Spinal Cord
Benjamin T. Haverkamp
Peter Winningham
Winnie C. Chu
Lisa H. Hutchison
Paul G. Thacker
INTRODUCTION
A variety of disorders affect the pediatric spine. Many present with serious acute symptoms such as paresthesia, paralysis, and loss of sensation or bladder and bowel function. Accurate, prompt diagnosis is essential to prevent development of chronic morbidity and mortality.
In this chapter, we first discuss main imaging modalities and techniques used in the evaluation of spine in infants and children. Next, anatomical and embryologic concepts that are important to understanding congenital and developmental anomalies of the pediatric spine, in addition to normal variants that may simulate disease, are reviewed. A discussion of common infectious disorders, neoplasms, spinal cord trauma, vascular anomalies, and inflammatory processes follows. Emphasis is placed on clinical and imaging findings that are useful in differential diagnosis. Finally, the section on each disorder concludes with a brief discussion of treatment and prognosis.
IMAGING TECHNIQUES
Radiography
Radiographs are insufficient for evaluating the spinal cord and surrounding cerebrospinal fluid. However, radiography is the first imaging modality used to evaluate the spinal column, particularly in pediatric patients with persistent back pain or in posttraumatic patients. Fractures seen on radiography may be better detailed with computed tomography (CT)1 and magnetic resonance imaging MRI; the latter is useful for evaluating concomitant underlying spinal cord injury.1 Osteolytic changes and periosteal reaction can be seen with infection and tumors, which need further cross-sectional imaging to assess for mass effect on the cord and/or invasion of the spinal canal. Widening of the spinal canal, a subtle radiographic sign of spinal cord tumors, should be searched for in children with back pain (Fig. 4.1).
Typically, cervical, thoracic, and lumbar spine radiographic series consist of a minimum of two views, a frontal and lateral projection. Specific imaging parameters vary by patient age and size. Although odontoid views are often obtained in children 0 to 8 years of age, their routine utility has been called into question even in posttraumatic patients in this age group.2 Flexion and extension lateral cervical spine radiographs are often added to evaluate for ligamentous instability in trauma patients. Oblique views of the lumbar spine may be added to identify spondylolysis and, rarely, neuroforaminal expansion.
Ultrasound
Ultrasonography (US) is a well-established and readily available noninvasive technique for newborn and infant spinal cord evaluation.3,4,5 Because of the incompletely ossified spinal arches and predominately cartilaginous components, infants have a superb acoustic window allowing sonography to characterize nearly all congenital spinal anomalies.3 Indeed, the diagnostic value of US in newborns is nearly equal to that of MRI.3,4 However, as the infant ages and progressive ossification of the posterior elements occurs, the utility of spinal US becomes more limited.5
Sonography of the spine is performed with the infant prone using a high-frequency 7- to 12-MHz transducer.
Images are obtained in transverse and longitudinal planes. Because of ossification of the posterior elements, paramedian scans may provide the only option for sufficient visualization of the spinal cord beyond 6 months of age.5 To accurately assess the position of the conus medullaris, vertebral level can be determined by counting inferiorly starting at the twelfth rib and confirmed by counting superiorly from the lumbosacral junction. Indeterminate cases may be further assessed by performing a radiograph after placing a marker on the skin to denote the tip of the conus medullaris. Extended field-of-view (panoramic) US can be used to evaluate the entire lumbosacral spinal canal in a single longitudinal image with up to a 60-cm field of view.6 In addition, cine images are able to show normal mobility of the cauda equina nerve roots. Color or power Doppler sonography may be added to assess overlying soft tissue or spinal canal masses.3,4 Lastly, although little literature currently exists to support the clinical value, 3-dimensional (3D) US may be used to eloquently assess the spinal canal.6
Images are obtained in transverse and longitudinal planes. Because of ossification of the posterior elements, paramedian scans may provide the only option for sufficient visualization of the spinal cord beyond 6 months of age.5 To accurately assess the position of the conus medullaris, vertebral level can be determined by counting inferiorly starting at the twelfth rib and confirmed by counting superiorly from the lumbosacral junction. Indeterminate cases may be further assessed by performing a radiograph after placing a marker on the skin to denote the tip of the conus medullaris. Extended field-of-view (panoramic) US can be used to evaluate the entire lumbosacral spinal canal in a single longitudinal image with up to a 60-cm field of view.6 In addition, cine images are able to show normal mobility of the cauda equina nerve roots. Color or power Doppler sonography may be added to assess overlying soft tissue or spinal canal masses.3,4 Lastly, although little literature currently exists to support the clinical value, 3-dimensional (3D) US may be used to eloquently assess the spinal canal.6
Computed Tomography
The value of computed tomography (CT) is its ability to assess osseous and paraspinal soft tissue structures. Specifically, it is able to rapidly evaluate acute conditions such as trauma and infection. Fracture patterns can be described with high sensitivity and specificity compared to other imaging modalities. CT is only able to delineate the spinal canal after administration of intrathecal contrast.
CT parameters, particularly mAs, should be adjusted based on patient weight to keep the radiation dose as low as possible. Typical multidetector spine CT technique includes thin collimation and high-resolution bony algorithm in addition to 3-mm standard axial CT images. Reformatted sagittal and coronal CT images are routinely performed as they are particularly advantageous in the posttraumatic patient (Fig. 4.2). Intravenous and intrathecal contrast may rarely be added for specific indications such as assessment of cord pathology in children who cannot undergo MR imaging because of medical devices (such as pacemakers) or orthopedic hardware with artifact obscuring adequate visualization of the area of concern.
Magnetic Resonance Imaging
MR imaging is currently the modality of choice for evaluation of spinal canal contents. It provides superior soft tissue contrast resolution relative to other techniques without exposing the pediatric patient to the harmful effects of ionizing radiation. Furthermore, physiologic information can be garnered by the assessment of cerebrospinal fluid flow characteristics using phase contrast cine imaging and by the evaluation of bone marrow edema patterns on standard T2 and short T1 inversion recovery (STIR) sequences (Fig. 4.2C).
Typical indications for pediatric spinal MRI include neurologic symptoms such as weakness, paresthesia, or loss of bowel or bladder function that may suggest spinal cord mass; posttraumatic spinal cord injury; spinal dysraphism; atypical scoliosis; and leptomeningeal metastatic disease. A complete
discussion of spinal MR imaging protocols is beyond the scope of this chapter. However, a brief discussion of technical factors and common imaging sequences follows.
discussion of spinal MR imaging protocols is beyond the scope of this chapter. However, a brief discussion of technical factors and common imaging sequences follows.
The small spinal cord volume and relatively increased respiratory and cardiac motion in the pediatric population can make high-quality imaging of the spinal cord challenging. To achieve high-quality MR imaging, smaller fields of view with a larger imaging matrix, thin sections, and no or small interslice gaps are utilized. Because these techniques inherently decrease the signal-to-noise ratio (SNR), an increased number of excitations and longer imaging times are needed. Imaging on a 3 Tesla (T) MRI scanner can increase the SNR to improve image quality or shorten imaging times.7 A multichannel surface coil should be utilized whenever possible. Only those coils aligned with the region of interest should be employed, with coils outside the imaging field of view turned off as they contribute to image noise.
Conventional spinal MRI protocols consist of sagittal and axial T1- and T2-weighted sequences without fat saturation. However, many institutions also include sagittal, fat-saturated STIR images in order to make edema more conspicuous. For pediatric patients with severe scoliosis, coronal and/or heavily T2-weighted isotropic images with reconstructions in various planes are invaluable. Demyelinating diseases and neoplasms
require T1 postgadolinium sequences in at least two planes, typically axial and sagittal.
require T1 postgadolinium sequences in at least two planes, typically axial and sagittal.
Newer sequences have recently been reported as advantageous for the evaluation of certain pathologic processes.8 Specifically, diffusion-weighted MR imaging is able to distinguish epidermoids from arachnoid cysts.9 Authors demonstrated restricted diffusion in epidermoids compared to arachnoid cysts, which have free diffusivity. More recently, a variant of 3D fast spin echo (FSE) sequences has been introduced. Depending on manufacturer, the acronym for these sequences varies and includes SPACE, FSE-XETA, and VISTA.8 These imaging sequences are characterized by flip angle modulation during the FSE readout resulting in highresolution 3D volumetric images, which can be reformatted into multiple imaging planes. Given these capabilities, the utility of these sequences has been described in the evaluation of nerve root avulsions, posttraumatic pseudomeningoceles, scoliosis, and nerve root compression by herniated disk material.8 Diffusion tensor imaging (DTI) is also a cutting-edge technique that has recently been proposed in the spinal cord. Nerve root avulsion may be characterized by the demonstration of discontinuity between the spinal cord fibers and adjacent nerve roots.8 DTI has also been utilized in the evaluation of fiber displacement by intramedullary spinal tumors.10 Nevertheless, DTI’s usage in pediatric spinal cord imaging remains largely experimental, and further study is needed before its clinical value can be fully elucidated. Finally, phase contrast images may be added to evaluate cerebrospinal flow characteristics.11 This sequence is particularly useful to assess motion of the cerebellar tonsils in Chiari I malformations, which may obstruct CSF flow and be associated with progressively enlarging spinal cord syringomyelia.
On T2-weighted MR images, the central canal is occasionally seen as a thin tubular area of hyperintensity.12 The conus medullaris terminates at or above the L2-L3 disk space, and the thecal sac generally terminates at S2. The filum terminale extends from the conus medullaris as a hypointense cord on T1- and T2-weighted MR imaging, measuring <2 mm.
Other Imaging Modalities
The utility of imaging modalities other than those listed above is limited in the pediatric population. Myelography has been largely supplanted by MRI in children except when MRI is contraindicated. In such cases, CT myelography may be used to assess the spinal cord. Likewise, conventional angiography for diagnostic purposes has been largely replaced by MR and CT angiography for the evaluation of spinal and paraspinal vascular anomalies. However, when MR and CT angiography are inconclusive or if therapeutic intervention is planned, conventional angiography is needed. Currently, nuclear and molecular imaging has a very limited role in the evaluation of the spinal cord. However, with the increased utilization of positron emission tomography with concurrent CT coregistration, CT/PET may take on an increased role in spinal cord disorders, such as evaluation of tumors and treatment response assessment.13
NORMAL ANATOMY AND VARIANTS
Spinal Cord Embryology
Knowledge of normal embryology is helpful to understand how congenital spinal lesions develop and their imaging findings. The spinal cord originates from the neural plate, which forms the neural fold and, eventually, the neural tube. Just ventral to the developing neural tube is the notochord, which ultimately forms the vertebral bodies and intervertebral disks. Abnormalities in neural tube development result in open defects occurring in the early embryologic stages of gastrulation (weeks 2 to 3), primary neurulation (weeks 3 to 4), and secondary neurulation (weeks 5 to 6).14 If mesenchyme migrates into the developing neural tube, a lipomyelocele or lipomyelocystocele can occur.15 Diastematomyelia develops early during embryogenesis, likely from adhesion between ectoderm and endoderm with splitting of the early notochord.5
Synchondrosis Closure
Knowledge of synchondrosis closure is important when evaluating CT studies in the setting of trauma. It can sometimes be challenging to distinguish between a normal developmental process from an acute fracture. Synchondrosis closure in the spine occurs from cranial to caudal. The anterior and posterior atlas arches close around 4 years of age, range 2 to 13 years. The C2 base of dens synchondrosis usually fuses around 9 years of age.16
Normal Anatomy
Ultrasound is the screening imaging modality of choice in infants because of its accuracy, relatively low cost compared to MRI, and lack of need for sedation or radiation exposure. In infants, suboccipital US can be used to show the hypoechoic pons, medulla oblongata, and spinal cord.5 The center of the spinal cord contains two parallel echogenic lines, due to overgrown glial debris, surrounding a hypoechoic center.5 The conus medullaris, the most caudal portion of the cord, is located at or above the L2-L3 disk level (Fig. 4.3). The filum terminale is a midline echogenic structure that extends from the tip of the conus to the distal thecal sac. It is surrounded by echogenic nerve roots. The spinal cord varies in diameter depending on level, being largest in diameter in the cervical and lumbar regions because of the increased density of efferent and afferent fibers supplying the upper and lower extremities (Fig. 4.4).
Normal Variants
Besides the normal variants previously mentioned, a prominent filum and central echo complex, one must be aware of other variants in order to avoid incorrectly suggesting disease. Common variants are discussed below including filar cysts,
prominent filum terminalis, ventricular terminalis, cauda equina pseudomasses, pseudosinus tracts, and borderline low conus position.
prominent filum terminalis, ventricular terminalis, cauda equina pseudomasses, pseudosinus tracts, and borderline low conus position.
Filar cysts, a common finding on neonatal sonography, are oblong midline cysts located within the filum terminale just below the conus medullaris. They should not be confused with a ventriculus terminalis (discussed below), a different normal variant that is located within the conus medullaris. The frequency in which filar cysts are seen on US is inversely proportional to age up until around 6 months.17 Seen in isolation, filar cysts are associated with normal developmental milestones. Although common on infant US, no filar cyst has ever been described at autopsy, begging the question as to whether they are merely pseudocysts or related to normal anatomic scaffolding of the neonatal spinal canal (Fig. 4.5).17
Prominent filum terminalis is seen as an echogenic linear midline structure extending from the tip of the conus to the distal thecal sac among the cauda equina nerve roots.
Ventriculus terminalis is a cystic structure located within the distal spinal cord just above the conus medullaris. It is thought to be a remnant of the embryologic canalization and retrogressive differentiation processes that form the tip of the conus and coccyx.18
Cauda equina pseudomasses occur when infants are scanned while lying on their side and are caused by nerve roots clumping together in the dependent portion of the spinal canal. This can be easily distinguished from a true mass by placing the infant prone and rescanning the region.4
Pseudosinus tracts are seen in many infants as a fibrous cord of tissue extending from the coccyx to a skin dimple in the gluteal crease (Fig. 4.6). If the dimple is 2.5 cm above the gluteal crease; if it has skin stigmata such as pigmentation, hair, or a skin tag; or if fluid is draining via this tract, then a true sinus tract requiring surgery is likely present. Without these clinical markers, a pseudosinus tract is most likely, especially if the dimple is within the gluteal crease.
Borderline low conus position between the L2-L3 disk space and the mid L3 vertebral body level is common in infants and has been shown to correlate with normal developmental milestones on clinical follow-up evaluation making
additional workup and MR imaging unwarranted (Fig. 4.7).19 However, if clinical concern remains, follow-up sonography may be easily performed up to 6 months of age.
additional workup and MR imaging unwarranted (Fig. 4.7).19 However, if clinical concern remains, follow-up sonography may be easily performed up to 6 months of age.
SPECTRUM OF PEDIATRIC SPINAL CORD DISORDERS
Congenital and Developmental Anomalies
Congenital and developmental anomalies of the spine and spinal cord are collectively referred to as spinal dysraphisms, which is a broad term that covers a spectrum of disorders with incomplete or absent fusion of midline mesenchymal and neural structures.
Spinal dysraphisms are approached by first categorizing lesions into open and closed defects.14 Open spinal dysraphisms (OSDs) have defects in the overlying skin allowing neural tissue with or without its associated subarachnoid space to be exposed to air. Open entities include myelomeningoceles and myeloceles. Hemimyelomeningocele and hemimyelocele are rare entities in the same group, in which the myelo(meningo)cele affects one of two hemicords in the setting of diastematomyelia.20,21 Closed spinal dysraphisms (CSDs) have components that extend through spinal defects with intact skin overlying neural tissue. Morphologically, CSDs are subcategorized based on the presence or absence of a raised lumbar subcutaneous mass. An approach to classifying spinal dysraphisms is included in Fig. 4.8.
Myelomeningocele and Myelocele
Both myelomeningoceles and myeloceles are classified as OSDs having a placode exposed to air. Both are due to defective closure of the primary neural tube15,22 with persistence of a segment of nonneurulated placode while cutaneous ectoderm does not detach from the neural ectoderm and remains in a lateral position.
Myelomeningoceles are far more common OSDs than myeloceles. The former account for more than 98.8% of OSDs.23 The previously quoted incidence is 0.6 per 1,000 live births, but the incidence is currently decreasing because of prenatal screening and preventive maternal dietary folic acid supplementation. Myeloceles only account for 1.2% of OSDs and are most common at the lumbar or lumbosacral levels.
On imaging, the main differentiating feature between the two conditions is the relative position of the neural placode with reference to the skin surface.14 In myelomeningoceles, the neural placode protrudes above the skin surface because of expansion and protrusion of the underlying subarachnoid space (Fig. 4.9). In myeloceles, the ventral subarachnoid space is not protruding; hence, the placode is flush with the skin surface (Fig. 4.10). Although the diagnosis is usually obvious clinically, MRI is useful for surgical planning because it is able to characterize the lesion and assess for associated findings of a Chiari II malformation, including hydrocephalus and hydromyelia.
Chiari II Malformation
There is always consistent association between OSD and the Arnold-Chiari II malformation. The latter is a complex
congenital anomaly of the hindbrain characterized by descent of the cerebellar vermis through the foramen magnum, elongation and kinking of the medulla, caudal displacement of the cervical spinal cord and medulla, and obliteration of the cisterna magna.24 The pathogenesis is explained by a CSF leak from the OSD, resulting in a lack of appropriate distention of the embryonic ventricular system. The chronic CSF hypotension within the developing neural tube leads to formation of a hypoplastic posterior fossa and subsequent herniation of the cerebellum and brainstem through the foramen magnum.25 A recent fetal MRI study has suggested that caudal traction by cord tethering also plays a role in a subset of patients with Chiari malformations.26
congenital anomaly of the hindbrain characterized by descent of the cerebellar vermis through the foramen magnum, elongation and kinking of the medulla, caudal displacement of the cervical spinal cord and medulla, and obliteration of the cisterna magna.24 The pathogenesis is explained by a CSF leak from the OSD, resulting in a lack of appropriate distention of the embryonic ventricular system. The chronic CSF hypotension within the developing neural tube leads to formation of a hypoplastic posterior fossa and subsequent herniation of the cerebellum and brainstem through the foramen magnum.25 A recent fetal MRI study has suggested that caudal traction by cord tethering also plays a role in a subset of patients with Chiari malformations.26
Conventionally, pediatric patients with myelomeningoceles and myeloceles undergo corrective surgery in the postnatal period aimed at covering the exposed placode to prevent ulceration and infection, as well as treating hydrocephalus with a ventricular shunt. Recently, in utero surgery has been performed on selected patients aimed at preventing secondary damage of the spinal cord by a combination of factors such as amniotic fluid exposure, direct trauma, and hydrodynamic pressure27 based on the “ two-hit” hypothesis.28 The goals of fetal surgery are to reverse hindbrain herniation (Chiari II malformation), reduce the need for ventriculoperitoneal shunting due to hydrocephalus, and prevent later neurologic deficits due to cord tethering.29
Lipomyelocele and Lipomyelomeningocele
Both lipomyeloceles and lipomyelomeningoceles are CSDs that present with a subcutaneous mass at the lumbosacral region beneath the intact skin. Lipomyeloceles are about twice as common as lipomyelomeningoceles.23 The lipomas are associated with a dural defect. These anomalies account for ˜76% of all spinal lipomas and 16.4% of all CSDs. Lipomyeloceles and lipomyelomeningoceles have been attributed to a primary neurulation defect in which there is focal premature disjunction of cutaneous ectoderm from neuroectoderm.30 The mesenchymal cells enter the neural tube to form fat (lipoma) and prevent further neurulation. The lipoma extends posteriorly through the spinal defect and merges with the subcutaneous fat.
MRI is the imaging modality of choice. The main MR imaging feature that differentiates lipomyeloceles from lipomyelomeningoceles is the position of the placode-lipoma interface relative to the spinal canal. In lipomyeloceles, the placode-lipoma interface is within or at the edge of the spinal canal. The subcutaneous fat extends into the spinal canal and attaches to the spinal cord (Fig. 4.11). In lipomyelomeningoceles, the placode-lipoma interface is outside the spinal canal because of protrusion of the subarachnoid space through the osseous defect. A subcutaneous fatty mass extends through a lumbosacral defect attaching to the open neural placode (Figs. 4.12 and 4.13). The cord is often rotated and asymmetrically tethered by the lipoma, and hydromyelia may be present.31 There is also unequal development of the nerve roots that may cause cord tethering.
Early surgical treatment is advocated in these pediatric patients to prevent neurologic deficits.32 The surgical goals include total or subtotal removal of the adipose mass, identification of the lumbosacral fascial defect to release tethering, possible release of the filum terminale, preservation
of neural elements, and prevention of retethering of the spinal cord.33
of neural elements, and prevention of retethering of the spinal cord.33
Meningocele
Posterior meningocele, which is classified under CSD, is uncommon and of unknown etiology. It has been hypothesized that it results from protrusion of meninges through a posterior spinal defect because of subarachnoid space CSF pulsations. Most posterior meningoceles are lumbar or sacral in location, but thoracic and cervical lesions are rarely reported. On imaging, the classic posterior meningocele is characterized by a CSF-filled sac herniating through a posterior spinal defect (Fig. 4.14). By definition, no neural tissue is contained within the sac, although it may be tethered to the neck of the meningocele. Additionally, nerve roots or even the filum terminale may subtly course through the meningocele.14 Because posterior meningoceles are frequently associated with other occult spinal lesions, MRI of the entire spinal column should be performed for a complete assessment.34
Anterior meningoceles and intrasacral meningoceles are occult spinal dysraphisms without a subcutaneous mass. Anterior meningoceles are presacral in location and found consistently in pediatric patients with caudal agenesis. They may be discovered only in older children or adults complaining of low back pain, urinary incontinence, or constipation.35 Intrasacral meningocele is an intrasacral extradural cystic lesion caused by arachnoid diverticulum through a small dural defect at the caudal end of the thecal sac. As distinguished from the more common perineural (Tarlov) cysts, intrasacral meningoceles are not associated with any exiting nerve root and, thus, spare the sacral foramina. They may cause sacral pain or sacral nerve root dysfunction by local compression.
Posterior meningoceles generally require surgical repair; anterior and intrasacral meningoceles are surgically treated only when symptomatic. The goal of surgery is to identify the point of herniation through the dura mater and ligate the cyst. After ligation, most affected children have resolution of symptoms.1
Terminal Myelocystocele
Terminal myelocystocele (TMC) is a rare form of CSD. The key feature is extrusion of the distal spinal cord into the extraspinal space with its terminal part expanding into a cystic cavity forming the TMC. The functional conus is located in the more rostral part of the TMC. There are a few associated features of TMC that vary from case to case. The intraspinal subarachnoid space may expand and extend into the extraspinal fat space, forming a meningocele surrounding the TMC. Some degree of hydromyelia may occur, usually confined to the lumbosacral region. Rarely, hydromyelia can be enormous and communicate with the TMC. The amount of subcutaneous fat covering the TMC varies, ranging from slightly thicker than normal skin to exuberant lipoma.36
The pathogenesis of TMC is not fully understood, but most likely related to an anomaly of secondary neurulation and retrogressive differentiation.36 It is suggested that TMC may be caused by a time-specific paralysis of apoptosis just before the dehiscence of the cystic distal cord from the future skin, thereby preserving the embryonic components within the cyst.
The key MR imaging feature is that of a “cyst within a cyst” (Fig. 4.15). The inner cyst represents the trumpet-shaped, CSF-filled myelocystocele, which is sometimes in continuity with a hydromyelia cavity (syringocele) within the intraspinal portion of the cord. The outer cyst wall represents the extraspinal extension of the expanded dura, which communicates with the spinal subarachnoid space. The cord is always tethered. Sagittal isotropic 3D reconstructed MR images are particularly able to demonstrate the entire spinal cord in a single plane when scoliosis is present, which can be useful to depict the continuity between the myelocystocele and the spinal cord syringocele.37
Early surgical repair is recommended in TMC as it may undergo rapid expansion in infancy, thus causing stretch injury to the conus, which is situated at the proximal part of the cyst. Goals of surgery are to reduce the size of the mass by resection of the nonfunctional caudal cyst wall, untether the placode, and finally, close the syringocele cavity by pia-arachnoid suture.38,39 The caudal part of the central canal is left open to the subarachnoid space by terminal ventriculostomy to avoid hydrosyringomyelia formation.40
Diastematomyelia
Diastematomyelia, or a split cord, is due to failure of midline notochordal integration resulting in two hemicords.21 Further subtypes of this malformation depend on variable primitive notochordal development.20 MR imaging features can differentiate subtypes by determining the number of dural sacs containing hemicords and if there is an osteocartilaginous spur or fibrous septum.
Axial MR images are best able to show splitting of the spinal cord as well as associated hydromyelia, low conus position, and intradural lipomas.41 In type 1 diastematomyelia,
the spinal canal is split into two halves by either an osseous or osteocartilaginous septum (spur), and each dural sac contains a hemicord. The hemicords usually fuse below the spur to form a single cord. Hydromyelia is common in the hemicords and the normal cord above and/or below the splitting. Most cases are also associated with vertebral segmentation anomalies. Osseous anomalies, including the bony septum, may be further evaluated by CT. In type 2 diastematomyelia, a single dural sac contains both hemicords with no osteocartilaginous spur (Fig. 4.16). However, a fibrous septum can occur seen as a thin hypointense band on high-resolution axial and coronal MR images. Again, associated vertebral anomalies are common but generally milder than in type 1 diastematomyelia.
the spinal canal is split into two halves by either an osseous or osteocartilaginous septum (spur), and each dural sac contains a hemicord. The hemicords usually fuse below the spur to form a single cord. Hydromyelia is common in the hemicords and the normal cord above and/or below the splitting. Most cases are also associated with vertebral segmentation anomalies. Osseous anomalies, including the bony septum, may be further evaluated by CT. In type 2 diastematomyelia, a single dural sac contains both hemicords with no osteocartilaginous spur (Fig. 4.16). However, a fibrous septum can occur seen as a thin hypointense band on high-resolution axial and coronal MR images. Again, associated vertebral anomalies are common but generally milder than in type 1 diastematomyelia.
Prophylactic cord untethering and repair of diastematomyelia are advocated for better clinical outcome because residual deficits persist once they are present preoperatively. Associated hydromyelia is always unchanged after surgery, indicating it does not contribute to the neurologic syndrome.42
Tethered Cord Syndrome and Filar Lipoma
Tethered cord syndrome is not a single entity but a clinical syndrome that involves progressive neurologic deterioration, in particular, both motor and sensory dysfunction of the lower limbs. Other associated neurologic deficits include spastic gait, muscle atrophy, abnormal reflexes, urinary incontinence, and bony deformities such as scoliosis. The pathogenesis is related to traction of the spinal cord. The cord traction can be directly related to lesions within the spinal canal (in the form of CSD) or secondary to scarring or formation of dermoids after surgical repair of myelomeningocele. When the cord is tethered/stretched, the vascular supply may be compromised, which leads to functional deterioration of nerve cells.43
MR imaging shows the classic low conus medullaris position (below the L2-L3 disk space) and variable other associated anomalies of the spine and spinal cord; the most common of which include a tight filum terminale, diastematomyelia,
intradural lipomas, and caudal agenesis (Fig. 4.17).44 The conus may be radiologically “normal” in position in cases of tight filum terminale with or without filar lipomas.45 The presence of hydromyelia heralds deterioration of neurologic function.
intradural lipomas, and caudal agenesis (Fig. 4.17).44 The conus may be radiologically “normal” in position in cases of tight filum terminale with or without filar lipomas.45 The presence of hydromyelia heralds deterioration of neurologic function.
Untethering of the cord is indicated in pediatric patients with progressive or new-onset neurologic symptoms, and the goal of surgery is to improve blood flow within the spinal cord. Surgical success rates vary depending on the nature and severity of anomalies. In general, earlier operative intervention is associated with improved outcome such as pain relief and stabilization of neurologic function. Cord untethering may also halt scoliosis progression.46
Dermal Sinus
The dermal sinus, a common CSD accounting for ˜25% of cases, is an epithelium-lined sinus tract or fistula extending from the skin surface inward where it may connect with the CNS and its meningeal layers.23 The incidence of dermal sinus is cited as 1 in 2,500 live births, but the exact incidence is unknown.47 It is most frequently found in the lumbosacral region as a midline dimple or pinpoint ostium above the gluteal crease in the midline, or less commonly, lateral of midline.48 Associated cutaneous stigmata such as hairy nevi, hyperpigmented patches, or hemangiomas are common. Dermal sinus is believed to be due to incomplete disjunction of cutaneous ectoderm from the neural tube. This leads to the formation of a sinus tract lined by epithelium with dermal elements (Fig. 4.18), all of which may communicate with the CNS.
On MRI, the key feature of a dermal sinus is a hypointense linear focus coursing obliquely and downward in the subcutaneous fat on sagittal images (Fig. 4.19). Associated
intraspinal anomalies are common. Dermal sinuses can be connected to a hypertrophic or fibromatous and/or fatty filum terminale49 and may have a low conus medullaris and/or an intraspinal lipoma.50,51 Dermoids at the level of the cauda equina may also be present (inclusion tumor) and are likely due to encystment of part of the dermal sinus tract.52
intraspinal anomalies are common. Dermal sinuses can be connected to a hypertrophic or fibromatous and/or fatty filum terminale49 and may have a low conus medullaris and/or an intraspinal lipoma.50,51 Dermoids at the level of the cauda equina may also be present (inclusion tumor) and are likely due to encystment of part of the dermal sinus tract.52
Neonatal lumbar US screening is useful to differentiate a dermal sinus from a coccygeal dimple.5 US may even be superior to MRI at depicting the relationship of the sinus tract to the conus because of its superior resolution in infants and lack of respiratory artifacts that occur on newborn MRI. However, the utility of US declines in pediatric patients older than 4 months when ossification of the posterior elements of the spine limits the acoustic window.53
Untreated dural sinus tracts with subarachnoid connections can serve as a pathway for spread of bacterial infection to the CNS resulting in meningitis and, less frequently, abscess formation. Thus, early surgical sinus tract resection and correction of associated abnormalities are needed.54
Intradural Lipoma
Intraspinal lipomas are rare congenital and histologically benign neoplasms that account for <1% of all spinal cord tumors.55 Of all spinal lipomas, 24% are intradural.23 Intradural lipomas are most common in the lumbosacral region at the lower thecal sac and present clinically with tethered cord syndrome.56 They may be solitary or multifocal and may grow to expand the spinal canal. In the cervicothoracic region, they may produce insidious signs of spinal cord compression.
Intradural lipomas are thought to be an anomaly of primary neurulation involving premature disjunction of the neural tube from surrounding ectoderm. The neural plate is left open posteriorly so that mesenchymal cells gain access to the ependymal lining, which induces them to differentiate into fatty tissue.15,57 The key imaging feature for diagnosis of intradural lipoma is that the mass follows fat signal on all MR sequences including fat suppression sequences (Fig. 4.20). Similarly, it has the same attenuation value as fat on CT and is echogenic on US.
Surgical resection is required in all symptomatic pediatric patients because intradural lipomas may cause mass effect on the conus and nerve roots. The surgical goals are to release tethered neural elements and reduce lesion size. The decision to operate in asymptomatic pediatric patients with intradural lipomas is controversial; however, early decompressive surgery is suggested for patients with large intradural lipomas to prevent clinical worsening if the lesion increases in size as the child grows.58
Hydrosyringomyelia
Hydrosyringomyelia is a chronic degenerative process of the spinal cord in which the central canal gradually expands. In children, it is most often developmental, is seen in association with Chiari malformations, and can present at any age.59 However, it may be idiopathic or acquired because of spinal cord tumors, hemorrhage, infarcts, or trauma. The pathophysiology of hydrosyringomyelia is thought to be related to abnormal cerebrospinal fluid (CSF) flow dynamics. One theory suggests that it may be due to increased pressure in the subarachnoid space, whereas a more recent theory suggests that it is an extracellular accumulation of CSF within the distended spinal cord.60,61 The clinical presentation of hydrosyringomyelia may include skeletal lesions such as scoliosis or pes cavus and sensory deficits.62 Other presenting symptoms
may include lower cranial nerve palsy, motor weakness, and muscle atrophy.62
may include lower cranial nerve palsy, motor weakness, and muscle atrophy.62
Although hydrosyringomyelia may be seen clearly with sonography in infants, MRI with contrast is usually needed for complete assessment of the brain and spinal cord, especially if surgery is planned. The key finding on MRI is T2-hyperintense central cord distention over one or many vertebral segments (Fig. 4.21). When a syrinx is seen, a careful search must be made for an underlying cause, such as a Chiari I malformation, cord tumor, or other cord lesion. Although no contrast enhancement is seen in simple cases of hydrosyringomyelia, contrast is often given during the initial assessment to search for small occult tumors or vascular malformations. MRI allows performance of reconstructed isotropic 3D heavily
T2-weighted MR images of the entire spinal cord, improving visualization of lesions in the setting of scoliosis and allowing physiologic assessment of CSF flow in Chiari I malformations using phase contrast sequences.60,63,64
T2-weighted MR images of the entire spinal cord, improving visualization of lesions in the setting of scoliosis and allowing physiologic assessment of CSF flow in Chiari I malformations using phase contrast sequences.60,63,64
Treatment of hydrosyringomyelia depends on its cause. Early posterior fossa decompression with or without duraplasty is performed in Chiari I malformations with associated syrinx, leading to symptomatic improvement in 70% to 85% of affected patients.59,65,66 Spinal cord tumors and vascular lesions may be debulked or resected. Some vascular lesions may undergo catheter embolization, which is discussed in greater detail later in this chapter.
Neurenteric Cysts
Neurenteric cysts are lined by a mucin-secreting epithelium resembling that of the alimentary tract (Fig. 4.22). They can be found anywhere along the spinal canal and are typically located in the cervicothoracic region anterior to the cord. Fewer lesions are found at the lumbar and sacral levels.67 The pathogenesis is related to endodermal differentiation of primitive streak remnants that remain trapped within a split notochord.
Neurenteric cysts can be viewed as gut duplication within the spinal canal. They include three histologic types. Type 1 is the simplest and most common form, which is thin walled with a layer of stratified or pseudostratified cuboidal or columnar epithelium. The more complex forms are less common, characterized by additional mesodermal elements such as smooth muscle and fat (Type 2) and sometimes ependymal or glial tissue (Type 3). Neurenteric cysts can be isolated or associated with spinal defects or diastematomyelia.68 The most common pediatric presentation is cord compression,50 usually in the first decade of life,69 although meningism (symptoms mimicking meningitis) is the presenting feature in newborns and infants.70
Key imaging features of neurenteric cysts (Fig. 4.23) include a cyst without enhancement that is either T1 isointense or slightly T1 hyperintense on MRI because of variable proteinaceous contents.71
The treatment of neurenteric cysts is complete surgical resection.71 If total excision is not feasible because of adherence of cyst to vital structures, wide fenestration of the cyst wall is performed. The overall prognosis depends on associated anomalies, but usually long-term stability and survival are achieved.72
Infectious Disorders
Viral Myelitis
Viral myelitis is rare, but important to consider in children with acute myelopathy. Although many viruses may involve the spinal cord, such as polioviruses, enterovirus 71 (which causes hand-foot-and-mouth disease), arboviruses (e.g., West Nile virus), flaviviruses, coxsackie viruses, human immunodeficiency virus (HIV), Japanese encephalitis virus, and echovirus, isolation of a specific virus is rare.73,74,75 The pathophysiology in viral myelitis is not well understood. It may be related to primary viral infection or postinfectious demyelination immune response.76
Clinically, affected pediatric patients most often present with sensory loss, bilateral upper and/or lower extremity weakness, aseptic meningitis, rhombencephalitis, myoclonus, tremor, and bladder and bowel dysfunction.74 Acute flaccid paralysis may occur, indicating spinal cord anterior horn involvement.76
Initial workup of these children should include MRI evaluation of the brain and entire spinal cord in order to determine the extent of disease, exclude surgical causes of paralysis, and narrow the differential diagnosis.76 Spinal cord
lesions are T2 hyperintense with variable enhancement. They may be single or multiple, unilateral or bilateral, and classically involve the anterior cord (Fig. 4.24).75,76,77 Superficial leptomeningeal enhancement of the spinal cord, nerve roots— especially the ventral roots—and cauda equina may be seen.75,76 When lesions involve both sides of the cord, the appearance is the same as transverse myelitis.78 MRI of the brain is useful to look for additional viral lesions and to help exclude other disorders such as multiple sclerosis.
lesions are T2 hyperintense with variable enhancement. They may be single or multiple, unilateral or bilateral, and classically involve the anterior cord (Fig. 4.24).75,76,77 Superficial leptomeningeal enhancement of the spinal cord, nerve roots— especially the ventral roots—and cauda equina may be seen.75,76 When lesions involve both sides of the cord, the appearance is the same as transverse myelitis.78 MRI of the brain is useful to look for additional viral lesions and to help exclude other disorders such as multiple sclerosis.
![]() FIGURE 4.24 Viral myelitis in a 5-year-old girl who presented with arm weakness and pain. Axial T2-weighted MR image shows a unilateral, mostly anterior lesion (arrow) in the thoracic spinal cord. |
The treatment for viral myelitis is largely supportive. However, it may include methylprednisolone or antiviral medications in cases with PCR sequence identification of a specific virus.74 The prognosis in viral myelitis varies. In one study of children with enterovirus 71 myelitis, 57% completely recovered and 43% were left with at least a mild deficit.76,79 Children with bilateral rather than unilateral MRI lesions were more likely to have persistent morbidity such as arm or leg weakness,76 and those with cardiopulmonary failure associated with brain lesions were more likely to have neurodevelopmental delay and permanent morbidity.80
Bacterial Myelitis
Bacterial myelitis is caused by a variety of agents, including streptococcus, staphylococcus, Mycoplasma pneumoniae, Mycobacterium tuberculosis, rickettsia, and Borrelia burgdorferi (Lyme disease/neuroborreliosis).81 Although intracranial manifestations, such as meningitis, are most common, spinal myelitis and abscess occur occasionally.82,83,84 The disease source is hematologic spread of infections from other organ systems, such as skin, lungs, and the gastrointestinal tract.85 The pathogenesis of bacterial myelitis may be because of direct bacterial infection and/or a secondary immune-mediated response in which the host produces antibodies that attack the spinal cord.83,86
The clinical presentation may be gradual or acute including fever, upper and/or lower extremity weakness, paralysis, and/or paresthesias. Bladder and bowel dysfunction, ataxia, postural instability, back pain, respiratory difficulty, intractable vomiting, and fever may also occur.83,84,87 Associated septic meningitis, encephalitis, stroke, radiculopathy, and transverse myelitis are seen with Mycoplasma.86
MRI is the initial imaging modality of choice in bacterial myelitis. Imaging findings are nonspecific, including T2-hyperintense cord swelling with variable enhancement of the superficial cord and/or nerve roots (Fig. 4.25).82,85,87 A focal lesion with ring enhancement should raise concern for a bacterial etiology.85 Lesions of the thoracic cord, enhancing basilar cisterns, and epidural and/or cord abscesses may suggest a diagnosis of tuberculosis (Fig. 4.26).84 Neuroborreliosis/Lyme disease and tuberculosis may have cranial nerve enhancement as well.88 The differential diagnosis for spinal cord lesions is lengthy but can be limited by correlating with history, physical findings, and laboratory data.
Treatment depends on the cause of myelitis but typically includes antibacterial medications and methylprednisolone.85 Bacterial abscesses of the cord usually resolve with antibiotics; however, in cases with associated epidural or soft tissue abscesses, surgical drainage may be required.83,84,85 Prognosis varies depending on the severity and extent of infection. Cord atrophy and cavitation portend permanent morbidity.84
Neoplastic Disorders
Spinal tumors occur predominantly in young or middle-aged adults and are less common in children. Only 2% of all childhood tumors are spinal in origin. Often symptoms are nonspecific, most commonly manifesting as neck or back pain and clumsiness.89 More specific symptoms may include motor weakness, changes in gait, spinal curvature, and bowel and bladder dysfunction. In the setting of acute trauma, peritumoral edema may cause paresis or paralysis. This chapter presents an overview of spinal neoplasms, with subsequent detailed discussion of lesions categorized based on anatomic location: intramedullary, intradural extramedullary, and extradural.90
The imaging features of spinal neoplasms are often nonspecific with overlapping findings. However, familiarity with age at diagnosis combined with key imaging findings and associations may be helpful in narrowing the differential diagnosis.
Although plain radiographs may show osseous spinal erosions, this is a late finding, making radiography of limited utility for defining and characterizing pediatric spinal cord tumors. Unenhanced CT is helpful to image primary osseous lesions, but it is not the best imaging modality for evaluating the spinal cord. Instead, MRI is the study of choice to define and characterize spinal cord lesions.
Differentiating intramedullary (within the cord) versus extramedullary (outside of the cord) and intradural (within
the thecal sac) versus extradural (outside the thecal sac, likely osseous) lesions is important and can be accomplished with multiplanar MRI. Intramedullary lesions show cord expansion, whereas extramedullary lesions are separate from the cord in at least one of three planes. Intraoperative ultrasound may be used to determine tumor location and borders during exposure and resection. Baseline postoperative MRI is deferred for at least 12 weeks as surgical changes make early postoperative scans difficult to interpret.91
the thecal sac) versus extradural (outside the thecal sac, likely osseous) lesions is important and can be accomplished with multiplanar MRI. Intramedullary lesions show cord expansion, whereas extramedullary lesions are separate from the cord in at least one of three planes. Intraoperative ultrasound may be used to determine tumor location and borders during exposure and resection. Baseline postoperative MRI is deferred for at least 12 weeks as surgical changes make early postoperative scans difficult to interpret.91
The treatment goal with benign, noninfiltrative spinal neoplasms is complete excision of both the solid tumor and associated syrinx cavities. If any portion of the neoplasm infiltrates the cord, surgical success decreases. Adjuvant radiation therapy and chemotherapy may be used, but the prognosis with an infiltrative, malignant lesion remains dismal.92
The surgical approach begins with a laminectomy and myelotomy to access and centrally debulk the tumor. Electrophysiologic monitoring techniques can be helpful when removing the periphery of the tumor, in order to prevent damage to the healthy lateral columns of the spinal cord. The tumor and its associated syrinx cavity are resected to their interface with normal-appearing spinal cord.
In general, preoperative neurologic deficits may persist postoperatively, but most tumors can be removed without new long-term disabilities. Symptomatic recurrences can be managed by reoperation. Residual and recurrent tumor deposits often remain static for years or grow very slowly. If complete tumor excision is not possible with histologically benign pediatric intramedullary gliomas, adjuvant radiation therapy is preferentially avoided because of adverse effects on the immature spinal cord and spinal column.
Surgical management is considered with extradural tumors to establish pathologic diagnosis with biopsy, to decompress the spinal cord in the setting of progressive myelopathy, for reconstruction in cases of spinal instability, and with attempted radical resection for cure. The majority of childhood spinal epidural lesions are responsive to radiation or chemotherapy.93
TABLE 4.1 Pediatric Intramedullary Spinal Neoplasms: Key Imaging Features, Associations, Treatments, and Other Comments | |||||||||||||||||||||||||||||||||
---|---|---|---|---|---|---|---|---|---|---|---|---|---|---|---|---|---|---|---|---|---|---|---|---|---|---|---|---|---|---|---|---|---|
|
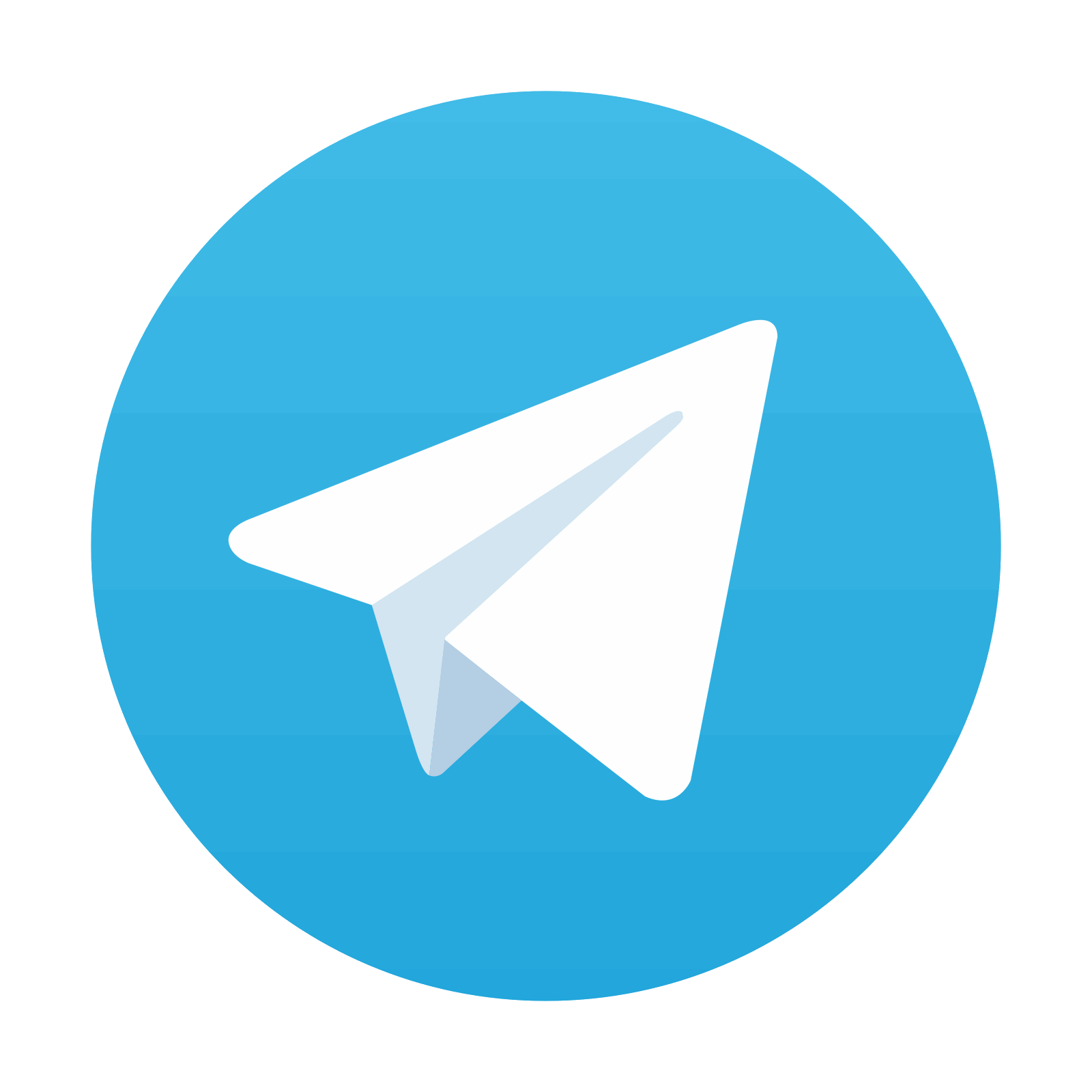
Stay updated, free articles. Join our Telegram channel

Full access? Get Clinical Tree
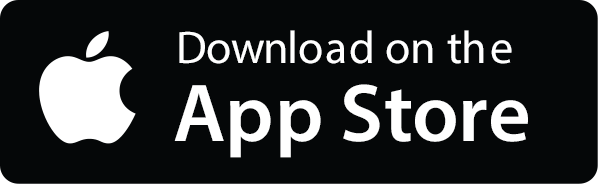
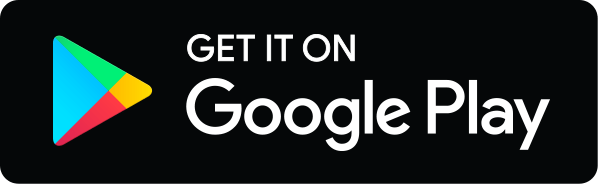