic values from the rest of the brain can be normalised. This procedure standardises intersubject differences in metabolic rate. Using occipital metabolism normalised to the sensorimotor cortex, Ishii et al. demonstrated that subjects with DLB could be differentiated from those with AD with a sensitivity and specificity of 92 %.
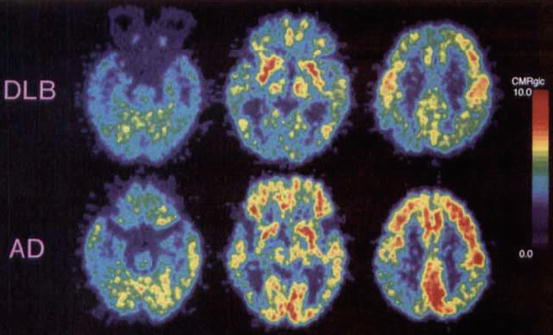
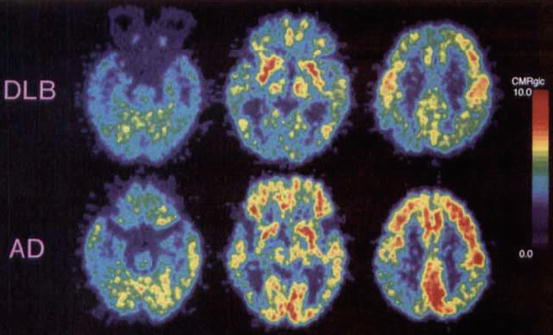
Fig. 17.1
Representative dementia with Lewy bodies (DLB) and Alzheimer’s disease (AD) CMRglc images. The DLB patient (subject 8) was a 76-year-old woman with an MMSE score of 21, whereas the patient with AD was a 75-year-old woman with an MMSE score of 23. These patients had almost the same age and dementia severity, though the global CMRglc in the patient with DLB was lower than that in the patient with AD and especially decreased in the occipital lobe (Reproduced from Ishii et al. (1998) with permission from Elsevier)
In addition to occipital hypometabolism, Lim et al. (2009) showed that relative preservation of the mid or posterior cingulate gyrus (cingulate island sign) had a very high specificity for a diagnosis of DLB (100 %). If this finding is replicated, this might be an even more useful pointer to the diagnosis of DLB than occipital hypometabolism alone.
In a later study by the same group, Ishii et al. (2007) found that the ratio of occipital to hippocampal metabolism was significantly reduced in subjects with DLB compared to subjects with AD or normal controls. When applied, this ratio could be used to differentiate between subjects with DLB and AD with 87 % accuracy. Gilman et al. (2005) compared the relative utility of 18F-FDG and neuropsychological testing for differentiating DLB from AD. They concluded that PET was more robust at differentiating groups than neuropsychological testing, with the exception of family ratings of patient motor functioning, which was diagnostically useful in the later stages of disease.
17.2.2 Differential Diagnosis of DLB and Other Clinical Syndromes
Mosconi et al. (2008) studied 548 subjects with 18F-FDG, including 110 normal controls, 114 subjects with mild cognitive impairment (MCI), 199 with AD, 98 with frontotemporal dementia (FTD) and 27 with DLB. They investigated whether rCMRglc could differentiate the various dementia types, MCI and normal aging from one another. The researchers split their subjects into two groups. They used the first ‘training’ cohort to identify specific patterns of rCMRglc associated with each group, and they used the second ‘testing’ cohort to assess the accuracy of these patterns at correctly diagnosing subjects. In the training cohort, Mosconi et al. found that 10 of 14 subjects with DLB (71 %) had hypometabolism in posterior brain regions preferentially involving the occipital cortex. The remaining four patients showed additional parietotemporal and posterior cingulate cortex (PCC) hypometabolism. Mosconi et al. noted prominent parietotemporal hypometabolism in subjects with AD and prominent frontal or temporal hypometabolism in subjects with FTD. In the training cohort, these criteria distinguished DLB from AD with 71 % sensitivity and 99 % specificity and DLB from FTD with 71 % sensitivity and 65 % specificity. If hippocampal metabolism was considered in addition to cortical evaluations, DLB could be distinguished from AD with 100 % sensitivity and 98 % specificity. This was due to hippocampal hypometabolism being present in 98 of 100 subjects with AD but in only 3 of 14 subjects with DLB. Adding hippocampal hypometabolism to the criteria did not enhance diagnostic accuracy between DLB and FTD. Mosconi et al. then applied these findings to their testing cohort for validation of the diagnostic criteria. In this cohort, 12 of 13 subjects with DLB (92 %) had occipitoparietal hypometabolism and were thus classified correctly. None of the other groups’ subjects were classified as DLB. Mosconi et al.’s criteria thus had 92 % sensitivity and 100 % specificity for differentiating DLB from AD, FTD, MCI and normal aging.
Teune et al. (2010) found broadly similar patterns of hypometabolism to those observed by Mosconi et al. (2008). Teune et al. (2010) analysed retrospective PET scans in patients with PD, multiple system atrophy (MSA), progressive supranuclear palsy (PSP), corticobasal degeneration (CBD), DLB, AD and FTD. The scans were performed in the early stages of the patients’ diseases at a time when many of their diagnoses were still uncertain. However, the patients had confirmed clinical diagnoses by the end of the study. Teune et al. (2010) wanted to investigate whether early PET scans could be used to predict subsequent clinical diagnoses. They established that patients with DLB showed the signature occipital hypometabolism and that this was present in early stages of the disease when clinical diagnosis was still difficult. They concluded that 18F-FDG PET can be used to differentiate between neurodegenerative diseases in their early stages with good reliability.
Yong et al. (2007) compared cerebral metabolism in patients with PD, DLB and PDD as well as healthy controls. They concluded that patients with DLB and those with PDD exhibited largely similar metabolic patterns, except that DLB featured additional metabolic reduction in the lateral temporal area, as well as slightly more exaggerated global hypometabolism. They also found that occipital metabolism was not significantly changed in the DLB and PDD patients relative to the PD patients.
17.2.3 Hypometabolism and Different Symptomatology
Researchers have investigated if regional cerebral metabolic rates differ across subjects with DLB depending on the clinical picture observed. Imamura et al. (2001) tested the hypothesis that occipital hypometabolism in PD/DLB is driven by nigrostriatal dysfunction. They noted that an association between Parkinsonism and occipital hypometabolism in DLB would support this hypothesis. Their hypothesis followed the work of Bohnen et al. (1999), who found that PD patients had greater occipital hypometabolism in the hemisphere contralateral to their more affected side. However, Imamura et al. (2001) found no difference in occipital hypometabolism between subjects with DLB with Parkinsonism and DLB without Parkinsonism. They also found that subjects with DLB without Parkinsonism had significantly lower occipital metabolism compared to AD subjects or controls. These observations suggest that the occipital hypometabolism observed in DLB is not primarily driven by nigrostriatal dysfunction.
Imamura et al. (1999) compared 16 subjects with DLB suffering from visual hallucinations (VH), 6 subjects with DLB without VH and 16 subjects with AD without VH. They observed the previously reported lower occipital hypometabolism in subjects with DLB compared to AD. In addition, their subjects with DLB had lower metabolism in frontal, posterior temporal and parietal areas. This study also noted that subjects with DLB and VH had milder hypometabolism in right posterior temporal and parietal areas compared to subjects with DLB without VH. There was no significant difference in occipital metabolism between patients with DLB with and without VH. Imamura et al. suggested that VH might stem from lesions in the visual system causing pathological activation of visual representations stored in sensory association cortices. This process might require preserved metabolism in the posterior temporal and parietal association cortices. Kantarci et al. (2012) found that greater occipital hypometabolism was associated with more frequent VH in a sample of 21 patients with DLB.
17.2.4 Correlation Between Hypometabolism and Pathology
There has been some discussion about the cause of the occipital hypometabolism observed in DLB. Higuchi et al. (2000) studied the brains of 19 subjects with AD, 17 subjects with DLB and 11 normal controls at autopsy, with a further 11 subjects with AD, 7 subjects with DLB and 10 normal controls studied using 18F-FDG PET. They found that α-synuclein- and ubiquitin-positive LB were least common in the occipital lobes of subjects with DLB compared to other brain regions. Conversely, they found extensive white matter spongiform changes in the DLB group most pronounced occipitally, with mild to moderate changes in the normal and AD groups. Higuchi et al. (2000) also noted more severe white matter gliosis in the brains of DLB subjects relative to AD, with the greatest gliosis observed occipitally in DLB. The authors concluded that white matter spongiform pathology and gliosis may be an important pathological substrate for the pattern of impaired glucose metabolism seen in DLB. The present authors note that LB are intracellular and may be lost during gliosis. This could account for the finding of minimal LB in occipital lobes.
17.2.5 Conclusion
The most reliable finding from the studies assessed is the relative occipital hypometabolism in DLB compared with AD and controls. Studies have demonstrated that this difference can separate DLB from AD and controls with high sensitivity and specificity. This procedure is reliable enough for use in clinical practice. Occipital hypometabolism is not associated with Parkinsonism in DLB, but may be associated with VH. One study suggests that hypometabolism is associated with spongiform pathology and gliosis rather than LB, but it is difficult to rule out a role for LB since they may be lost during gliosis.
17.3 Cerebral Perfusion in DLB
Results from cerebral perfusion SPECT imaging in DLB are not as uniform as those from metabolic studies. However, this technique has been successfully used in differential diagnosis of DLB and is more easily available than metabolic scans. Ligands commonly used are 99mTc-hexamethylpropylene amine oxime (99mTc-HMPAO), 99mTc-ethyl cysteinate dimer (99mTc-ECD) and N-isopropyl-p-123I-iodoamphetamine (123I-IMP).
Investigators have observed that 99mTc-HMPAO, 99mTc-ECD and 123I-IMP show different perfusion in several brain areas including the occipital lobes (Shimizu et al. 2005). Van Dyck et al. (1996) found that 99mTc-ECD is stable for a much longer time than 99mTc-HMPAO and therefore differentiates grey and white matter with greater clarity, but both 99mTc-HMPAO and 99mTc-ECD are particularly effective at facilitating AD diagnosis. The results from Odano et al. (1997) suggested that 123I-IMP was superior to 99mTc-ECD and 99mTc-HMPAO in the detection of occipital hypoperfusion in DLB, indicating that the detection of occipital hypoperfusion may depend on the ligand used (Koulibaly et al. 2003).
The analysis of perfusion studies has greatly altered over the last 20 years. Early studies used simple visual analysis which had drawbacks such as lack of inter-reader reliability and within-reader reproducibility. Now, more sophisticated statistical analysis techniques are used such as statistical parametric mapping (SPM), 3-dimensional stereotactic surface projection technique (3D-SSP), easy z-score imaging system (eZIS), receiver operating characteristic (ROC) analysis, region of interest (ROI) data sets and principal component analysis (PCA) all of which have improved the consistency between studies.
17.3.1 Studies Using 99mTc-Hexamethylpropylene Amine Oxime
Donnemiller et al. (1997) studied six patients with AD and seven patients with DLB using 99mTc-HMPAO or 99mTc-ECD dependent on availability. Visual analysis found significant differences in the regional cerebral blood flow (rCBF) between AD and DLB patients. The AD group had bilateral temporal and parietal hypoperfusion. In the DLB group (and one AD patient), there was bilateral parieto-occipital hypoperfusion, displayed as a ‘horseshoe-like shape’. Lobotesis et al. (2001) reported occipital hypoperfusion in 15 of 23 DLB patients (65 %) compared with only 9 of 50 AD cases (18 %) with a sensitivity of 64 % and specificity of 86 %.
Kemp et al. (2007) evaluated the diagnostic utility of 99mTc-HMPAO SPECT for individual patients with suspected DLB in a clinical setting, concentrating on occipital perfusion. Of 84 subjects, 39 (44 %) had a high probability of having DLB as shown by abnormal 123I-FP-CIT SPECT scans; of this group, 11 (28 %) demonstrated occipital hypoperfusion which was also evident in 14/45 (31 %) of the non-DLB group. This study acknowledges that previous research relied on clinical diagnosis only, which would suggest patients were at a more advanced stage of the disease when scanned. Kemp et al. concluded that occipital lobe appearances are not specific enough to confirm a diagnosis as deficits are evident in both DLB and non-DLB subjects. In their 2009 review, Morgan and Walker suggest that some discrepancies in the above findings may be attributed to the older studies using the 1996 consensus criteria for DLB (McKeith et al. 1996). Kemp et al.’s use of 123I-FP-CIT SPECT is advantageous as this is at present regarded to be the most reliable indicator for the clinical diagnosis of DLB (Walker et al. 2007). This has been corroborated by Colloby et al. (2008) who found that for AD and DLB, consensus diagnosis matched imaging diagnosis in 56 % of cases using perfusion scans and 84 % using 123I-FP-CIT (see Fig. 17.2). Colloby et al. (2010a) found principal component analysis very useful in identifying differences between AD and DLB and were able to differentiate these patients with a sensitivity of 73 % and specificity of 72 %. This discrimination was made on the basis of perfusion in the bilateral cerebellum, bilateral medial temporal, bilateral thalamus and right striatal regions.
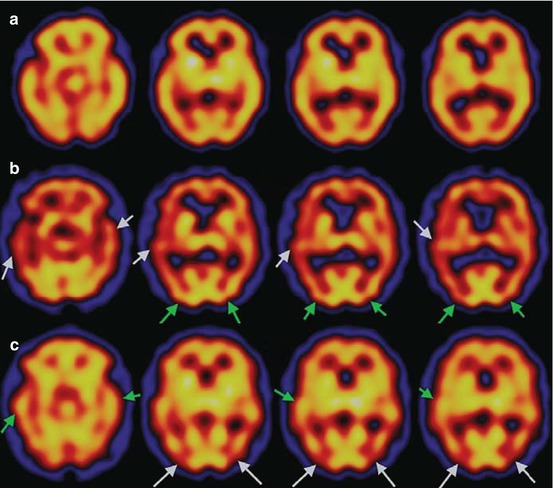
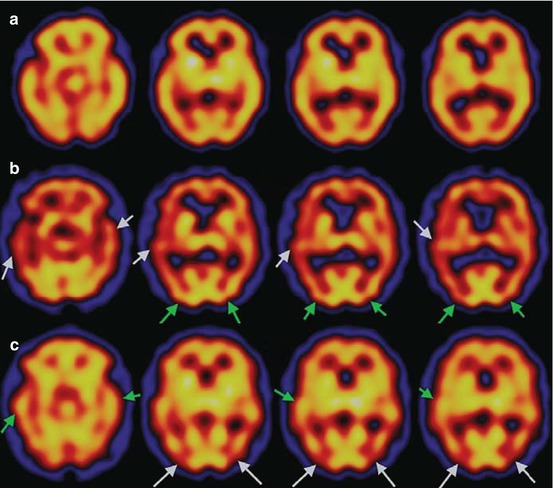
Fig. 17.2
Representative axial 99mTc-exametazime SPECT scans of groups in standard MNI space. (a) Control: perfusion is relatively well preserved in cortical regions. (b) AD: loss of uptake in temporal and medial temporal lobes (white arrows). Observe that occipital binding remains largely unaffected (green arrows). (c) DLB: reduced uptake in occipital lobe (white arrows), whilst temporal and medial temporal blood flow is relatively unchanged (green arrows). Red indicates low perfusion, and yellow indicates higher perfusion (Reproduced from Colloby et al. (2008) with permission from Cambridge University Press)
Patients with PDD have been shown to have similar patterns of occipitoparietal hypoperfusion to those with DLB (Colloby et al. 2002; Pasquier et al. 2002; Firbank et al. 2003). Defebvre et al. (1999) found that compared to PD, DLB showed a decreased uptake of 99mTc-HMPAO in cortical areas with the exception of the posterior frontal and occipital regions. Patients with DLB also had significantly lower global 99mTc-HMPAO uptake compared with patients with AD. This suggests diffuse cortical abnormalities in DLB. Firbank et al. (2005) tracked changes in perfusion in DLB and PDD subjects over 1 year, using SPM99. They observed that the DLB, but not the PDD, group demonstrated significantly increased perfusion in the putamen and a nonsignificant but notable increase in the left cerebellum relative to controls. Furthermore, the researchers observed a correlation between increasing striatal perfusion and worsening motor symptoms in both patient groups, independent of antiparkinsonian medication changes. Mori et al. (2006) explored the neural substrate of visual hallucinations (VH) in DLB by investigating changes in rCBF and VH before and after cholinesterase inhibitor treatment. Hallucinations decreased while receiving therapy, whereas occipital rCBF focally increased.
17.3.2 Studies Using 99mTc-Ethyl Cysteinate Dimer (ECD)
Pasquier et al. (2002) were among the first to use 99mTc-ECD specifically for the diagnosis of DLB. Using ROI analysis, DLB was correctly identified in 22/34 patients (sensitivity, 65 %) and AD was correctly identified in 20/28 patients (specificity, 71 %). Mean perfusion indices in the right and left occipital regions were lower in DLB than in AD patients. Conversely, the mean perfusion index in the left medial temporal region was lower in AD than in DLB patients. Within the DLB group, significantly lower mean perfusion indices were observed in the right occipital region of the 26 DLB patients with VH as compared to the eight DLB patients without VH. These findings support a link between VH and structural/functional changes in the occipital region in DLB patients. Ceravolo et al. (2003) also assessed the perfusional pattern in DLB and AD. SPM was used for analysis and confirmed a significant decrease in temporoparietal blood flow in AD compared to DLB and significant occipital hypoperfusion in DLB compared to AD. It was acknowledged that the findings support the hypothesis that occipital hypoperfusion may account for visual hallucinations in DLB, although in this study no correlation was found between occipital hypoperfusion and visuospatial abnormalities.
Waragai et al. (2007) evaluated brain perfusion SPECT scans in patients with various neurodegenerative diseases at an early stage. Using eZIS, a computer-aided statistical analysis of rCBF, the researchers found patterns of perfusion consistent with earlier reports. Specific patterns were visible for subjects with AD, DLB, FTD, PD, vascular Parkinsonism, multiple system atrophy of cerebellar type, cortical cerebellar atrophy and amyotrophic lateral sclerosis. Decreased rCBF was observed in the occipital lobe, precuneus gyrus and posterior cingulate cortex in DLB. The limitation with this study was that the sample groups were too small to compare with one another and so they were compared to normal controls. However, a specific rCBF pattern was observed for each disease, indicating that eZIS analysis of perfusion SPECT images may be useful for early and differential diagnosis of DLB.
Tateno et al. (2008) found that DLB subjects had a lower bilateral rCBF in posterior cerebral segments compared to AD. Using the automated ROI analysis software 3DSRT and clinical symptoms, it was found that DLB patients with Parkinsonism had lower rCBF through the cerebrum with statistical significance in the posterior cerebral segments. This study suggests a relationship between occipital hypoperfusion and Parkinsonism in DLB; however, this is not in keeping with a PET metabolic study reported in the previous section (Imamura et al. 2001).
17.3.3 Studies Using N-isopropyl-p-123iodoamphetamine
Ishii et al. (1999) used SPM to reveal that rCBF was lower in the occipital lobes and higher in the right medial temporal lobe in patients with DLB compared to patients with AD. However, they also found AD-like patterns of perfusion among DLB participants. Shimizu et al. (2005) replicated the finding of relative occipital hypometabolism in patients with DLB relative to AD. Receiver operating characteristic (ROC) analysis revealed that rCBF measurement of the medial occipital lobe, including the cuneus and lingual gyrus, demonstrated sensitivity of 85 % and specificity of 85 % in discriminating DLB from AD using 3D-SSP.
Mito et al. (2005) made comparisons between DLB, PD, PDD and AD using 123I-IMP and found that patients with DLB had a shorter disease duration and greater blood flow reduction compared to patients with PDD. It was concluded that different regional patterns suggest disease-specific combinations of underlying pathological and neurochemical processes. Inui et al. (2007) compared the results of 123I-IMP SPECT scans between 9 patients with possible DLB, 12 with probable DLB and a database of normal controls. The researchers observed decreased perfusion in the parietotemporal and occipital cortices, posterior cingulate and precuneus regions in the probable DLB group but no significant reduction in the possible DLB group, where the diagnosis is much less reliable.
Combining different imaging and clinical measures can be useful in aiding differential diagnosis as shown in the next two studies. Hanyu et al. (2006) found that medial occipital perfusion was significantly decreased in DLB patients compared to AD patients. Applying an equation using z-scores from the medial occipital lobe and a weighted index for Mini-Mental State Examination (MMSE) sub-scores of DLB and AD patients, differentiation of DLB and AD was possible with sensitivity of 81 % and specificity of 85 %. Combined SPECT/MMSE diagnosis was more accurate than imaging diagnosis alone. This demonstrates that MMSE sub-scores used to routinely measure cognitive deficits can be useful tools in enhancing the interpretation of SPECT results to differentiate between AD and DLB. Additionally, combinations of SPECT and MRI have been found useful in differentiation of AD and DLB (sensitivity, 89 %; specificity, 84 %) finding that DLB patients had significantly lower rCBF than AD patients (Goto et al. 2010).
17.3.4 Conclusion
Overall, studies have generally demonstrated abnormalities in a number of areas in DLB. A degree of overlap exists between AD and DLB in regional uptake patterns across various studies (Colloby et al. 2002; Goto et al. 2010). The aspect most useful for differential diagnosis from AD is occipital hypoperfusion (which also has a relationship to clinical measures). At this point, a link between hypoperfusion and LB can only be speculative because very few LB are present in the occipital lobes (Ishii et al. 1999). However, VH (a core feature) and perceptual abnormalities (a supportive feature; Firbank et al. 2003) appear to be related to the occipital region. Differences in rCBF in the occipital lobe within samples of DLB may be accounted for by differences in presentations between individuals (Donnemiller et al. 1997; Pasquier et al. 2002). Mori et al. (2006) proposed that optimum best practice would involve scanning participants during, before and after hallucinations for comparison.
The reliability of the finding of occipital hypoperfusion in DLB has been questioned by more recent studies that validated DLB diagnoses using 123I-FP-CIT scans. For example, Kemp et al. (2007) did not observe occipital hypometabolism consistently in DLB cases, and Colloby et al. (2008) found that only 60 % of diagnoses made on the basis of perfusion scans matched clinical diagnoses of DLB in a sample largely validated using 123I-FP-CIT. Earlier studies may have used patients in later stages of disease, increasing the frequency with which occipital hypoperfusion was observed. However, early imaging biomarkers are more clinically useful, and perfusion SPECT does not fulfil these requirements.
Variations in the specificity and sensitivity between studies can be partially accounted for by differences in methodology, sample sizes, ligands, scanners and methods of analysis. Particular problems associated with qualitative and semi-quantitative studies include increased subjectivity, poor reproducibility and acquisition of information only in pre-selected regions of interest. The newer statistical brain mapping techniques are superior because the images taken with the gamma camera are compared statistically on a pixel-by-pixel basis with a normal database by the data being superimposed on a standard anatomical brain atlas. This makes it possible to visually evaluate abnormalities occurring outside vascular territories and to assess the association areas, which was difficult to do using conventional tomographic methods. Also advantageous is that the statistical method can define an abnormal pattern characteristic of a disease by comparison between a group of subjects and the normal database as well as between individuals and over time (i.e. pre- and posttreatment) resulting in more objective evaluation of the severity, extent and localisation of regional abnormalities. However, this has not yet been validated by large multicentre or postmortem studies (Morgan and Walker 2009).
Overall the outcomes of perfusion SPECT scans for DLB are not conclusive, but they are a very good indicator particularly when paired with other readily available clinical measures (e.g. MRI, neurocognitive testing). Overall perfusion SPECT scanning is a useful, supportive and accessible tool for clinical settings (Table 17.3).
Table 17.3
SPECT studies of perfusion in DLB
Study | Subjects | Findings in DLB | |||||
---|---|---|---|---|---|---|---|
DLB | AD | PD | PDD | Other | Control | ||
Defebvre et al. (1999) | 20 | 20 | 20 | – | – | – | Diffuse cortical abnormalities in DLB with significant frontal hypoperfusion relative to AD. No occipital deficits |
Lobotesis et al. (2001) | 23 | 50 | – | – | – | 20 | Reduced rCBF in parietal and temporal regions in DLB and AD. Occipital deficits in DLB. 65 % sensitivity, 87 % specificity |
Kemp et al. (2007) | 39 | – | – | – | 45 | – | Occipital hypoperfusion found in DLB and non-DLB dementia |
Pasquier et al. (2002) | 34 | 28 | – | – | – | – | Hypoperfusion in right and left occipital regions in DLB. 64 % sensitivity, 86 % specificity |
Colloby et al. (2010a) | 30 | 36 | – | – | – | – | Topographic expression differentiated DLB from AD with 73 % sensitivity and 72 % specificity |
Ceravolo et al. (2003) | 20a | 24a | – | – | – | – | Relative occipital hypoperfusion in DLB; dopaminergic nigrostriatal functioning also impaired |
Shimizu et al. (2005) | 20 | 75 | – | – | – | 28 | DLB: relative hypoperfusion in medial/lateral occipital lobes which differentiated from AD with 85 % sensitivity and 85 % specificity |
Hanyu et al. (2006) | 36a | 96a | – | – | – | – | Relative medial occipital hypoperfusion in DLB. Sensitivity 75 %, specificity 78 % |
Goto et al. (2010) | 19 | 19 | – | – | – | – | DLB patients had significantly lower grey matter volume in the striatum and significant lower rCBF versus AD. 89 % sensitivity, 84 % specificity |
17.4 Amyloid Deposition in DLB
In recent years, there has been an increasing interest in the role of amyloid in the pathogenesis of dementia. Amyloid is a general term used to describe polypeptide filaments that are folded into a cross β-sheet structure (Lockhart et al. 2007). In the brain, β-amyloid typically aggregates in spherical senile plaques, which can be differentiated into neuritic or diffuse plaques. β-amyloid may also accumulate in the form of cerebrovascular amyloid. Postmortem evidence of β-amyloid is necessary for the diagnosis of definite AD, and studies have observed extensive cortical β-amyloid deposition in AD. However, cerebral β-amyloid is not always associated with clinical dementia, since 20–30 % of non-demented older adults have moderate to severe numbers of cortical neuritic plaques (Price and Morris 1999; MRC CFAS 2001). Researchers have postulated that amyloid deposition is an early event in the disease process and occurs before cognitive impairment becomes apparent (Villemagne et al. 2011), now referred to as prodromal AD. This idea is supported by the results of Engler et al. (2006), who found that amyloid deposition remained stable in 16 patients with AD over a period of 2 years.
Until the first decade of the twenty-first century, researchers could only measure cerebral β-amyloid at postmortem or from a tissue biopsy. Thus, efforts were made to synthesise a 11C-labelled version of the amyloid-binding histological dye, thioflavin-T, for use with PET. These efforts culminated in the development of N-methyl-[11C]2-(4′-methylaminophenyl)-6-hydroxybenzothiazole, which is known as ‘Pittsburgh compound B’ (PiB). Klunk et al. (2004) injected 16 AD patients and 9 controls with PiB and scanned them with PET. They found that, compared to controls, the AD patients showed increased PiB retention. Notably, marked retention was observed in areas of association cortex known to contain large amounts of β-amyloid at postmortem in AD. Klunk et al. concluded that PET imaging with PiB can provide quantitative information on amyloid deposits in vivo. A multitude of studies followed Klunk et al.’s, and their findings have been extensively replicated. Although amyloid is most strongly associated with AD, autopsy series of adults with dementia report that 15–30 % of brains show senile plaques and LB (Galasko et al. 1996), suggesting that many patients with clinical DLB may also have cerebral β-amyloid. This section reports research that has used PET and PiB or newer ligands to image amyloid in vivo in patients with DLB.
17.4.1 Amyloid Frequency and Distribution
Studies imaging amyloid in vivo in DLB have reported a range of frequencies of increased amyloid. These frequencies range from 29 % (Villemagne et al. 2011, 2012) to 90 % (Rowe et al. 2007; see Table 17.4). Foster et al. (2010) offer an explanation as to why such great variation is reported. They found raised amyloid in just one third of patients with DLB and noted that this was much lower than previous reports. Foster et al. (2010) suggest that the source of patient recruitment has a strong bearing on the observed frequency of amyloid. They note that studies recruiting DLB patients from movement clinics (including their own) observe low rates of amyloid, whereas studies recruiting patients from dementia clinics observe higher rates.
Table 17.4
PET and SPECT studies of amyloid deposition in DLB
Study | Subjects | Findings in DLB | |||||
---|---|---|---|---|---|---|---|
DLB | AD | PD | PDD | Other | Control | ||
Rowe et al. (2007) | 10 | 17 | – | – | 15 | 27 | 9/10 (90 %) of DLB patients had raised amyloid. In DLB, high neocortical binding correlated with shorter time between the onset of cognitive impairment and development of the diagnostic clinical features (r = −0.75, p = 0.01) |
Edison et al. (2008a) | 13 | – | 10 | 12 | – | 41 | 11/13a (84.6 %) of DLB patients had raised amyloid. Maximum mean increases in binding in DLB were in the anterior or posterior cingulate followed by the frontal, parietal, temporal and occipital cortex |
Gomperts et al. (2008) | 8 | 15 | 11 | 7 | – | 37 | 7/8 (87.5 %) of DLB patients had raised amyloid. Cortical amyloid burden was higher in the DLB group than in the PDD group and comparable to the AD group |
Maetzler et al. (2009) | 9 | – | 14 | 12 | – | – | 4/9 (44.4 %) of DLB patients had raised amyloid. Unlike PiB(−) Lewy body disease patients, all PiB(+) patients were demented |
Foster et al. (2010) | 6 | – | 17 | 15 | – | 9 | 2/6 (33.3 %) of DLB patients had raised amyloid. No differences were identified in PiB binding among the healthy controls, PD without MCI, PD with MCI, PDD and DLB groups |
Kantarci et al. (2012) | 21 | 21 | – | – | – | 42 | 11/21 (52.4 %) of DLB patients had raised amyloid. The global cortical PiB retention ratio in patients with DLB was lower than in patients with AD (p < 0.001) but higher than normal controls (p = 0.03) |
Villemagne et al. (2012) | 7 | 5 | 5 | – | – | 3 | 2/7 (28.6 %) of DLB patients had raised amyloid. DLB patients with a high β-amyloid burden had a 3.7 times shorter time from onset of symptoms to clinical diagnosis |
Gomperts et al. (2012) | 18 | – | 29 | 12 | 14 | 85 | Amyloid deposition was higher in DLB than PD, PD-MCI, PDD or controls. Amyloid deposition was associated with cognitive impairment in DLB only |
Maetzler et al. (2009), who recruited from movement clinics, found that a combined cohort of patients with DLB and PDD with raised amyloid (PiB+) did not differ in their Parkinsonism scores compared to those without (PiB-). They concluded that amyloid does not impact on motor scores. They suggested that patients seen at movement clinics with motor-related presentations are therefore less likely to display raised amyloid, whereas patients presenting at dementia clinics with more AD-like patterns of impairment will often have raised amyloid.
The pattern of raised amyloid distribution in DLB is largely similar to that seen in AD. However, the extent of amyloid is generally lower and varies greatly between subjects (Rowe et al. 2007). Across the literature assessed, the most common finding in DLB is raised amyloid in frontoparietal cortex and anterior and posterior cingulate (Rowe et al. 2007; Gomperts et al. 2008; Maetzler et al. 2009; Kantarci et al. 2012; see Fig. 17.3) although a number of these studies also reported raised amyloid in the temporal lobes and striatum. There was inconsistency regarding the occipital lobes, with three studies reporting raised amyloid (Rowe et al. 2007; Gomperts et al. 2008; Maetzler et al. 2009) and one large study reporting no difference relative to controls (Kantarci et al. 2012).
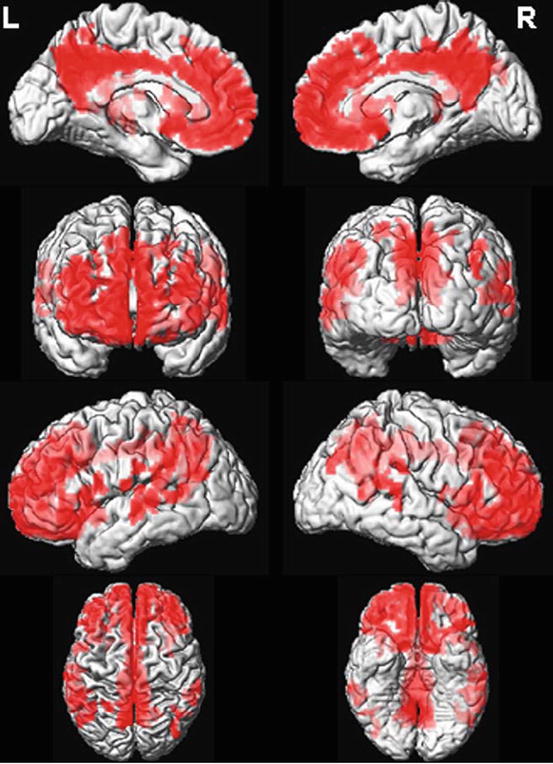
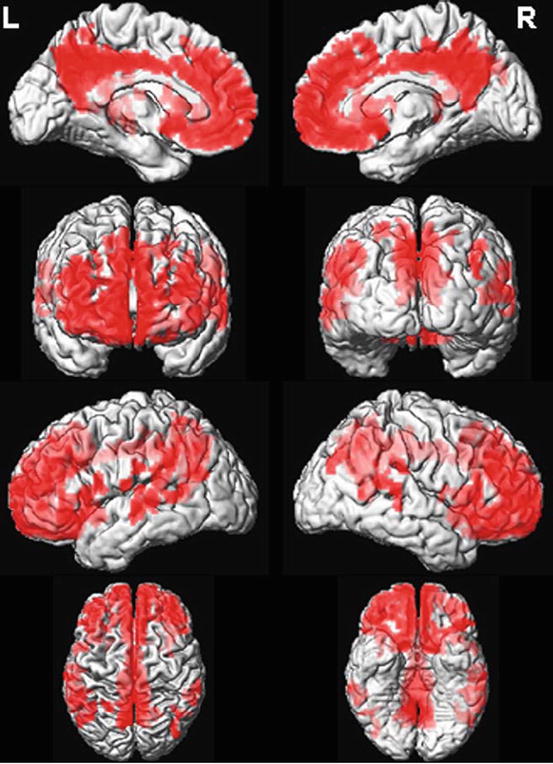
Fig. 17.3
Regional Pittsburgh compound B (PiB) retention in patients with dementia with Lewy bodies (DLB). Regional distribution of PiB retention in PiB-positive DLB patients (n = 11) was compared with PiB-negative cognitively normal (CN) subjects (n = 33). PiB retention was increased (p < 0.05; using family-wise error correction for multiple comparisons) in the frontal, parietal and superior temporal lobe association cortices (shown in red on the surface-render images). Inferior and medial temporal lobes, occipital lobes and pre- and postcentral gyri were typically spared in patients with DLB who were PiB-positive (Reproduced from Kantarci et al. (2012) with permission from Elsevier)
17.4.2 Amyloid Deposition Relative to Other Disorders and Healthy Controls
At the group level, both Rowe et al. (2007) and Kantarci et al. (2012) reported that patients with DLB showed higher PiB retention than healthy controls, but lower retention than patients with AD. Edison et al. (2008a) reported that 11 of 13 patients with DLB were PiB+, compared to just 2 of 12 patients with PDD. Gomperts et al. (2008) reported that patients with DLB had higher mean PiB retention than patients with PDD and healthy controls but that there was no significant difference between DLB and AD. Foster et al. (2010) found no difference in cortical PiB binding between healthy controls, PD (both with and without MCI), PDD and DLB, although this study lacked power due to small numbers in each group. Gomperts et al. (2012) found that patients with DLB had higher mean PiB retention than patients with PD, PD-MCI, PDD and controls, whilst the latter groups did not differ significantly from one another.
Regarding the usefulness of amyloid imaging to differentiate between DLB and healthy controls or other neurodegenerative disorders, Rowe et al. (2007) found that scans were not able to reliably distinguish DLB from AD. Gomperts et al. (2008) found that amyloid burden across their selected regions of interest (ROI) did not differentiate DLB, PDD or PD groups from one another. Kantarci et al. (2012) used a voxel-based analysis to establish if amyloid distribution differed topographically between DLB and healthy controls, but found no significant differences. However, Kantarci et al. (2012) reported that ROI-based and voxel-based analyses showed that global cortical PiB retention was useful in distinguishing patients with DLB from AD. They also used MRI and 18F-FDG PET across subjects (focusing on hippocampal volume and occipital metabolism, respectively) and found that the combination of amyloid imaging with either one of the other two modalities was a better predictor of diagnostic status than amyloid imaging alone. Incidentally, Kantarci et al. (2012) found no difference in metabolism between PiB+ and PiB- patients with DLB, despite PiB retention correlating inversely with metabolism in AD (Klunk et al. 2004). Villemagne et al. (2012) found that amyloid imaging has a low accuracy for differentiating between AD and DLB, but found that imaging striatal monoaminergic terminal integrity with the novel radioligand 18F-AV-133 and PET was effective at distinguishing DLB from AD.
17.4.3 Amyloid and Clinical Correlates
A number of studies have calculated correlation coefficients between amyloid deposition and clinical measures in patients with DLB. Rowe et al. (2007) reported that there was no significant relationship between amyloid deposition and MMSE or Clinical Dementia Rating (CDR) in DLB. Maetzler et al. (2009) analysed a combined group of PDD and DLB patients and found that PiB+ patients had lower MMSE scores than PiB− patients, although amyloid did not affect parkinsonian symptoms. Gomperts et al. (2012) found that amyloid deposition was associated with lower MMSE and worse semantic memory in DLB but not PD, PD-MCI or PDD. Conversely, this study found no association between amyloid burden and motor dysfunction in any group. Kantarci et al. (2012) found that amyloid deposition had no impact on functional impairment as measured with the CDR or Unified Parkinson’s Disease Rating Scale (UPDRS). Rowe et al. (2007) found that a higher neocortical amyloid burden was associated with a more rapid development of the core features of DLB (r = −0.75, p = 0.01) following patients’ initial presentation of cognitive impairment. This finding was supported by Villemagne et al. (2012), who found that PiB+ DLB patients had a 3.7 times shorter time from onset of symptoms to clinical diagnosis compared to PiB− patients.
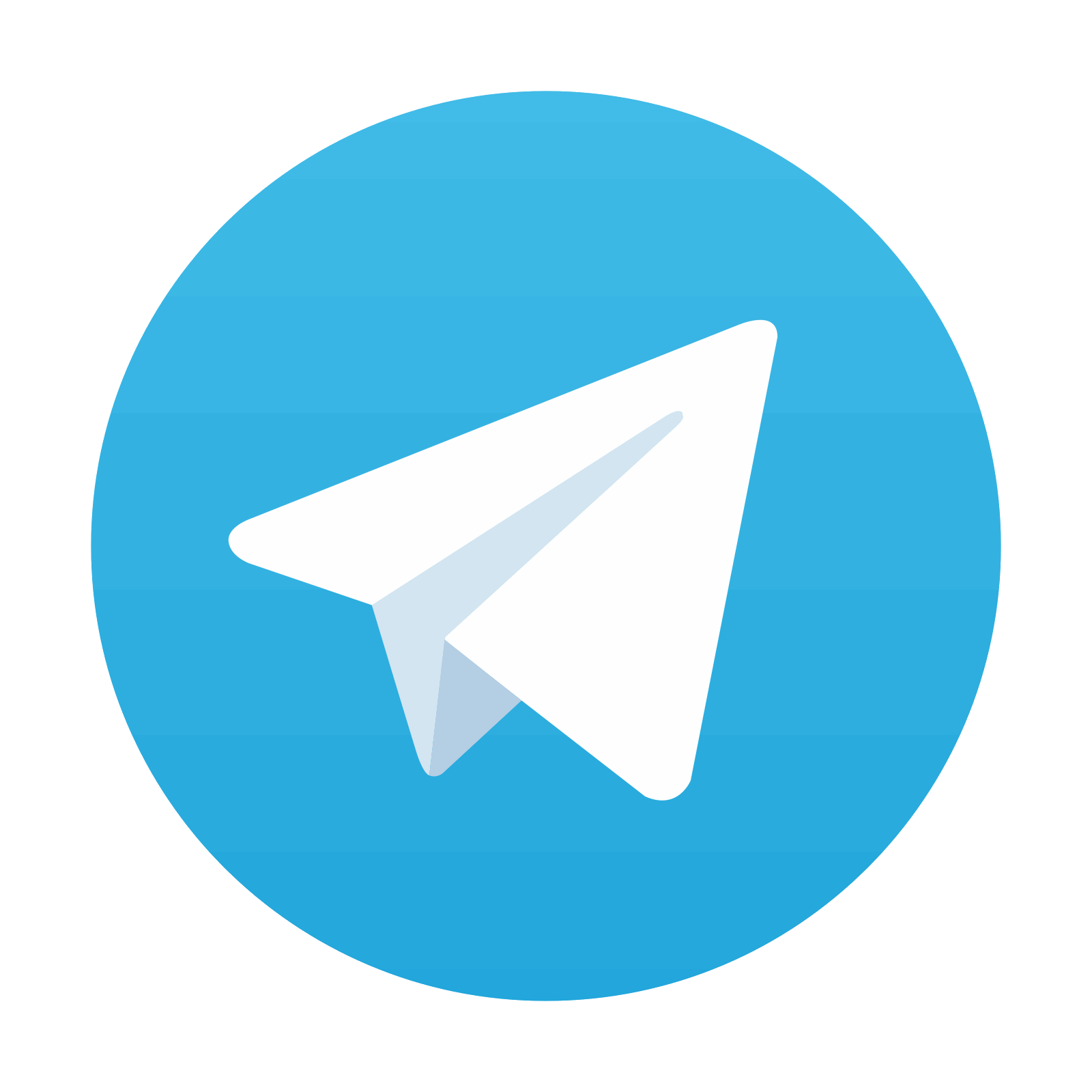
Stay updated, free articles. Join our Telegram channel

Full access? Get Clinical Tree
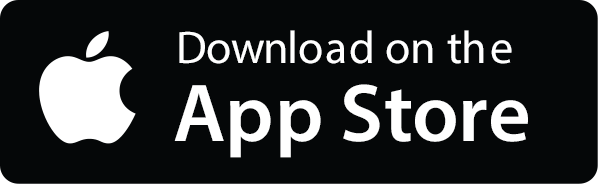
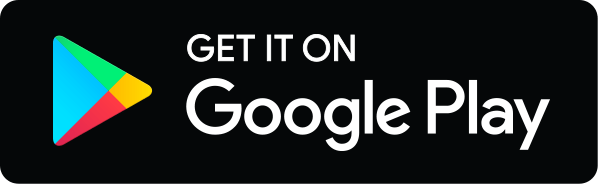